Geotechnical Engineering - A Historical Perspective PDF
Document Details
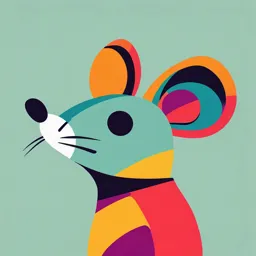
Uploaded by HappyBoolean4509
KSU-CEIT
Gine O. G.
Tags
Summary
This document provides a historical overview of geotechnical engineering, from the early developments in soil mechanics to modern approaches. It discusses core concepts like soil mechanics and soil engineering. The document is presented as lecture notes.
Full Transcript
GEOTECHNICAL ENGINEERING —A HISTORICAL PERSPECTIVE Prepared by: Gine O. G. (KSU-CEIT) SOIL is defined as the uncemented aggregate of mineral grains and decayed organic matter (solid particles) with liquid and gas in the empty spaces between the solid...
GEOTECHNICAL ENGINEERING —A HISTORICAL PERSPECTIVE Prepared by: Gine O. G. (KSU-CEIT) SOIL is defined as the uncemented aggregate of mineral grains and decayed organic matter (solid particles) with liquid and gas in the empty spaces between the solid particles. GEOTECHNICAL ENGINEERING is the subdiscipline of civil engineering that involves natural materials found close to the surface of the earth. It includes the application of the principles of soil mechanics and rock mechanics to the design of foundations, retaining structures, and earth structures. (Some Importance: Investigation of existing subsurface condition, determination of soil properties, assessment of risk posed by site conditions, designing earthworks and structure foundation) SOIL MECHANICS Branch of Civil engineering that concerns with the application or the principles of mechanics and hydraulics to the engineering problems related to soil. It deals with the study of the characteristics, classification and behavior of soil under loaded and unloaded condition. SOIL ENGINEERING is the application of the principles of soil mechanics to practical problems. Prepared by: Gine O. G. (KSU-CEIT) In true engineering terms, the understanding of HISTORY OF SOIL geotechnical engineering as it is known today began MECHANICS early in the 18th century (Skempton, 1985). KARL TERZAGHI’S contribution in the field of soil mechanics have been immense and he is fittingly called the “Father of Soil mechanics”. The soil mechanics was coined by him. (1883-1963) Four (4) Major Periods of Geotechnical Engineering (Skempton 1985) ✓Preclassical (1700 to 1776 A.D.) ✓Classical soil mechanics—Phase I (1776 to 1856 A.D.) ✓Classical soil mechanics—Phase II (1856 to 1910 A.D.) ✓Modern soil mechanics (1910 to 1927 A.D.) Prepared by: Gine O. G. (KSU-CEIT) This period concentrated on studies relating to natural slope and unit weights of various types of soils, as well as the Preclassical (1700 to 1776 A.D.) semiempirical earth pressure theories. Henri Gautier In 1717, a French royal engineer, studied the natural slopes of (1660–1737) soils when tipped in a heap for formulating the design procedures of retaining walls. In 1729, he proposed a theory for lateral earth pressure on Bernard Forest de Belidor retaining walls that was a follow-up to Gautier’s (1717) original (1671–1761) study. 1746, French engineer, observed the existence of slip planes in Francois Gadroy the soil at failure. later summarized by J. J. Mayniel in 1808 (1705–1759) Jean Rodolphe Perronet 1769, French engineer, studied slope stability and distinguished (1708–1794) between intact ground and fills. Prepared by: Gine O. G. (KSU-CEIT) During this period, most of the developments in the area of geotechnical engineering came from engineers and scientists Classical soil mechanics in France. In the preclassical period, practically all theoretical Phase I (1776 to 1856 A.D.) considerations used in calculating lateral earth pressure on retaining walls were based on an arbitrarily based failure surface in soil. Charles Augustin Used the principles of calculus for maxima and minima to determine the Coulomb (1736–1806) true position of the sliding surface in soil behind a retaining wall. He extended Coulomb’s theory by providing a graphical method for Jean Victor Poncelet determining the magnitude of lateral earth pressure on vertical and (1788–1867), inclined retaining walls. He was also the first to use the symbol Ф for soil friction angle. He also provided the first ultimate bearing-capacity theory for shallow foundations. Alexandre Collin In 1846, he provided the details for deep slips in clay slopes, cutting, and (1808–1890) embankments. Collin theorized that in all cases the failure takes place when the mobilized cohesion exceeds the existing cohesion of the soil William John Macquorn Rankine This study provided a notable theory on earth pressure and (1820–1872), equilibrium of earth masses. Rankine’s theory is a simplification of Coulomb’s theory. Prepared by: Gine O. G. (KSU-CEIT) Several experimental results from laboratory tests on Classical soil mechanics sand appeared in the literature in this phase. Phase II (1856 to 1910 A.D.) Henri Philibert Gaspard In 1856, he published a study on the permeability of sand filters. He defined Darcy (1803–1858) the term coefficient of permeability (or hydraulic conductivity) of soil. A professor of astronomy, conducted laboratory tests to determine the Sir George Howard overturning moment on a hinged wall retaining sand in loose and dense Darwin (1845–1912) states of compaction. Joseph Valentin In 1885, he develop the theory of stress distribution under loaded bearing Boussinesq (1842–1929) areas in a homogeneous semi-infinite, elastic, and isotropic medium. Osborne Reynolds In 1887, he demonstrated the phenomenon of dilatancy in sand. (1842–1912) Prepared by: Gine O. G. (KSU-CEIT) In this period, results of research conducted on clays were published in which the fundamental properties and parameters Modern Soil Mechanics of clay were established. (1910-1927) In 1911, a Swedish chemist and soil scientist, defined clay-size fractions as the percentage by weight of particles smaller than 2 microns in size. He realized the Albert Mauritz Atterberg important role of clay particles in a soil and the plasticity thereof. In 1911, he (1846–1916) explained the consistency of cohesive soils by defining liquid, plastic, and shrinkage limits. He also defined the plasticity index as the difference between liquid limit and plastic limit. Arthur Langley Bell He developed relationships for lateral pressure and resistance in clay as well as bearing capacity of shallow foundations in clay (see Bell, 1915). He also used shear- (1874–1956) box tests to measure the undrained shear strength of undisturbed clay specimens. An engineer from Sweden, developed the stability analysis of saturated clay slopes (that is, Ф= 0 condition) with the assumption that the critical surface of sliding is the arc of a Wolmar Fellenius circle. The paper published in 1926 gave correct numerical solutions for the stability (1876–1957), numbers of circular slip surfaces passing through the toe of the slope. 1919-1924 Developed the theory of consolidation for clays. Five different clay soils Karl Terzaghi were used. The liquid limit of those soils ranged between 36 and 67, and the plasticity (1883–1963) index was in the range of 18 to 38. Prepared by: Gine O. G. (KSU-CEIT) IMPORTANCE OF SOIL MECHANICS ❑ Application to foundation ❑ Application to Earth and Dams ❑ Embankments ❑ Canal and other retaining and under ground structures (retaining wall) Prepared by: Gine O. G. (KSU-CEIT) ORIGIN OF SOIL AND GRAIN SIZE Prepared by: Gine O. G. (KSU-CEIT) SOIL FORMATION AND TYPE OF SOIL The mineral grains that form the solid phase of a soil aggregate are the product of rock weathering. Weathering is the process of breaking down rocks by mechanical and chemical processes into smaller pieces. Mechanical weathering may be caused by the expansion and contraction of rocks from the continuous gain and loss of heat, which results in ultimate disintegration. Chemical weathering, the original rock minerals are transformed into new minerals by chemical reaction. Water and carbon dioxide from the atmosphere form carbonic acid, which reacts with the existing rock minerals to form new minerals and soluble salts Prepared by: Gine O. G. (KSU-CEIT) ROCK CYCLE Three (3) Basic Types of Rock (based on mode of origin) ✓ Igneous ✓ Sedimentary ✓ Metamorphic. Prepared by: Gine O. G. (KSU-CEIT) IGNEOUS ROCK Igneous rocks are formed by the solidification of molten magma ejected from deep within the earth’s mantle. The types of igneous rock formed by the cooling of magma depend on factors such as the composition of the magma and the rate of cooling associated with it. SEDIMENTARY ROCK The deposits of gravel, sand, silt, and clay formed by weathering may become compacted by overburden pressure and cemented by agents like iron oxide, calcite, dolomite, and quartz. Cementing agents are generally carried in solution by groundwater. They fill the spaces between particles and form sedimentary rock. Rocks formed in this way are called detrital sedimentary rocks. Sedimentary rock also can be formed by chemical processes. Rocks of this type are classified as chemical sedimentary rock. Sedimentary rock may undergo weathering to form sediments or may be subjected to the process of metamorphism to become metamorphic rock. METAMORPHIC ROCK Metamorphism is the process of changing the composition and texture of rocks (without melting) by heat and pressure. During metamorphism, new minerals are formed, and mineral grains are sheared to give a foliated texture to metamorphic rock. Gneiss is a metamorphic rock derived from high- grade regional metamorphism of igneous rocks, such as granite, gabbro, and diorite. Low-grade metamorphism of shales and mudstones results in slate. Prepared by: Gine O. G. (KSU-CEIT) TRASPORTATION OF WEATHERING The products of weathering may stay in the same place or may be moved to other places by ice, water, wind, and gravity. RESIDUAL SOILS: The soils formed by the weathered products at their place of origin. An important characteristic of residual soil is the gradation of particle size. Fine-grained soil is found at the surface, and the grain size increases with depth. At greater depths, angular rock fragments may also be found. TRANSPORTED SOIL: The soils formed by the weathered products moved to other places by ice, water, wind and gravity. Classified into several groups, depending on their mode of transportation and deposition: 1. Glacial soils—formed by transportation and deposition of glaciers. 2. Alluvial soils—transported by running water and deposited along streams. 3. Lacustrine soils—formed by deposition in quiet lakes. 4. Marine soils—formed by deposition in the seas. 5. Aeolian soils—transported and deposited by wind. 6. Colluvial soils—formed by movement of soil from its original place by gravity, such as during landslide. Prepared by: Gine O. G. (KSU-CEIT) PHASES OF SOIL (Soil and Voids) SOIL COMPOSITION SOIL DIAGRAM AIR The mineral grains that form WATER the solid phase of a soil aggregate are the product of SOIL rock weathering. AIR WATER WATER LOOSE SOIL SOIL SOIL SOIL PARTIALLY FULLY DRY SOIL SATURATED SOIL SATURATED SOIL Prepared by: Gine O. G. (KSU-CEIT) DENSE SOIL PARTICLE SIZE SOILS GENERALLY CLASSIFIED AS GRAVEL, SAND, SILT AND CLAY GRAVEL SAND SILT CLAY Prepared by: Gine O. G. (KSU-CEIT) PARTICLE SIZE ANALYSIS: CORSED GRAINED SOILS ❑ GRAVELS (Grain size > 4.75 mm) ❑ SAND (gran size < 4.75 mm) Prepared by: Gine O. G. (KSU-CEIT) PARTICLE SHAPE OF SOIL BULKY GRAINS - Formed mostly by mechanical TYPES OF SOIL GRAINS weathering of rocks and minerals. ❑ Small sand particles located close to their origin 1 BULKY GRAINS are generally very angular. ❑ Sand particles carried by wind and water for a long distance can be subangular to rounded in shape 2 FLAKY GRAINS The shape of granular particles in a soil mass has a great influence on the physical 3 ELONGATED GRAINS properties of the soil, such as maximum and minimum void ratios, shear strength parameters, compressibility etc. FLAKY PARTICLES – Have very low sphericity, usually 0.01 or less (Predominantly clay NEEDLE SHAPED/ELONGATED PARTICLES– minerals) Much less common than the two other particle types. Ex. Coral deposits Prepared by: Gine O. G. (KSU-CEIT) MECHANICAL ANALYSIS It is the determination of the size range of particles present in a soil, expressed as a percentage of the total dry weight. Two methods generally used to find the particle-size distribution of soil: ❑ sieve analysis - for particle sizes larger than 0.075 mm in diameter ❑ hydrometer analysis - for particle sizes smaller than 0.075 mm in diameter. Prepared by: Gine O. G. (KSU-CEIT) SIEVE ANALYSIS Sieve analysis is a fundamental technique in civil engineering and soil mechanics used to determine the particle size distribution of granular materials, such as soil, sand, gravel, or other aggregates. Sieve analysis, also known as gradation test, involves passing a material sample through a series of sieves with progressively smaller mesh sizes to separate particles of different sizes. The percentage of material retained on each sieve is recorded, providing a detailed breakdown of the particle size distribution. Sieve analysis consists of shaking the soil sample through a set of sieves that have progressively smaller openings. U.S. standard sieve numbers and the sizes of openings are given in Table 2.5. Prepared by: Gine O. G. (KSU-CEIT) SIEVE ANALYSIS PROCEDURE 2 Prepare the stack of sieves 1 (smaller opening at the Collect a representative soil bottom) and record the mass sample and oven dry after which of each sieve. break all lumps into smaller particles. Then weigh approximately 500 g 4 After the sample is Pour the soil sample in the stack of shaken, record mass of sieves cover then run the sieves soil retained on each through a mechanical shaker sieve. 3 5 Calculate mass of soil retained on each sieve. Plot particle distribution curve (logarithmic graph) Prepared by: Gine O. G. (KSU-CEIT) SIEVE ANALYSIS: CALCULATION ❑ Calculate the mass of sample retained ❑ Calculate the cumulative percent of mass retained on each sieve 𝐖𝐧 = 𝐌𝐚𝐬𝐬 𝐨𝐟 𝐬𝐢𝐞𝐯𝐞 + 𝐬𝐨𝐢𝐥 𝐫𝐞𝐭𝐚𝐢𝐧𝐞𝐝 − 𝐦𝐚𝐬𝐬 𝐨𝐟 𝐬𝐢𝐞𝐯𝐞 𝐢=𝐧 ❑ Calculate the percent of mass retained on each sieve Ʃ𝐑𝐧 = 𝐑𝐧 𝐢=𝟏 𝐦𝐚𝐬𝐬 𝐫𝐞𝐭𝐚𝐢𝐧𝐞𝐝, 𝐖𝐧 𝐑𝐧 = ∗ 𝟏𝟎𝟎 𝐭𝐨𝐭𝐚𝐥 𝐦𝐚𝐬𝐬 𝐨𝐟 𝐬𝐨𝐢𝐥 𝐬𝐚𝐦𝐩𝐥𝐞, 𝐖𝐓 ❑ Calculate the percent passing on each sieve WT = Total summation of mass of sample retained % 𝐩𝐚𝐬𝐬𝐢𝐧 𝐠Τ𝐟 𝐢𝐧𝐞𝐫 = 𝟏𝟎𝟎 − Ʃ𝐑𝐧 ❑ Plot the particle size distribution curve. ❑ Calculate the percentage of mass loss during sieve analysis, should be less than 2%. 𝟓𝟎𝟎 − 𝐖𝐓 % 𝐥𝐨𝐬𝐬 = 𝟏𝟎𝟎 𝟓𝟎𝟎 SIEVE ANALYSIS: GRAPH ❑ Determine D10, D30 and D60 from the grain size distribution curve, which respectively the diameters are corresponding to percent finer PARTICLE SIZE DISTRIBUTION CURVE of 10%, 30%, and 60%. 100 ❑ Determining the uniformity coefficient 90 (Cu) and the coefficient of 80 gradation (Cc) 𝐃₆₀ 70 𝐂𝐮 = 𝐃₁₀ PERCENT FINER (%) 60 𝐃2 𝟑₀ 𝐂𝐜 = 𝐃₆₀ ∗ 𝐃₁₀ 50 ❑ Sorting coefficient (S0): 40 This parameter is another measure of uniformity and is 30 generally encountered in geologic works and expressed as 20 𝐃𝟕𝟓 10 𝐒𝐨 = 𝐃𝟐𝟓 0 10 1 0.1 0.01 PARTICLE SIZE DIAMETER (mm) Prepared by: Gine O. G. (KSU-CEIT) GRADATION TYPE OF SOIL The percentages of gravel, sand, silt, and clay-size particles present in a soil can be Different types of particle-size distribution curves obtained from the particle-size distribution curve. Poorly Graded Soil - a type of soil in which most of the soil grains are the same size. Well Graded Soil - a soil in which the particle sizes are distributed over a wide range. A well- graded soil has a uniformity coefficient greater than about 4 for gravels and 6 for sands, and a coefficient of gradation between 1 and 3 (for gravels and sands). Gap Graded Soil - a type of soil in which most of the soil grains are of the larger particle sizes or smaller particle sizes. Prepared by: Gine O. G. (KSU-CEIT) Following are the results of s sieve analysis. Make the necessary calculations and draw a particle-size distribution curve. Mass of soil Cumulative mass U.S. sieve no. Opening (mm) retained on each retained above % Finer sieve (g) each sieve 4 4.75 0 100 10 2 40 94.51 20 0.850 60 86.28 40 0.425 89 74.07 60 0.250 140 54.87 80 0.180 122 38.13 100 0.150 210 9.33 200 0.075 56 1.65 Pan - 12 0 SAMPLE PROBLEM SOLVING: Prepared by: Gine O. G. (KSU-CEIT) 01 PARTICLE SIZE DISTRIBUTION CURVE 100 90 80 70 PERCENT FINER (%) 60 50 40 30 20 10 0 10 1 0.1 0.01 PARTICLE SIZE DIAMETER (mm) From the particle-size distribution curve at problem 01, determine the following: a) D10, D30 and D60 b) Uniformity coefficient, Cu c) Coefficient of gradation, Cc From the particle-size distribution curve at problem 01, determine the percentages of gravel, sand, silt and clay size particles present. Use USCS with the following description: Gravel: % retained at No. 4 U.S. sieve (4.75mm opening) Sand: % passed at No. 4 and retained at No. 200 U.S. sieve Silt and Clay: % passed at No. 200 U.S. sieve SAMPLE PROBLEM SOLVING: Prepared by: Gine O. G. (KSU-CEIT) 02