Lecture 20: Renal Physiology PDF
Document Details
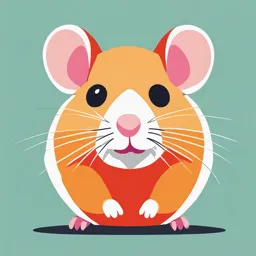
Uploaded by ExaltedCanyon98
School of Human Nutrition
Tags
Summary
This lecture provides an overview of renal physiology, focusing on the processes of reabsorption and secretion in the nephron. It also discusses renal transport saturation and countercurrent systems.
Full Transcript
About This Chapter 19.5 Reabsorption 19.6 Secretion Copyright © 2019, 2016, 2013 Pearson Education, Inc. All Rights Reserved 19.5 Reabsorption • Reabsorption may be active or passive Active: to move solute out of lumen, tubule cells must use active transport to create concentration or electro...
About This Chapter 19.5 Reabsorption 19.6 Secretion Copyright © 2019, 2016, 2013 Pearson Education, Inc. All Rights Reserved 19.5 Reabsorption • Reabsorption may be active or passive Active: to move solute out of lumen, tubule cells must use active transport to create concentration or electrochemical gradients since the filtrate flowing out of Bowman’s capsule into proximal tubule has the same solute concentrations as ECF Passive: Once luminal solute concentrations are higher than solute concentration in ECF, solutes diffuse out of the lumen if epithelium of tubule is permeable to them • Transepithelial transport (transcellular transport) – Substances cross apical and basolateral membranes of the tubule epithelial cells to reach interstitial fluid • Paracellular pathway – Substances pass through the cell–cell junction between two adjacent cells • Active transport of Na+ NHE – Na+ H+ exchange (Na+ - K + - ATPase) Primary driving force for most renal reabsorption Filtrate entering proximal tubule is similar in ion composition to plasma, with a higher Na+ concentration than is found in cells, so Na+ in filtrate can enter tubule cells passively In proximal tubule, NHE plays a major role in Na+ Once inside a tubule cell, Na+ is actively transported out across the basolateral membrane in exchange for K+ A bas-latéral leak channel prevents K+ from accumulating in the cell End result: Na+ reabsorption across tubule epithelium Copyright © 2019, 2016, 2013 Pearson Education, Inc. All Rights Reserved Figure 19.8(b) Reabsorption Copyright © 2019, 2016, 2013 Pearson Education, Inc. All Rights Reserved Renal Transport Can Reach Saturation • Saturation – maximum rate of transport that occurs when all carriers are occupied by (are saturated with) substrate • Transport maximum (Tm) – the transport rate at saturation • Renal threshold – the plasma concentration at which a substance first appears in the urine – Example: glucose blood glucose concentration —> ▪ Glucosuria or glycosuria – glucose in urine elevated genetic disorder where nephron does not make enough carriers Copyright © 2019, 2016, 2013 Pearson Education, Inc. All Rights Reserved Figure 19.9 Saturation of mediated transport Copyright © 2019, 2016, 2013 Pearson Education, Inc. All Rights Reserved Figure 19.11 Reabsorption in peritubular capillaries Copyright © 2019, 2016, 2013 Pearson Education, Inc. All Rights Reserved 19.6 Secretion • Active movement of molecules from extracellular fluid into nephron lumen • Important in homeostatic regulation: K +and H+ • Increasing secretion increases nephron excretion • Organic anion transporter (OAT) family – Substrates compete to bind to OATs due to their broad specificity Na+ gradient used to concentrate a decarboxylate tubule cell (found on both apical and • Na+-dicaboxylate cotransporter (NaDC) inside basolateral membranes in proximal tubule) Copyright © 2019, 2016, 2013 Pearson Education, Inc. All Rights Reserved Figure 19.12 Organic anion secretion Copyright © 2019, 2016, 2013 Pearson Education, Inc. All Rights Reserved Figure 19.13 Renal handling of common substances (1 of 2) Copyright © 2019, 2016, 2013 Pearson Education, Inc. All Rights Reserved Figure 19.13 Renal handling of common substances (2 of 2) Renal handling of key solutes Solute Proximal tubule Ascending limb of loop of Henle Distal nephron Na+ Reabsorbed Reabsorbed Regulated absorption Cl− Reabsorbed Reabsorbed Reabsorbed + Reabsorbed Reabsorbed Regulated absorption or secretion depends on K + intake K Net renal handling (% of filtered load excreted) 1% excreted 1% excreted Low K + intake: 2% excreted Normal K + intake: 10-20% excreted High K + intake: net secretion up to 150% of filtered load 1% excreted Ca2+ Reabsorbed Reabsorbed Reabsorbed absorption Glucose 100% Reabsorbed Blank Blank 0% excreted Urea Reabsorbed Secreted Reabsorbed 30-50% excreted PAH1 Secreted Blank Blank 500% excreted 1 PAH = para-aminohippurate Copyright © 2019, 2016, 2013 Pearson Education, Inc. All Rights Reserved About This Chapter 20.2 Water Balance 20.3 Sodium Balance and ECF Volume Copyright © 2019, 2016, 2013 Pearson Education, Inc. All Rights Reserved The Renal Medulla Creates Concentrated Urine • Osmolarity of urine represents how much water is excreted in the urine – Low osmolarity = high water, high osmolarity = low water • Medullary interstitial osmolarity allows urine to be concentrated – Fluid in descending loop of Henle loses water by osmosis to the medulla – Cells in thick portion of the ascending limb of the loop are impermeable to water and actively transport Na+ out of the lumen into the medulla – Fluid leaving loop of Henle is more dilute (100 mOsm) than fluid entering (300 mOsm) – Distal nephron – water permeability is under control of hormones ▪ vasopressin Permeable to water, filtrate becomes concentrated – Collecting duct – can reabsorb additional solute, filtrate can become even more dilute Copyright © 2019, 2016, 2013 Pearson Education, Inc. All Rights Reserved Figure 20.4 Osmolarity changes as fluid flows through the nephron Copyright © 2019, 2016, 2013 Pearson Education, Inc. All Rights Reserved Figure 20.5(a-b) Vasopressin makes the collecting duct epithelium permeable to water Copyright © 2019, 2016, 2013 Pearson Education, Inc. All Rights Reserved Figure 20.5(c) Vasopressin makes the collecting duct epithelium permeable to water Copyright © 2019, 2016, 2013 Pearson Education, Inc. All Rights Reserved The Loop of Henle Is a Countercurrent Multiplier • Made up of closely associated tubules in loop of Henle and capillaries of the vasa recta require arterial and venous blood vessels that pass very close to each other, with their fluid flow moving in opposite direction —> allows passive transfer of heat or molecules from one vessel to other • Countercurrent exchange systems – Countercurrent multiplier enhanced by active transport of solutes • The renal countercurrent multiplier – Loop of Henle ▪ Transfers solutes by active transport (ascending limb) into the medulla ▪ Results in greater ECF osmolarities peritubular capillaries, dip down into medulla and then go back up to cortex forming hairpin loops that act – Vasa recta as countercurrent exchanger like loop of Henle Water or solutes that leave the tubule move into the vasa recta if an osmotic or concentration gradient exists between medullary interstitial and blood in vasa recta AS blood in vasa recta flows back toward the cortex, high plasma osmolarity attracts water that is being lost from descending limb • The vasa recta removes water • Urea increases interstitial osmolarity Urea transporters in collecting duct and loops of Henle —> help concentrate urea in medullary interstitial, contributing to high interstitial osmolarity Copyright © 2019, 2016, 2013 Pearson Education, Inc. All Rights Reserved Figure 20.7(c-d) Countercurrent mechanisms Copyright © 2019, 2016, 2013 Pearson Education, Inc. All Rights Reserved 20.3 Sodium Balance and ECF Volume • Extra NaCl raises osmolarity – Leads to vasopressin release; → water conservation – Thirst • Aldosterone controls sodium balance – Steroid hormone produced in adrenal cortex – Reabsorption of Na+ (and K + secretion) in the distal tubules and collecting ducts ▪ Targets principal cells (P cells) in the distal tubule leaky channels for Na+ leaky channels for K+ – Increased opening time of ENaC and ROMK channels – Increased activity of Na+ - K + - ATPase – Low blood pressure stimulates aldosterone secretion – High extracellular [K + ] stimulates adrenal cortex – Decreased blood pressure → angiotensin II that stimulates aldosterone secretion Copyright © 2019, 2016, 2013 Pearson Education, Inc. All Rights Reserved Figure 20.9(a) Aldosterone Aldosterone Origin Adrenal cortex, zona glomerulosa Chemical Nature Steroid Biosynthesis Made on demand Transport in the Circulation 50-70% bound to plasma protein Half-Life 15 min Factors Affecting Release Blood pressure (via renin) K +(hyperkalemia) Natriuretic peptides inhibit release Target Cells or Tissues Renal collecting duct - principal cells Receptor Cytosolic mineralocorticoid (MR) receptor Tissue Action Increases Na+ reabsorption and K + secretion Action at CellularMolecular Level Synthesis of new ion channels and pumps increased activity of existing channels and pumps. Copyright © 2019, 2016, 2013 Pearson Education, Inc. All Rights Reserved Figure 20.9(b) Aldosterone Copyright © 2019, 2016, 2013 Pearson Education, Inc. All Rights Reserved The Renin-Angiotensin Pathway • Renin-angiotensin system (RAS) – Juxtaglomerular cells secrete the enzyme renin if blood pressure decreases – Renin converts angiotensinogen to angiotensin I – Angiotensin converting enzyme (ACE) converts angiotensin I to angiotensin II – Stimuli respond to low blood pressure in renal arterioles by secreting renin 1. Granular cells are sensitive to blood pressure when blood pressure terminate on 2. Sympathetic stimulation form cardiovascular center decreases, granular cells and stimulate renin secretion 3. Paracrine feedback from macula densa cells in distal tubule to granular cells: stimulates renin release When fluid flow through distal tubule is high, macula dense cells release paracrine signals that inhibit renin release When fluid flow in distal tubule decreases, macula densa cells signal granular cells to secrete renin Copyright © 2019, 2016, 2013 Pearson Education, Inc. All Rights Reserved ANG II Has Many Effects • Stimulates the adrenal cortex to produce aldosterone • Angiotensin II increases blood pressure through these pathways: 1. ANGI I increases vasopressin secretion 2. ANG II stimulates thirst 3. ANG II is one of the most potent vasoconstrictors 4. Activation of ANG II receptors in the cardiovascular control center increases sympathetic output to the heart and blood vessels 5. ANG II increases proximal tubule Na+ reabsorption ▪ Na+ - H+ exchanger (NHE) Copyright © 2019, 2016, 2013 Pearson Education, Inc. All Rights Reserved Figure 20.10 The Renin-Angiotensin System (RAS) Copyright © 2019, 2016, 2013 Pearson Education, Inc. All Rights Reserved + Natriuretic Peptides Promote Na and Water Excretion • Natriuresis: urinary Na+ loss • Atrial natriuretic peptide (ANP or atriopeptin) – Produced in atrial myocardial cells • Brain natriuretic peptide (BNP) – Produced in ventricular myocardial cells and certain brain neurons • Decrease blood volume – Dilates afferent arterioles, increasing GFR – Decreases Na+ reabsorption in the collecting ducts – Suppress RAS Copyright © 2019, 2016, 2013 Pearson Education, Inc. All Rights Reserved Key words • Na+-H+ exchanger (NHE), epithelial Na+ channel (ENaC), saturation, transport maximum (Tm), renal threshold, organic anion transporter (OAT) family, tertiary active transport, Na+-dicarboxylate cotransporter (NaDC). • aquaporins, medullary interstitial osmolarity, vasopressin, countercurrent exchange system, countercurrent multiplier, vasa recta, aldosterone, cortical collecting duct, principal cells (P cells), renin-angiotensin system (RAS), renin, angiotensinogen, angiotensin I (ANG I), angiotensin II (ANGII), angiotensin converting enzyme (ACE), granular cells Copyright © 2019, 2016, 2013 Pearson Education, Inc. All Rights Reserved