Lecture 1-31-79 PDF
Document Details
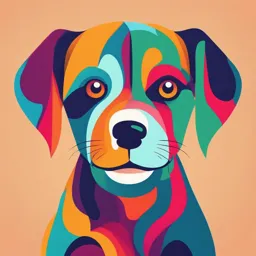
Uploaded by ExpansiveHarpy2650
Khalifa University of Science and Technology
Tags
Summary
This document provides information on various types of energy production, including different power plants such as Rankine cycle, fossil fuel, and solar power plants. It also covers nuclear fission and its relevance to energy production.
Full Transcript
Rankine Cycle Process (2) 3. Vapor (dry) expands through turbine Turbine spins – generates electricity 4. Vapor (wet) enters condenser Cycle restarts @1...
Rankine Cycle Process (2) 3. Vapor (dry) expands through turbine Turbine spins – generates electricity 4. Vapor (wet) enters condenser Cycle restarts @1 Flow 31 Thermodynamic Efficiency η = Thermodynamic Efficiency = Net Work Out Heat Added THOT − TCOLD “Carnot Cycle” -- ηc = THOT Absolute Temperature: T (Absolute) = T (Centigrade) + 273⁰ Carnot cycle is ideal Others (e.g., Rankine) are less efficient. Example: Modern Coal-fired Power Plant THOT − TCOLD (350 + 273) − (50 + 273) ηc = = = 48% THOT 350 +273 32 Fossil Fuel Steam Power Plant Water is converted into steam Chemical Energy → Thermal Energy → Kinetic Energy → Mechanical Energy → Source: "Nuclear Reactor Concepts" Workshop Manual, U.S. NRC http://www.nrc.gov/reading-rm/basic-ref/teachers/unit3.html Electrical Energy 33 Concentrating Solar Power Plant 1. Sunlight is concentrated and directed from a large field of heliostats (mirrors) to a receiver on a tall tower. 2. Molten salt from a cold salt tank is pumped through the receiver, where it is heated to 1050°F (566°C). 3. The heated salt from the receiver is stored in a hot salt tank. 4. Molten salt is pumped from the hot salt tank through a steam drum that creates steam to drive a steam turbine and generate electricity. 5. Cold salt (525°F/288°C) flows back to the cold salt tank to be re-used. Source: US DOE/EERE (www.eere.energy.gov) Solar Energy → Thermal Energy → Kinetic Energy → Mechanical Energy → Electrical Energy Source: Sandia National Labs 34 Nuclear Fueled Steam Plant Nuclear Energy → Kinetic Energy → Thermal Energy → Kinetic Energy → Mechanical Energy → Electrical Energy Fossil Fueled Steam Plant Nuclear Fueled Steam Plant Source: "Nuclear Reactor Concepts" Workshop Manual, U.S. NRC http://www.nrc.gov/reading-rm/basic-ref/teachers/unit3.html 35 Nuclear Fission Energy (E = mc2) Fission Demonstration Uranium in nature Fissioning 1 kg. U-235 Equivalent to Burning 99.3 percent U-238 3,000 tons Coal 0.72 percent U-235 36 Where the Thermal Energy (Heat) Comes From UO2 fuel pellet. Enriched to 3-5 percent U-235 37 Electricity Production Global Electricity Production (2012) Solar 0.4% Wind Geo- 2.4% thermal 0.3% Biomass 2% Fossil Fuels 67% Renewables 22% Hydro 17% Nuclear 11% Source: US Energy Information Agency (http://www.eia.gov/) 38 World Nuclear Energy Production 2011 3000 Fukushima Accident Electricity Generation Worldwide (TW-h) 2500 2000 1500 1000 500 0 39 Current Status of World Nuclear Power 41 reactors in LTO Future Plans POWER REACTORS NUCLEAR 69 UNDER CONSTRUCTION NUCLEAR POWER REACTORS 96 PLANNED 380 GWe installed capacity 83.5 GWe NEW CAPACITY PLANNED LTO = long-term outage (>4 years) Source: https://www.iaea.org/pris/ 40 Greenhouse Gas Emissions Life Cycle GHG Emissions Nuclear 41 Energy Demand & CO2 Emissions Gtoe = gigatonnes of oil equivalent 42 Safety, Safeguards, and Security Nuclear Power is unique among energy sources Nuclear Fuel o Specialized technology and design knowledge Decay Heat o Reactors continue to generate thermal energy after shut down Nuclear Waste o Radioactive and hazardous materials Dual Use o Nuclear technology can be used to develop materials for nuclear weapons Special requirements for Safety, Security, and Safeguards (3S) 43 Images from US NRC (http://www.flickr.com/photos/nrcgov/) 44 NSSS – Reactor Vessel Internals The fuel rods are grouped together APR1400 Upper Guide in a regular array Structure Assembly or fuel assembly The ceramic fuel The fuel assemblies pellets are stacked are arranged in a in a column and larger regular array sealed inside a or reactor core metallic alloy case, called the cladding, to form a fuel rod 45 Types of Nuclear Power Reactors Power Reactors are classified by – Neutron energy Thermal reactors : “thermal” neutrons (energy < 1 eV) Fast reactors: “fast” neutrons (energy > 1 MeV) – Moderator – Coolant – Fuel & Enrichment Main Types of Nuclear Power Reactors – Light Water Reactors (LWRs) Pressurized Water Reactor (PWR) Boiling Water Reactor (BWR) – Gas-cooled Reactors (GCRs) Thermal or Fast – Heavy Water Reactors Canadian Deuterium-Uranium Reactor (CANDU) 46 Boiling Water Reactor (BWR) Reactor Coolant drives the Turbine – Water boils in the core; steam drives turbine; condenses & returns to core – Simple design 47 Pressurized Water Reactor (PWR) Reactor Coolant heats Steam Generator – Two circulatory systems (loops) – Water boils in Steam Generator; steam drives turbine; condenses & returns to Steam Generator Secondary loop Primary loop Source: NRC http://www.nrc.gov/reading-rm/basic-ref/students/animated-pwr.html 48 Canadian Deuterium-Uranium Reactors Two Loops Source: www.Nuclearfaq.ca “Heavy” Water 49 Gas-Cooled Reactor -- Magnox CO2 Two Loops © Österreichisches Ökologie-Institut http://www.ecology.at/nni/index.php?p=type&t=gcr 50 Nuclear power plants in commercial operation ** ** 270 248 84 78 47 23 17 8.8 15 10 2 0.6 GWe = capacity gigawatts (millions of kilowatts) (** These two columns are from Jan 2012 IAEA data) (Sources: Nuclear Engineering International Handbook 2008 and http://www.world-nuclear.org/info/inf32.html) 51 Pressurized Water Reactor Primary loop – Reactor coolant (hot) ➔ steam generator – Reactor coolant (cold) ➔ reactor Secondary loop – Steam Generator cooling water Water vaporizes (dry steam) & heat sink – Steam (dry) from steam generator ➔ turbine Turbine generates electricity Primary Loop – Steam (wet) from turbine ➔ condenser Secondary Loop Heat removed and steam condenses (to water) Cold-water supply & heat sink (lake, stream, cooling tower) – Water (cold) ➔ steam generator Primary & Secondary Loops do not mix APR-1400 is a PWR 52 Reactor Power System Layout 53 APR1400 Nuclear Steam Supply System in Containment 54 NSSS – Reactor Vessel 4 Inlet nozzles, 2 Outlet nozzles and 4 Direct Vessel Injection (DVI ) nozzles. Provides barrier to fission product release Part of coolant system pressure boundary 55 NSSS – Reactor Coolant System Loop configuration 1 Reactor vessel 1 Pressurizer 2 Steam generators 4 Recirculating Coolant Pumps 2 Hot legs, 4 Cold legs 56 NSSS – Steam Generator (SG) SG tube design No. of tubes per SG : 13,102 Plugging margin : 10 % Material : Inconel 690 Improved upper tube support bar and plate To reduce flow-induced vibration Modified primary outlet nozzle angle To improve stability of mid-loop operation 57 NSSS – Pressurizer Design values Total Free volume : 2,400 ft3 (67.9 m3) 4 POSRV Coolant volume at full power : 1,100 ft3 (31.1 m3) Nozzles Heater capacity : 2,400 kW Increased pressurizer volume Enhance capability against RCS transients Pilot Operated Safety Relief Valve (POSRV) 4 POSRVs Function both over-pressure protection and safety depressurization Reliable valve operation without chattering and leakage Low valve stuck-open susceptibility 58 NSSS – Reactor Coolant Pump (RCP) Type Vertical bottom suction Horizontal discharge Single stage impeller Motor-driven centrifugal pump Speed : 1,190 rpm Shaft seal assembly Two face-type mechanical seals Reduce the RCS pressure to volume control tank pressure Third face-type low-pressure vapor seal Withstand RCS pressure when RCP is stopped 59 Turbine & Generator Turbine Number : 1 double flow HP TBN, 3 double flow LP TBN Type : Tandem-Compound Turbine Speed : 1,800 rpm Output : 1,455 MWe Last Stage Blade : 52 inch LSB Generator Number : 1 Type : Direct Driven (conductor cooled) Voltage : 24 kV, 3Phase Frequency : 60 Hz 60 What are the costs? Power Plant Siting, Licensing & Construction – Siting & Licensing Fees, applications, legal challenges – Construction costs Land, materials, labor, insurance Interest on money borrowed Fuel These costs can vary – Production/mining dramatically across – Fabrication/processing technologies – Transportation & storage – Waste management Plant Operations & Maintenance – Labor, plant maintenance, insurance, upgrades Plant Decommissioning 61 Construction Costs © WM Symposia, Inc. All Rights Reserved. 62 Fuel Costs © WM Symposia, Inc. All Rights Reserved. 63 Operations and Maintenance Costs © WM Symposia, Inc. All Rights Reserved. 64 Land Use & Cost of CO2 Emissions (if taxed) © WM Symposia, Inc. All Rights Reserved. 65 What are some incentives? Government Support* Industry Options – Tax incentives & subsidies – Consortia Credits, exemptions, deductions Shared cost & risk Penalties – Alternative options – Emissions & environmental (e.g., Turnkey (fixed price) CO2 “tax”; emissions requirements) Build, Own, Operate (BOO) CO2 “Cap & Trade” Technology transfer – Loan guarantees Fuel leasing & take-back Reduce risk to lenders Public Opinion – Regulatory Licensing, Environmental, – Demand for low-emission International technologies – Liability Limits/Protection – Willingness (and ability) to pay – Infrastructure – Health & Wellbeing Development & Maintenance Improved standard of living – Education & Training Programs Reduced mortality Incentives vary dramatically across technologies and among countries * Many government-support programs are considered “indirect” costs, as they are not born by power company shareholders or investors, but by taxpayers and the public. Such programs may, however, factor into risk analyses and perceptions. 66 Economic Considerations How to compare the costs of different technologies over extended production periods? Levelized Cost of Electricity (LCOE) o LCOE compares unit* costs of different technologies over their economic lives o Primary variable for this study How to calculate LCOE? *Comparison is not for units of equal generating capacity 67 Economic Considerations For each technology to be evaluated, estimate the LCOE: t=1,n{[It + O&Mt + Ft + Ct + Dt]×(1+r)-t} LCOE = t=1,n{Et×(1+r)-t} Et: Cost of producing Electricity in year “t” (USD); r: Annual discount rate in year “t” (unitless) It: Investment costs in year “t” (USD); O&Mt: Operations & Maintenance costs in year “t” (USD); Ft : Fuel costs in year “t” (USD); Ct: Carbon costs in year “t” (USD); D t: Decommissioning cost in year “t” (USD) t summations are over all years (t1, t2, t3, …, tn) Many input values are uncertain and vary over time and by region 68 Discount Rate (r) The interest rate used to determine the present value of future cash flows – Expected return on investment Discount rate accounts for: 1. Time-value of money 2. Risk or uncertainty of future cash flows Current Value = (Future Value at time t) (1+r)t The greater the uncertainty of future cash flow, the greater the discount rate A high discount rate implies a high (perceived) investment risk r and t must be compatible; that is, if r is the rate of compounding over some time interval (e.g., one year), t is the compound interval (e.g., years). 69 Effect of Risk on Capital Costs: Intensity Capital Intensity reflects vulnerability to changes in output price and/or demand Lower risk - - - - - - - - - - - - - - Higher risk Large nuclear power plants tend to be more cost efficient over long term 70 Cost & Capacity Comparison CCGT: Combined-cycle gas turbine; SC/US: Supercritical & Ultrasupercritical Coal Technologies; CC(S): Carbon Capture & Storage; PV: Photovoltaic. Note-1: Costs estimates reflect 2010 data and are subject to change. Note-2: Costs cannot be known to the precision indicated! Realistic uncertainties might be 10% or more. Note-3: Current (2014) cost estimates for new construction are on the order of $7 to $8 billion US dollars for a 1200 MWe reactor (e.g., Vogtle units 3 & 4 in the US), and Finland’s new 1600 MW(e) Olkiluoto unit 3 is currently expected to cost nearly 11 billion US dollars and come on line nearly six years late. Construction delays are common and expensive. Note-4: Carbon Capture & Storage technologies are still under development and subject to considerable cost uncertainties. 71 Cost Structure 100% 5% discount rate (r) As a percentage of total costs: 75% Capital investment is the Investment major contributor to life-time cost (LCOE) 50% O&M 25% Fuel – except for gas-powered plants CO2 Decommissioning Fuel cost is largest contributor 0% to LCOE for gas-powered plants – Based on 2010 gas-price data – Natural gas prices are highly 100% variable 10% discount rate (r) 75% Investment 50% O&M Fuel 25% CO2 Decommissioning 0% 72 Main Risk Factors for Investment in Power Generation Plant Risk Market Risk Regulatory Risk Policy Risk Environmental Construction Costs Fuel Costs Market Design Standards Regulation of Lead Time Consumer Demand CO2 Constraints Competition Operational Costs Competition Transmission Technology Support Availability & Licensing & Price of Electricity Energy Efficiency Performance Approval Many risks are outside the scope of the LCOE methodology 73 Main Risk Factors for Investment in Power Generation Capital Cost Operating CO2 Regulatory Technology Unit Size Lead Time Fuel Cost (per kW) Cost Emissions Risk Nuclear V. Large Long High Medium Low Nil High Coal Large Long High Low Medium High High CCGT Medium Short Low Low High* Medium Low (gas) Wind Small Short High Medium Nil Nil Medium Hydro V. Large Long V. High V. Low Nil Nil High * Based on 2010 costs 74 Cost Summary Total Global Costs for Producing 266 tkWhrs from Existing Fleet -- 2010 to 2060.* Total Global Costs for Producing 994 tkWhrs from New Builds -- 2010 to 2060. 75 Conclusions No single electrical-generation technology is a least-expensive option for all situations Nuclear power is a cost-competitive source of electricity – Economy of scale Tends to favor large-capacity power plants (E.g., nuclear) But – require considerable up-front investment – Large financial resources of national governments and large corporations – Most favorable cost advantage compared to renewables or when accounting for carbon capture But – No carbon-capture technology currently in use 76 References U.S. NRC "Nuclear Reactor Concepts" Workshop Manual, http://www.nrc.gov/reading-rm/basic-ref/teachers/unit3.html – Nuclear Power for Energy Generation http://www.nrc.gov/reading-rm/basic-ref/teachers/01.pdf – The Fission Process and Heat Production http://www.nrc.gov/reading-rm/basic-ref/teachers/02.pdf – Pressurized Water Reactor Systems http://www.nrc.gov/reading-rm/basic-ref/teachers/04.pdf DOE Fundamentals Handbook “Nuclear Physics and Reactor Theory”, http://www.hss.doe.gov/nuclearsafety/ns/techstds/standard/ hdbk1019/h1019v2.pdf 77 References DOE/NE-0088, “History of Nuclear Energy” http://www.uic.com.au/nip50.htm United Nations http://disarmament.un.org/TreatyStatus.nsf U.S. State Dept http://www.state.gov/t/ac/trt/ Acronym Institute http://www.acronym.org.uk/npt/ http://www.iaea.org, especially: 1. http://www.iaea.org/NewsCenter/Focus/Npt/ 2. NPT = IAEA Information Circular (“INFCIRC”) 140 3. Model Comprehensive Safeguards Agreement = INFCIRC/153 4. Model Additional Protocol = INFCIRC/540 78 References U.S. NRC "Nuclear Reactor Concepts" Workshop Manual, http://www.nrc.gov/reading-rm/basic-ref/teachers/unit3.html – Nuclear Power for Energy Generation http://www.nrc.gov/reading-rm/basic-ref/teachers/01.pdf – The Fission Process and Heat Production http://www.nrc.gov/reading-rm/basic-ref/teachers/02.pdf – Pressurized Water Reactor Systems http://www.nrc.gov/reading-rm/basic-ref/teachers/04.pdf DOE Fundamentals Handbook “Nuclear Physics and Reactor Theory”, http://www.hss.doe.gov/nuclearsafety/ns/techstds/standard/ hdbk1019/h1019v2.pdf Lamarsh, J. R., and Baratta, A. J., “Introduction to Nuclear Engineering”, third edition, Prentice Hall, 2001 Stacey, W. M., “Nuclear Reactor Physics”, second edition, Wiley-VCH, 2007 79