Lecture 20 Actin 2024 PDF
Document Details
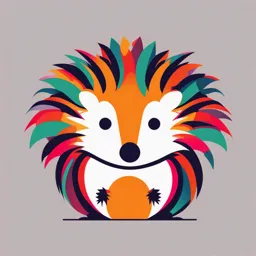
Uploaded by WellRunCopper
University of North Carolina at Chapel Hill
2024
Tags
Summary
This document is a lecture about the actin cytoskeleton, its structure and dynamics, its regulators, the biological structures it forms, and how cells use it. It discusses various aspects of the actin cytoskeleton and includes diagrams and figures illustrating the concepts.
Full Transcript
In this lecture, we will investigate actin, its structure and dynamics, its regulators, the biological structures it forms, and how cells use the actin cytoskeleton. Let’s now turn to the actin cytoskeleton Actin filaments allow cells to adopt a variety of shapes and perform specific funct...
In this lecture, we will investigate actin, its structure and dynamics, its regulators, the biological structures it forms, and how cells use the actin cytoskeleton. Let’s now turn to the actin cytoskeleton Actin filaments allow cells to adopt a variety of shapes and perform specific functions These images show actin filaments (pink) in some of these structures Actin filaments are thin, flexible protein threads Actin filaments are 7 nm in diameter and are less rigid than microtubules Similar to microtubules in that they are polarized with a fast growing plus end and a slow growing minus end The plus end is also called the barbed end, while the minus end is also called the pointed end. These names are based on EM images of actin filaments decorated with myosin motors - which formed a chevron: barb at one end, and a point at the other. Unlike microtubules, the basic subunit is a single actin monomer that polymerizes into a helical chain Also unlike microtubules, actin binds to ATP Actin in the filament makes interactions with subunits in the other protofilament (short pitch interactions) and along the protofilament (long pitch interactions). Actin has four subdomains. Subdomains 1 and 3 are similar to one another. ATP binds at the center of the molecule. This contrasts with the GTP binding site on Beta-tubulin, which sits between the beta and the alpha subunits of different tubulin heterodimers. Thus, the ATP catalytic machinery for actin lies entirely within the protein itself, but hydrolysis is activated through allostery when actin polymerizes. On the actin filament, subdomains 1 and 2 face outward, subdomains 3 and 4 face inward. G-actin polymerizes in the ATP-bound state. Once polymerized, the actin hydrolyses ATP to ADP – resulting in a conformational change that makes actin-actin contacts weaker – and can lead to minus end depolymerization. Actin exhibits a similar polymerization/depolymerization cycle to microtubules but with a few key differences: Monomeric actin binds to ATP and polymerizes by addition to the plus end Upon polymerization, actin’s endogenous ATPase activity cleaves ATP to ADP ATP hydrolysis can be thought of as a molecular “clock” Older actin filaments with ADP are unstable and disassemble from the minus end Note the differences – and similarities - between tubulin and actin Actin can form different types of local network structures, including filopodia and lamellipodia. The formation, regulation, and dynamics of these distinct networks is regulated by distinct sets of actin binding proteins. Let’s look at a few key regulators and examine their activities and general mechanisms. Actin is induced to form various structures based on its interaction with actin-binding proteins and with its own set of motor proteins Actin filaments are shown in red, actin-binding proteins are illustrated in green. Let’s look at a few of these general actin binding proteins, and how they engage and regulate the F-actin polymer. In the images at left, you can see an actin network. You can also see daughter F-actin filaments that grew at a ~70 degree angle from a mother filament. These are nucleated from mother F-actin filaments by the Arp2/3 complex. Arps are actin-related proteins. Arp2 and Arp3 are part of a larger complex that binds the mother filament. Binding to the mother filament serves to activate Arp2/3 (each Arp is an ATPase) – it recruits Wasp-Profilin-Actin complexes, and this recruitment initiates their nucleation / polymerization into the daughter filament. Much of the Arp2/3 network is in the lamellipodia. This animation shows the process of actin nucleation Note: the ARP complex is composed of 7 different proteins, but I don’t want you to memorize all their names. Do know that two of them are, Arp2 and Arp3, are Actin Related Proteins – they have a similar structure to actin and bind and hydolyze ATP Arp2/3 complex activation requires the full complex, plus ATP, plus a mother filament to bind to, plus G-actin bound to the protein WASP, which helps recruit free actin to the Arp2/3 complex. The actin bound to WASP (two of these) is transferred to the Arp2/3 complex – completing the nucleation seed, and polymerization ensues. The daughter actin filament branches off the mother filament at a 70 degree angle. Hmmmmm. Profilin binds to free ADP-bound G-actin and induces nucleotide exchange. Profilin has higher affinity for the ATP-bound state of G-actin. The profilin actin complex adds to the plus end of the actin filament, and profilin dissociates. Eventually, actin in the lattice hydrolyses the ATP to ADP, forming a weaker lattice, and dissociation/depolymerization occurs at the minus end – overall creating the treadmilling behavior of actin dynamics. Note that actin has a conformational change due to ATP hydrolysis. The ATP-bound state is slightly opened relative to the ADP state. These conformational changes affect lattice stability. To be able to open and close, there is a hinge-like region at the base of actin (as shown) – profilin binds this hinge region and induces a more open conformation – amenable for ADP release, and the binding of ATP. Figure depicting the different actin network structures. Lamellipodia are rich in Arp2/3 and form branched networks, while filopodia have parallel bundles of actin filaments. The filopodia filaments are cross-linked, and their polymerization is driven by formins. Many formins will engage the membrane, localize to the tip of the filopodia, and drive actin polymerization. Formins are proteins that promote actin polymerization. Formins are dimers that process along the plus ends of actin filaments, increasing the rate of polymerization. The formin dimer toggles between the protofilaments, sequentially adding actin monomers. To do this, formins bind actin-profilin complexes to deliver actin to the filament plus end. Formins function primarily in regions where the actin runs in long parallel bundles, as found in filopodia. Yet another cool movie! Filament length can be regulated by competing activities of capping proteins and anti-capping proteins (e.g. the anti-capping proteins Ena/VASP as shown above). Filament length can be regulated by competing activities of capping proteins and anti-capping proteins. The structure of a capping protein is shown above, bound to the plus end (barbed end) of an actin filament Cofilin binds the actin lattice that is in the ADP state. Binding happens cooperatively – ie cofilin binds this lattice, induces some conformational change in the lattice locally – and this altered lattice structure has high affinity for more cofilin molecules to bind. As you get more lattice conformational change, the intersection of the region bound by cofilin and the region not bound by cofilin experiences strain. This strain leads to severing at the junction points. Severed actin filaments can depolymerize and/or be used as seeds for more nucleation. Different drugs can be used to study actin dynamics and function in cells. The cell cortex is a region of cytoplasm just beneath the plasma membrane made of actin filaments and actin cross-linking proteins The cortex provides mechanical strength to the cell membrane and helps cells maintain the cell’s shape One of these cross-linkers is the protein filamin Filamin cross-links actin filaments into a meshwork The image at bottom (A) is an image of highly metastatic melanoma (skin cancer) cell – note the membrane bubbles or “blebs” that protrude from their plasma membranes This cancer line has a mutation that inactivates filamin, as a result of these blebs that constantly inflate and deflate from their membranes these melanoma cells exhibit robust motility Motility is a hallmark of metastatic cancers – they aggressively crawl from the primary tumor to colonize other sites within the body Expression of a normal copy of filamin in these cells (panel B) rescues the membrane bleb phenotype and reduces metastasis when injected into mice This migrating cells is expressing GFP-actin The brightest regions correspond to regions of the cell with the most actin Note the leading edge is rich in actin and the membrane is “ruffling” This structure is called a lamellipodium Let’s next take a closer look at the structure of actin inside the lamellipodium These images were acquired using a specialized TEM protocol that removes the plasma membrane while preserving the 3-dimesionality of the cellular ultrastructure (A) is a high magnification view of the lamellipodium of a crawling cell with the leading edge at the top Each of the filaments is a single actin filament – thousands of actin filaments can be seen oriented with their plus ends terminating at the cell margin (B) and(C) show higher magnification insets of the boxed regions further in from the leading edge Notice how many of the actin filaments terminate at other actin filaments in a “Y”-shaped junction? Polymerization of actin filaments at their plus ends produces the force that pushes the leading edge forward The growth of thousands of individual actin filament produces sufficient force to push the membrane These actin filaments are organized into a branched network by the actions of several proteins: The ARP (actin-related protein) complex is an actin nucleator Capping protein binds to growing actin plus ends to stop their elongation (keeps individual filaments short) Cofilin is a protein that binds to older actin filaments (remember – as actin filaments age, they hydrolyze ATP to ADP) and depolymerizes them The order of events: 1. ARP is inactive until it binds to the side of a pre-existing filament (we’ll call this the mother actin filament) 2. ARP binds to a mother filament and nucleates the growth of a new daughter actin filament at a 70- degree angle to the mother filament; ARP stays bound to the mother and anchors the daughter filament in place 3. The daughter actin filament grows for a short time towards the leading edge and exerts force to push the membrane forward 4. Polymerization of the daughter filament is stopped by binding of capping protein 5. Meanwhile further back from the leading edge, the mother filament is hydrolyzing ATP to ADP as it ages which acts as a signal for cofilin to bind and trigger its disassembly 6. Monomeric actin is released from the depolymerized mother filament, it binds to ATP, and diffuses to the front of the cell for the process to start again How do cells regulate which actin structures they form based on cell type, region of a cell, and over time? Signaling pathways involving small GTPases regulate many types of actin structure formation. Activation of Rho, Rac, or Cdc42 trigger different pathways that drive distinct F-actin network formations. Regional activation of these GTPases can lead to distinct actin structures in different regions of a cell. These GTPases are membrane associated, and thus drive these network formations close to the membrane. Growth cones are critical for neuronal cell migration. Microtubules and F-actin dynamics are coordinated in this process, and require microtubule-motor based delivery of factors to the growth cone, as well as co- regulation of actin and microtubule dynamics. Spectraplakins are large proteins that co-bind microtubules and F-actin, cross-linking them and coordinating they dynamic behavior. Spectraplakins play critical roles in processes such as neuronal migration, required for brain development.