Lecture 2-1 PDF
Document Details
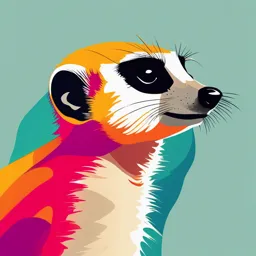
Uploaded by VerifiablePrologue3527
Tags
Summary
This document provides a lecture on neurobiology, specifically detailing the process of repolarisation and the role of sodium and potassium channels in maintaining membrane potential. The document also discusses synaptic transmission, and how neurotransmitters and receptors function.
Full Transcript
WEBVTT Into a repolarized state we have a state the loss of cations, in this case now it's potassium leaving our intracellular space and now we are going to repolarize. We're going to come back down into a negative state along our resting or along our membrane. Potassium channels are very slow in...
WEBVTT Into a repolarized state we have a state the loss of cations, in this case now it's potassium leaving our intracellular space and now we are going to repolarize. We're going to come back down into a negative state along our resting or along our membrane. Potassium channels are very slow in general, and there are different types of sodium channels. Obviously, I'm trying to kind of generalize this when I'm explaining it. But in this case, potassium channels move a little bit more slowly than the sodium channel so that sodium was open, floods sodium into the cell, and then kind of almost immediately is inactivated Well, once that potassium channel opens, it's slow to open and then it's slow to close. So we're just constantly losing potassium to the point that we overshoot our original resting membrane potential. And so our cell membrane becomes even more negative than it normally kind of lives at baseline. So we call that hyper polarization. So you get a nice little dip If you're monitoring kind of the electrical signals of this. And then how we get back to resting and brain potential is your sodium potassium pump. So we have all this sodium kind of sitting intracellularly of course we typically have more sodium outside of the cell. That's where sodium more so lives. Its plasma level is usually like 140. So we really don't want all that sodium in the cell and all of our potassium outside the cell. We want to get back to our homeostasis. And that's where the sodium potassium pump will kick in. We're going to pump our sodium pump. Back where it likes to be and kind of bring some of that potassium back in. So we're going to exchange the two so the pink guy in the center is kind of activation of that. So you can see it trying to exchange sodium. And potassium so that we can get back to resting membrane potential. The other thing you'll note here is that our sodium channel The configuration of that has changed a little bit. So you can see our little tail that was kind of closed off the channel when that's receptor was inactivated. That has now kind of moved back to its original position However, that channel is not open. So you can see the interior part of that channel has closed off. So we're still not letting sodium ions in. That's actually a different conformational state for that sodium channel. So now it's in a closed state. Generally, the difference is that in this inactivated state. This channel should not be able to respond to a stimulus. Once it gets to the close date. It's now ready to respond to the next stimulus. If that membrane potential gets back to that negative about 55 level. This is also very generalized. This is a very complex process of interaction between electrolyte levels inside and outside of the cell So, you know, this is not just a kind of a black and white description of this, but in general. There's some changes that we expect if we have electrolyte abnormalities. So for example. With calcium, hypocalcemia. That prevents sodium channels from closing between our action potentials. If our channel doesn't close then we're going to sustain our depolarization, right? We're not able to bring that membrane potential back down to a negative level and for that Sell really to rest So that has the effect of kind of this repetitive firing Which is similar to what you get with tetany. A titanic response. Hypercalcemia decreases your cell membrane permeability to sodium. So if we can't really get sodium in, then we're not really able to excite that neuron. Potassium hypokalemia means you have a more negative resting membrane potential. The reason for that is because if in the setting of hypokalemia, so too little potassium in our plasma. The cell is going to be it's going to be kind of triggered to give up potassium. So it's going to lose potassium in an effort to kind of restore potassium level levels in the plasma. And if you lose potassium, just like when that potassium channel is open. And our membrane potential went way down into the negative it has the effect of hyperpolarizing your neuron. Or your cells. So you'll have decreased membrane excitability because of that kind of extra negative state, it's going to be a lot harder to get all the way back up to threshold and then generate an action potential. Sodium channel blockade can prevent getting to threshold potential and getting that action potential generated as well. If I can't get sodium in. You know it's hard to you know it's hard to continue that process on. So the synapse. So basically, obviously, you guys all know when you generate an action potential, it's going to travel down that neuron. It's not just kind of a one and done. It's going to get to the end of that. Neuron and then cause something to happen to communicate with the next neuron. Or other cells and tissues are going to have kind of the same effect. So enter the synapse. We have a presynaptic cell with a membrane. And a postsynaptic cell across a synaptic cleft. So that presynaptic membrane, you guys all know there's usually going to be vesicles packaging our little neurotransmitter hanging out down at the bottom. If we have calcium channels at the bottom of that membrane. You know, when calcium binds, causes a voltage gated change that vesicle can now merge with the cell membrane and dump out neurotransmitter. There's also going to be reuptake pumps along this presynaptic membrane. The reuptake pump is usually used to help bring that neurotransmitter back into the presynaptic cell. Or if the neurotransmitter is broken down. It'll bring pieces of that neurotransmitter back up into the presynaptic cell So that cell can make more of that neurotransmitter. So we see this with catecholamines. We see this with like acetylcholine coming out of a neuron. At the neuromuscular junction, acetylcholine is broken down in the cleft and then you'll have part of that acetylcholine go back up presynaptically by reuptake pump merge acetate and choline to form acetylcholine. And then that's packaged again and sent back out. And so you're getting this ending of this neuron Saxon's getting that first action potential, it's going to receive that afferent action potential And get ready to kind of change this area so that we can now Ignite or start an action potential in the postsynaptic cell and send it forward. Postsynaptically, we're going to have neurotransmitters now flooding this area and they can bind receptors on the postsynaptic cell generate an efferent action potential which is sent down the cell to communicate to the next one. What you can't see from this image, but there are And maybe you can kind of appreciate there's kind of a lot going on on this cell you can see compared to the presynaptic cell. But all of these like proteins and other like different receptors receptors and structures that are kind of along the membrane in like right underneath within the cell is what we call postsynaptic density And it actually helps maintain homeostasis and integrity in that particular environment. So you can see all of that better on this image. Where it's all it's where it's all cartoonized, we'll say, is where it's a little bit easier to see exactly what those mechanisms are. So the synapse, modulation is when we have some change in synaptic function. So it could just be signaling from cell to cell It could be some change in depolarization or change in response. There is a delay. So again, it's very, very quick, but there is a slight delay between you know, when you're thinking about one neuron communicating with the next. It's going to take a moment for that neurotransmitter to be kind of you know the vesicles diffuse with the membrane, release that neurotransmitter neurotransmitter to diffuse down to the postsynaptic cell across the synapse bind and then generate the next action potential. The reason for that delay, also it's beneficial in that it can help reduce like fatigue or just constant triggering of the postsynaptic cell. Fatigue can also occur If the conditions are right for repetitive stimulation. It can reduce the response of that postsynaptic cell. So if you think about We talked about down regulation. Of receptors and how if a cell is just constantly bombarded to respond, it may start to undergo genetic changes that say, I'm going to respond less. I'm going to reduce the number of receptors I have up there that are looking for a response. And I'm going to reduce my sensitivity to something binding to a receptor that would have a response. And then fatigue can also be related to depletion of neurotransmitter. So this is kind of what you would see As an example, in the heart failure patient. That has catecholamine depletion. If you think about that patient is just constantly stimulating their sympathetic nervous system. To try to get the heart to pump and try to maintain blood pressure and things like that and perfusion. Well, that constant stimulation in that case will eventually lead to a depletion of those catecholamine stores there. Trying to pump out so much norepi and epi to have an effect that eventually you know the cells just can't keep up Like, would that be another comparison in the older person as well? Like they're releasing or epi all the time too like in their um vasculature I would say if they have a disease process going on, I don't know. Okay. I don't know that how common that is with just normal aging, but for sure if there's acute illness or even a chronic illness that is just constantly you know, they're trying to compensate or something like that then possibly Post satanic facilitation so This is something we do. You might have done it in the ICU when we're monitoring neuromuscular blockade is if you have a patient with zero out of four twitches if you're using qualitative. Neuromuscular peripheral nerve stimulation, I should say qualitative and you're looking for a count So you're counting, you're looking for four twitches. So let's say you have zero twitches. Basically, in that instance. You have so much. Neuromuscular blocker sitting on receptors on the post synaptic membrane which is your muscle that there's nothing that can stimulate that muscle to have a response. With post satanic facilitation If we, instead of just hitting your, you know. Train a four button to stimulate four quick times If we now hit the tetany button and hold that for a period of time. What we're doing is we're stimulating that nerve to release just a lot of acetylcholine. And we're pushing out so much acetylcholine. So this first bullet point, repetitive stimulation of the presynaptic terminal. We're releasing so much neurotransmitter that now We have enough neurotransmitter to compete with rock uronium sitting on that membrane And if we have enough to push that rock uranium off of the receptor and acetylcholine sit on the receptor. Then we might get a muscle twitch. So the idea with post satanic facilitation is to stimulate that presynaptic terminal flood the area with neurotransmitter And then the idea is that the receiving cell, that postsynaptic neuron. Is now going to have an exaggerated response. And we'll definitely talk about this when we get to neuromuscular blockers but even beyond the synapse, you've got like cell synapse, cell. If you kind of spread kind of along the membrane outside of the synapse. There's extra receptors out there And they're very sensitive to neurotransmitter. And so if you flood that synapse area with neurotransmitter and some of that neurotransmitter is going to kind of spread out it's going to target actually extra receptors that don't normally get hit with neurotransmitter. So that's the idea of post titanic facilitation. Neuronal responsiveness. Factors can affect the ability to respond. So beyond just kind of fatigue and neurotransmitter depletion or depletion increasing neurotransmitter and getting an exaggerated response If you have changes in pH, That can affect the excitability, alkalosis being increased, acidosis decreasing. Hypoxia can decrease. Excitability. As well. Any questions? So far. Sam, we're glad you made it. Receptor pharmacology. I know this slide's a little bit busy and I added all kinds of things to it to make it look like how I wanted it to really look. But this is a nice little zoomed in picture of your phospholipid bilayer. And as you guys know, you've got these polar heads and non-polar tails. And those nonpolar tails like each other, right? They're not going to repel They shouldn't be charged or anything like that. So they're going to hang out together and that means your polar heads are on either end. And then we have receptors and channels and all kinds of things in the middle. You've got some steroid ions kind of hanging out Sorry, steroid molecules kind of hanging out on the inside oxygen here. I threw an oxygen molecule here. You can see that can easily diffuse SIVO fluorine molecule just to be fancy. I threw that in that can easily diffuse across the membrane. But we have bigger things like ions, potassium, drugs. That actually need a channel to get through that membrane or carrier of some sort. Glucose is another one. So not everything can just readily across. We need it to be lipid soluble, small enough like a gas, something that could easily kind of diffuse. And then down here at the bottom, you see all these different proteins these strands These filaments, that's that postsynaptic density that helps maintain kind of the integrity of that area. Okay, so the receptor, we said receptors are proteins And they can be other substances, but generally they're proteins and they bind Either in endogenous chemical or a drug. Properties of the receptor. So sensitivity is the concentration that you need for cellular response. We'll really start looking at sensitivity when we get a little further in this lecture. Selectivity just means can your molecule fit into the opening of that receptor? So we can see here A3 fits on this top receptor A2 looks like it would need to be kind of rotated in order to fit A1 does not so A1 has no selectivity. For this middle receptor. Specificity is a cellularly determined response So depending on activation of a receptor, the response you get from that is going to depend on the tissue that it's in. There is a spectrum of weak to strong bonds that are formed. So whatever molecule it is or endogenous chemical. It's going to form a bond with your receptor. Receptors are often somewhere along the membrane kind of could be like inside the membrane or on the surface Or they can be intracellular. So once we give a drug to when it actually has an onset It's going to, of course, like we said, get to that bio phase or that area where it's going to have an effect And then it's going to have to orient itself into the right You know position and attached to that receptor. Typically, this involves hydrophobic bonding, hydrophobic bonding and why hydrophobic bonding. If you guys remember if you guys from last week we talked about To be pharmacologically active, we did not want that molecule to have a charge. If it didn't have a charge, if it wasn't ionized, if you remember our HA and B. Forms for a weak acid and a weak base that uncharged molecule is also lipophilic. And lipophilic and hydrophobic are basically the same. It's not water loving. It's not water soluble. So this is going to be a nonpolar molecule that's going to react with another nonpolar molecule being your receptor. So that is hydrophobic. Bonding. Conformational change will occur. So meaning when you have a molecule that fits perfectly on that receptor. Something is now going to change. So the receptor may change. It may be attached to some other protein that changes in some way. But basically, there's going to be some sort of like shape change that now is going to cause a response in that cell And that might easily spread to other cells and the tissue. A-acceptors. Aceptors are endogenous proteins that are alternative drug binding sites. So we talked about albumin. There are other proteins like alpha-1 acid glycoprotein. Beta globulins. If our drug is floating around in the plasma and holds on to albumin It's very much like our drug floating in the plasma and then getting our effect site cell in binding to receptor. So any other substance that can kind of bind onto that protein or sorry, bind onto the drug molecule but is not our desired receptor is called an aceptor. So we consider albumin an acepter. These are different types of receptors in drawn form. We will talk about you know these will kind of mention the basic types now But as we go and start to learn more and more about different drug classes, we will revisit A lot of these receptor types Especially when we get to our anesthetics, a lot of our drugs are G protein coupled. A lot of them are ligand gated. And voltage gated. Fun fact, the G protein couple receptor crosses a membrane seven times in this kind of ribbon-like formation So anytime you see a receptor on an image and it looks like that and it crosses seven times it is g protein coupled Chemistry application. So you guys so you guys ages ago when I taught pharmacology It always came after chemistry physics. And then when I came to Mary Baldwin. Our chemistry physics was kind of flipped. And so I couldn't really like do the review when they didn't have the chemistry physics course yet, but now you guys have had it. So I technically could have asked you guys these questions but Hopefully this is review. So dipole-dipole interactions. Which one causes considerable energy to break and helps hold globular proteins together, like an enzyme, what helps an enzyme keep that kind of weird twisted shape is hydrogen bonds. So hydrogen bonds are one of the more common bonds you would actually see in pharmacology. So a drug may bind to its receptor with a fairly strong interaction and that would be from a hydrogen bond Sorry, from the recent, I'm looking at the question in the chat. Yes, you did chem physics first. We like that. For the receptor slide… If something lipophilic nonpolar, I thought it did not need a receptor And can easily diffuse across the membrane. So if your drug molecule is too big, it can be hydrophobic, but it's not going to move across the membrane. It's not going to just diffuse like an oxygen molecule. It's still going to need assistance, if that makes sense. So some drug molecules, they interact similarly to this or some receptors interact with whatever molecules binding sometimes as sometimes with hydrogen bonding. Intermolecular forces of attraction I'm just kind of receding what you guys already which dipole interaction is the weakest type? That's your van der Waal london And this is also very, very common. It's probably the most common is to have more of a weaker attraction It depends on the drug and it's not as simple as saying, well, every drug with a longer duration of action must have a stronger bond it's much more complex than that. But a lot of our drugs kind of have this weaker, like it'll kind of hold on to the receptor and then it'll kind of float away from the receptor. Or it can easily be knocked off of the receptor by something else. Next one, true or false, nonpolar tales, love interacting with water molecules. We know that is false. That are non-polar tails are hydrophobic. So they're going to interact with each other. Hydrophobic interactions are especially important for lipid soluble drugs. Next one. Strongest molecular bond, covalent bonding This is the least common. Type of drug receptor interaction you'll see. So one example of this is phenoxybenzamine. Typically, when we do, it's very rare, but typically when we do talk about a drug being non- competitive. Non-competitive. That means it's going to bind via a covalent bond. So phenoxybenzamine is non-competitive as it sits on an alpha receptor. And what that means is you can flood the synapse something that antagonizes phenoxybenzamine. So, you know, in this case, it would be a catecholamine And it's not going to be enough to knock phenoxybenzamine off of its receptor. It has that strong of a bond. So this particular drug Lasts for days and days and days. And so that actually that duration of action And the ability to eliminate phenoxybenzamine is quite long. So this is an older drug. Not really sure how often it's really used. Because of that. Really like a limitation in a way. But there are some mechanisms or some drugs that It's kind of preferred that you have covalent bonding like aspirin holding on to a platelet. For the life of the platelet like you usually want that prolonged effect in that case. Yes. Quick question. So you said the phenoxybenzini isn't used You serve as an alpha blocker. Right. So why did they… I mean, I know Alpha blocker, but what would the patient, why would the patient need it, I guess? Why would they need it? Well, it is an old drug. Like, yeah. And so I believe, I mean, I've never even seen or heard of it being in use. We still teach it a little bit. I think because i think because science advanced and they came out with shorter acting alpha blockers that those are probably safer to use. So for example. You know, if you had a patient with an IV where you had it infiltrated and you were giving phenylephrine through that iv massive issue. You know, instead of giving something like phenoxybenzamine to kind of counteract that, that could last Just for way too long. You would give something shorter acting that really has the same mechanism But less risk for side effects. So the desired effect for a prolonged period of time might be helpful depending on the indication for why you're giving the drug. But the side effects for a prolonged period of time is generally not desired, right? It's not what you want. So that's probably part of that. Reasoning for like, let's do let's find a drug that does not bind so strongly And use that instead. Something that is actually reversible When you think of non-competitive, generally we will consider that drug irreversible. There's really not much you can do to kind of help reverse desired effect or clinical effect. And so that's the problem with it. Thanks. Yeah. Dr. Elmar, I just consulted Dr. Google, and I guess it was used specifically to treat pheochromocytomas. Yeah. Probably just a prolonged condition that's going to be there. But I know Dr. Owens has mentioned Omeprazole and the proton pump inhibitors. Seems like everybody's on those now. So that's a much more contemporary example of the The covalent bonding. Yeah. Dr. Elmore, with the strength of bonds, I feel like I've drilled it into my head that ionic is the strongest. Is that like just the wrong application or context for the type of bonding that we're talking about here or For receptor interactions, yes. I mean, you may get some ionic bonding there may be drugs that are bound that way, but as far as it's usually for us kind of the dipole-dipole. Interaction, the intermolecular forces going up to maybe more so covalent And that's really just dependent on how drugs are kind of formulated, manufactured You really don't want it to be that irreversible in general. To have that complete you know, ring and such but I would assume it is possible there could be substances out there that are It's going to form that kind of connection. Thank you. Any other questions? Okay, a review of stereochemistry and chirality so With the 3D molecular structure. You know there it's that basically the stereochemistry basically describes that structure for our enzymes, our sugars our anything that, you know, proteins, anything that's in the body, it's not going to be this flat two-dimensional shape. It's going to have More structure to it. It's going to affect its ability to bind a receptor bind a protein And then what happens afterwards? Chirality describes molecules with 3D asymmetry so If we think about some of our drugs being a mixture of kind of these different molecules that it's one drug, but they kind of have different enantiomers in place And a Nancy Mirror is just this mirror image that can't be super imposed. So that has it gives that molecule chirality So you can see besides drugs you know we have a lot of endogenous substances that are considered chiral compounds. Two of those enantiomers in a mix of 50 50 proportion is a racemic mixture. So some of the drugs we give Racemic mixtures. So isomers. Generally, we have an S sinister and an R rectus isomers. I'm assuming those are the Latin terms, which I should know because I took Latin for five years. Way back when, just a FYI for you Ketamine. Ketamine is a racemic mix generally, at least the formulations we tend to have in the US. Are a racemic mix of ketamines. We have the two isomers, S-ketamine, which is the desired form and then our ketamine Which generally gives you all the side effects like it being a hallucinogen. As ketamine is more potent, it's less likely to cause delirium. And so it is preferred. So ideally we You would really just want the esketamine formulation And in some facilities and a lot of countries, they have isolated out S-ketamine. And I think they're using it a lot in psych if i'm… I believe, and it's actually called esketamine But that's the ideal formulation because it's giving you kind of all those benefits of the mix. Now, like I said, in general, at your facility when you Grab a vial of ketamine, it's going to be the cheap racemic mix where you will now split between giving enough for an anesthetic and unfortunately experiencing the patient that sees purple hippos after surgery. So another example is levobivacaine and arbupivacaine. Intravascularly, if you give too much of the arbupivacaine. Form in the molecule. It is very cardiotoxic. That patient is likely going to have an arrest. Levo bupivacaine, if some of that goes intravascularly. A little bit less likely to be as toxic. Um… accidentally put a patient to a was not fun at all i would imagine Helena, did I answer your question before? Hopefully, with the ion. Okay. Yeah, you did. I was wondering, though, is this like the Levo the same as I thought it was D instead of R for the like dx dexville for the right. Or right rotating. Okay. No, this is just the name of the drug. It's not like the chemical term It's just the actual name. Like if this was isolated, it would be marketed as Leva bupivacaine. But it's really spupivacaine. So R is to the right and S is left rotating. Um… I think so. I don't you guys would have to look that up for me. Okay. I just have an SNR cinema. During rectus isomers. Okay. In the chat, we're getting yeses. Let's see. Let's… Let's stop here and we'll come back at 10. And take a break. Totally didn't mean to stop sharing my screen. Okay, let's get started again. You guys looking that up because it's been forever since I've had both Latin or chemistry. So yes, your R is right. S left. Receptor states. So binding a receptor Like I said before, causes some sort of what we call conformational change. So it's going to change in shape. So here you can see ligand-gated ion channels where a ligand will bind to that receptor. And then it will change some sort of shape, allowing some molecule or ion or something to pass through that would not otherwise be able to pass through the cell. So there can be different states Sometimes we'll look at that much detail when we go through a drug class of whether or not molecules in an inactivated state, an open state or things like that. But can affect the pharmacologic effect that you see. Receptors receive and transduce signals. Once a ligand binds. That signal that is now activated by that receptor can be relayed. So sending it on to the next cell, it can be amplified so it can increase whatever the intracellular response normally is. Or it can be integrated into a biochemical pathway. Different receptor types, G protein coupled, which I mentioned. Before ligand-gated ion channels, we'll see a lot of those. Voltage gated ion channels. Just like our sodium channels kinase linked, meaning that they are linked to an enzyme And then nuclear receptors. So we have different hormones and molecules that affect genetic expression. Much like your corticosteroids. So we'll start with G protein coupled receptor. So you have a receptor protein, G protein, and then some effector mechanism that's kind of the three components of this system. So your ligand would bind extracellularly. It causes a change or an effect intracellularly. And then generally you'll have a like a conversion of here guanosine diphosphate to guanosine triphosphate. And then it causes all of these other potential effects Depending on the cell or depending on the tissue that it's in. Might cause an increase in cyclic AMP or an increase in calcium. Or something like that. Definitely depends on the cell, whether or not you get activation in that cell or inhibition. If there's some sort of production of an enzyme or if it's going to target some other sort of response. Ion channels. So here you can see kind of the different conformations that an ion channel might take or ligand channel might take Ion channels, generally the ion is going to flow in favor of a concentration gradient. So sodium, calcium, and chloride tend to go into the cell. So if you have a calcium channel, chloride channel. It's going to send that ion into the cell. Potassium is going to go out. So we consider sodium and calcium channels depolarizing and excitatory. So if we're bringing cation into our cell. That is going to increase our membrane potential Very similar to the very similar effect of depolarization we saw with an action potential. Chloride coming into the cell as an anion or the loss of potassium as a cation are going to both have hyperpolarizing effects And generally are inhibitory. To the cell. So you might have a membrane polarization change. You might have a ligand that binds. Or an ion channel can be coupled with a G protein And then that starts the stream of effects. So there's different possibilities here. Ligand gated ion channels, typically when a ligand binds, it is going to send ions in and sometimes ions back out as well. So very similar to what we've already kind of talked about, but it's basically helping to increase the permeability um to of that ion's movement across the membrane. You should know these, excitatory versus inhibitory examples. These obviously are not every ligand gated ion channel or receptor system in the body, but these are the ones we will talk about the most in farm one and two. So acetylcholine and nicotinic receptors are excitatory. Glutamate is the neurotransmitter and binds to either NMDA, AMPA, or kinate. In an excitatory fashion. Glutamates, the primary excitatory neurotransmitter in the central nervous system. So we'll talk about glutamate a lot. Over the summer. Serotonin and serotonin receptors, which are often abbreviated 5-HT, Those are excitatory. On the inhibitory side, it's GABA and GABA receptors. So gamma amino butyric acid And then glycine and glycine receptors. Gaba is predominant in the brain, glycine in the spinal cord. So we will talk about those as well. When we get to. Anesthetics. Okay, voltage-gated ion channels open and close when we have some sort of change in our membrane. We see these a lot with neurons, muscles, endocrine cells. And then typically named for the ion that goes through so In this instance, sodium channel, this is where our local anesthetics work. To… help block the… Oh gosh, I just lost my term. Help block the response to a noxious stimulus. A variety of cellular responses can occur. So when we do activate a receptor and cause some sort of cellular response, tissue response, et cetera. There's a lot that can happen kind of downstream from that initial drug molecule or whatever is attaching itself to the receptor what happens further on in the cell can be can vary. So you might have some change in genetic Expressions. So you might trigger a cell to for example produce more of a certain protein cellular metabolisms, it may cause a cell to become more or less active. And then cellular changes such as migration, function changes or even apoptosis. I want you, and I forgot to do this cross out propofol and GABA. In ketamine and MDA, just like put a line through those examples Because I can't remember why I put that there to explain it to you. And I forgot to go back and delete it. Um so obviously different responses can occur. Chain reactions can occur. I mean, there's really, this slide's very self-explanatory, but I want you to cross those examples out because I do not want to confuse you and I can't figure out why I said that, why I put that on the slide. Receptor upregulation, we mentioned this Did I mention up and down regulation or is this new? You know, when I was thinking we had that last week. We did not. Okay, so receptor upregulation is when we have an increase in the number and sensitivity of ourselves. So when I was kind of talking about this before, sorry, I was thinking for some reason that we Talked about it last week, but I guess we hadn't So basically, if you continuously kind of I'm sorry, if a cell is used to a certain kind of baseline amount of activity. And it does not receive that baseline amount of activity or what it's used to. Then it is going to undergo genetic changes to help get the response that it's looking for. So in this case, it may increase the number and sensitivity of receptors along the membrane to try to respond to an agonist. Because it is looking for some sort of effect. So it wants to increase the frequency with which that cell is going to have a response. So an example pharmacologically is if you have two little agonist in an area that Cell is going to upregulate receptors. Or if you are constantly blocking the activity of that cell. So it's bombarded with an antagonist. Then you may see upregulation in response to that. Constant antagonism. It wants to respond. Down regulation is the opposite. Decrease in the number and sensitivity of receptors. So again, that cell is going to undergo genetic changes to decrease the response, decrease the receptors because it's now, you know, it feels like it's doing too much And so in an effort to get back to like a homeostasis. Or baseline level of functioning, its goal is going to be to decrease cellular response. Question. Sorry, David, I missed your question before. Um… Yes, you're right. Propofol increases the effects of GABA. I mean, it's a GABA agonist. So maybe that's why I put it, but it's still confusing. It sounds confusing because I know what GABA does. Yes, I was saying that propofol being an agonist, ketamine being an antagonist. That's probably why I put those examples in there. But I still kind of feel like it's confusing. If you guys still want to cross it off, just cross it off. Okay. Hopefully that makes sense. Upregulation, downregulation. You know. If it's expecting a certain amount of a response. Then it's going to increase receptors to get that response if it needs to. Or vice versa, if it's getting too much action, being stimulated too much, then you might see a decrease And the number and sensitivity of those receptors so that it can decrease response. Mental break. It's a break. If you could have a superpower What superpower would you have or what is your favorite superpower? I would want to have a photographic memory. It's good. Teleportation. A couple photographic memory to slow down and sleep more okay radiology bypassing a CT machine. I don't know what that says about you. Carlos. Reading thoughts. Okay, teleportation, a lot of that. If I can make any food I wanted to appear. Yes. It's a good one. Oh, you just hate waiting for results. Flying. I think flying would be cool. Time travel. Any particular moment in time you would go back to or forward to? If you could predict the stock market, then I guess you wouldn't be here. Um… Invent whatever you wanted to invent. Oh, exactly. August 2027. I wonder why. Clone your dog. Interesting. Very interesting. Be a wizard okay All right. Very good. Now we are going to kind of get into more of that receptor or kind of more so into the clinical effects. And once you have your molecules react with your receptor? What is our clinical response going to be? How do we get to That desired response. So, as you guys all know, when we study drugs, when they go through trials. Whether or not it's on humans, but we're not always going to study a drug in every single type of person that we would actually give that drug to. So in human trials, it's usually going to be a normal, healthy adult. There may be some exceptions to that. And I shouldn't. Forgive me for using the word normal But just generally, it's going to be someone probably within the age range of 20 to 40 And no health issues or something like that. So that requires us to individualize drug therapy. Because, you know, what trials say about a clinical response is not necessarily what we will see in actual practice when we start to change up that population from what was originally studied. So we have to titrate because we have to oftentimes we may underdose or overdose a patient Thinking that they're going to respond the way a textbook says they should respond. So we do have a lot of variability within a population. You're all about the science. Behind these drugs, you're learning about the science behind farm in general, but when it comes time for you to put a plan of care together. And assess the response in your patient, assess the side effects in your patient. Learning how to titrate based on that, that's where you start to get a little more into the art of pharmacology. So graded dose response. You can see these dose response curves. Generally, you're going to have some representation of dose on the x-axis, usually concentration of the drug Or log of concentration or something like that. And then response is often going to be on the y-axis. Some of the graded dose response curve It shows you a change in response with an increase in dose. So you can they will plot these as they do trials to determine what drug concentration needs to be achieved in a certain tissue to get a maximal response, usually where that's going to plateau. So as we get up here to this plateau. You know this is the highest response we're going to achieve giving any greater dose is not going to you know give us any more of a therapeutic response. Additionally, and this is plotted linearly versus logarithmically. So additionally, you can look at the slope which is going to represent the number of receptors that need to be bound before you get that clinical effect. So if it's a very steep slope. Generally, we need the majority of the receptors bound by that drug In order for it to have an effect. And we see that behavior a lot of times with anesthetics like inhaled anesthetics, neuromuscular blockers etc. Often you'll see quantal dose curves being plotted out Usually this is kind of a more binary response like does a certain drug concentration have an effect or not have an effect? And so it's like this all or nothing. They're trying to basically figure out How far can we get before that drug starts to have toxic side effects, maybe even lethal effects. So they're trying to figure out safety margins. And so they will Plot drugs in this all or nothing sense. So for example. When studying propofol, they found that you know like a half milligram per kilogram was enough to start to get hypnosis in a lot of patients but then A milligram per kilogram was enough to get more patients into a hypnotic state Two to three everybody was in that hypnotic state. So now you've got two to three as this effective dose. And I'm sure if they were to kind of to keep going, they would start to see more side effects maybe heading towards like a toxic type level. So potency and efficacy. Again, we're still looking at dose response curves. So drug concentration on the bottom response along that y-axis for efficacy, it's the ability of the drug to get to a maximum clinical effect. So if we know you know, we have a drug that can cause 100% the desired clinical effect It has full efficacy or maximal efficacy Some drugs may only get to 50% response before they plateau. Or even less. And so that drug would have partial efficacy. So as you look at these, if you compare these three drugs a b and c A has greater efficacy than C, obviously. For the same dose. Potency over here on the right is the concentration you need to cause a specific response. So if you look at drug A, at about 50% response, you would only need, let's say I mean, I know this is a different scale, but we'll just say it's a milligram of the drug. B, you might need about five milligrams. C, you would need 10 milligrams. So obviously A is has the greater potency So as you go left on the x-axis. You're going to increase in potency. Further diving into potency, we can look at the EC50 or the C50. So you sometimes will see it written both ways. That's the effective concentration at which you get a response in 50%, 50% response. So, you know, it's kind of a measure of potency Sometimes they will actually You know, in a drug book with a lot of detail they may actually give you an EC50. Effective dose ED50 is the dose where you would get your desired response in 50% of the population. So ED50 is the beginning of our identification of the safety margin. So they'll look for the ed50 And then get down to the LD50. Now, obviously. Ld50 being lethal dose is going to be what they use in animal studies. Hopefully not in human trials. In human trials, they look for the toxic dose of the TD. But the way they do calculate therapeutic index is the division of LD50 divided by ED50 gives you your therapeutic index. Yes. So question on that. Just so that I'm clear, when you're looking at the therapeutic index, they do not use the toxic TD50. They use the LD for animals for understanding the therapeutic index for humans. Yeah, I'm not sure if there are some drugs out there where they may have calculated the toxic dose, but generally it's LD50 divided by ED. So if we were looking for the therapeutic index I mean, unless they had a trial where they had deaths, then unfortunately, if they had a human with it, then yeah, that's the one they would use. Sure. I was just getting mixed up why for the therapeutic index, we wouldn't use the toxic dose 50 over ED50. Is that a thing or no? There can be drugs that do. It's just, I mean, this is the definition I have. I can't tell you if every single drug manufacturer is using LD versus TD, just know this particular equation because that's generally That's the ratio that is generally used. I can't say that that's 100% of the time what's happening in real life. That I don't know. Okay, so receptor pharmacology. So if you look at these curves up here. And I wish that they wish that would have moved this x-axis up. But it's basically the same thing. It's still a dose response curve. And so you can see a full agonist, full efficacy partial agonists here, antagonists is really kind of along the baseline, really along the x-axis. And then inverse agonist dips below. So we'll talk about all four of these. Agonists. Often bind to the receptor using those different types of bonds that we talked about. Including maybe an ionic bond. I think Ionic is going to be more so if you see ionic bonds, it might be more so in the formulation of the drug. Less so in how it binds to its receptor, but it's not impossible. But usually with an agonist, your bonding is reversible. And so if you had that full stable configuration with a receptor You know, it's really not going to be as easily pulled apart from that. So you tend to see weaker Forces or weaker bonds with your agonist and receptor. Rarely covalent bonding. And then your magnitude of effect is going to be that total number of receptors that are occupied. And… Actually, just another point there. If you think about if you think about anesthesia. And I mean, as far as a great kind of application of this. It's a spectrum. It's a spectrum from awake to totally unconscious and under general anesthesia. So if we occupy a few receptors. And um with maybe midazolam. Let's say we're occupying some of those GABA receptors and we induce an anti-anxiety state, anxiolysis. Then we occupy a few more receptors and maybe we make that patient somewhat sedated. And we occupy a few more receptors and now they are getting into hypnosis or a sleep state That's the spectrum. That we see with anesthesia and that's kind of how Same basic idea with a full agonist. So with agonists, we're going to activate the receptor. So a full agonist should get to that maximal response if you have the right concentration. So they have the capacity to cause full efficacy. Continuous administration of an agonist causes downregulation of receptors. So propofol and fentanyl are examples of agonists. Partial agonists or the mixed agonists, antagonists. Causes limited receptor activation so It does not matter how much partial agonist you have in a tissue You're never going to get a maximal response. It just cannot achieve what a full agonist can. So our efficacy is reduced. These drugs are usually not as potent as a pure agonist as well. So partial agonists are said to have a ceiling effect because they cannot get to 100%. They can block the effects of a full agonist And maybe even produce withdrawal symptoms if you think about the patient who is opioid dependent opioid dependent but given a partial agonist can potentially have withdrawal. So an issue with partial agonist For example, buprenorphine. If you have a patient that's taking buprenorphine, they do not stop their buprenorphine before they come into surgery. If you think about the partial agonist that's sitting on those opioid receptors. Having only a partial effect It may cover some baseline level of pain. It may cover a little discomfort. So let's say they were getting a colonoscopy, you know, not painful in general but they might be okay if they still have that buprenorphine kind of sitting in their system. If, for example, that patient comes in still taking buprenorphine And now we are really inducing a lot of surgical trauma like they were in a car accident, had a femur fracture that we now need to kind of like ream a nail through And that patient has all those receptors occupied with drug that only has a partial effect. Think of how much agonist you would have to kind of dump into them to try to push that partial agonist off the receptor So that you can give them a full you know, opioid effect. You would just really be flooding them with agonists with very little you know effect because you're you've got buprenorphine sitting there and having that partial effect. That's a problem. So when we come across issues like that, we really have to get into other mechanisms of addressing pain and stimulation Or we have to have that patient stop a partial Agnes. You know, you've introduced this higher level of stimulation But then you've got partial efficacy on the receptor. And having a harder time getting to full advocacy. So that can be a problem. This example here of the withdrawal, that's kind of like the opposite. Effect. Antagonists. Again, can form a bunch of different types of interactions. It's going to be reversible bonding. Usually an antagonist is going to have a very similar structure as the agonist because they can both bind the receptor Now, an antagonist is not going to activate that receptor. In any way it's blocking an agonist from activating a receptor. If it's a competitive block. Then you can send in enough agonists to compete with your antagonist and hopefully reverse the effects of the antagonist. And I shouldn't say reverse the effects of it, but reverse it sitting there blocking a desired effect. Non-competitive antagonism. If you increase the level of agonist It will not overcome the antagonist. It is sitting there you know tightly bound There really is no competition. It's usually considered irreversible. Like I mentioned with aspirin. Now, if we put the two together, how does that affect a dose response curve?