Microelectronics: Devices to Circuits Lecture 07 PDF
Document Details
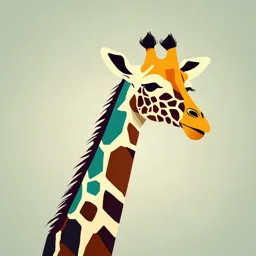
Uploaded by PeerlessBugle
Indian Institute of Technology Roorkee
Sudeb Dasgupta
Tags
Summary
This document is lecture notes on microelectronics, covering the topic of BJT as an amplifier, focusing on small circuit models. The lecture explains various configurations and concepts related to BJT amplifier design.
Full Transcript
Microelectronics: Devices to Circuits Professor Sudeb Dasgupta Department of Electronics and Communication Engineering Indian Institute of Technology Roorkee Lecture−07 BJT...
Microelectronics: Devices to Circuits Professor Sudeb Dasgupta Department of Electronics and Communication Engineering Indian Institute of Technology Roorkee Lecture−07 BJT as an Amplifier, Small Circuit Model-II Hello everybody and welcome to the online NPTEL online certification course on microelectronics: Devices and Circuits. In our previous discussion on our lecture and the previous module we have understood how your BJT acts as a switch as well as an amplifier and where should we bias our device, so that the device works as an amplifier. In this slide we are continuing with the same thing which we have left in the previous one. (Refer Slide Time: 1:05) That we see that in a common emitter mode configuration, if you have a common emitter mode configuration BJT, right? Then you have RC here, right? You have VCC and then you will have, this so for a common emitter mode configuration you will have this and then there will be a bias given here and this bias will bias the device at a particular, appropriate Q-point, let’s see how do, I do that. Assuming, so if you go back to this table this is basically your VCE, so I get, 𝑉𝐶𝐶 = 𝐼𝐶 𝑅𝐶 + 𝑉𝐶𝐸 , right? So you see, 𝑉𝐶𝐶 = 𝐼𝐶 𝑅𝐶 + 𝑉𝐶𝐸 ,, therefore if you want to make 𝐼𝐶 𝑅𝐶 one side I get 𝐼𝐶 𝑅𝐶 = 𝑉𝐶𝐶 − 𝑉𝐶𝐸 , so as I discussed with you IC will be there for equals to VCC by RC minus VCE by RC. So now if you plot a graph of, let us say on this side you have got IC, right? And on this side you have got VCE, right? Now putting the same equation in this one if you look at the x- axis your IC will be equals to zero in that case. So when IC equals to zero, I get VCE or VCE to be equals to VCC so what I can point out is, that this is the VCC point, right? And then on the y-axis your VCE will be equal to zero. So, at x- axis, right? IC equals to zero, place it into this equation and you get VCE equals to VCC this is the first thing you get and you place a point here. The second point is at y-axis, I get VCE to be equal to zero. As I get VCE equal to be zero, I safely write down this to be as IC to be equals to VCC by RC, so I put a point here which is basically VCC by RC. Now simply join these two lines and we define this to be as the load line. I will explain to you why it is known as load line. So it’s a load line, whose slope is incidentally equals to -1 by R, fine, which means that if the resistance 1 by RC actually this RC is collector resistance. So depending upon the collector resistance the slope of this line will change, right? Now wherever it cuts the I-V characteristics of the device, we define that to be as the Q-point those are the possible points where you will get the Q-point. So if you have I vs V characteristics here that’s a good idea to plot it first of all like this and I get something like this then, I get this to be possibly a Q-point because within the active region this Q-point seems to be further away from saturation as well as from cut-off. So this is my cut-off point, this is my active and this is saturation. So how did you find cut- off? Cut-off is when your IB is zero. For any point in which IB is less than zero, it is cut-off. So this is your cut-off region. So your device is cut-off there is no idea, this one is saturation we have already discussed yesterday and this is the active region, so you should bias your device using a DC bias, external DC bias such that the Q-point is somewhere lying here. So the Q-point is this much. Corresponding to this Q-point you will have VCE in this direction and IC in this direction, so this VCE and so we write VCEQ and ICQ. So ICQ is the quiescent collector current and we define this to be as the collector to emitter quiescent voltage, right? And once you have found out, now what you do? With this value of a voltage and current, if you give in the input side using a DC bias I superimpose my AC signal over this DC bias, right? (Refer Slide Time: 5:41) And that is what they’re trying to do over the period of time that we are trying to find out our DC signal, so as you can see from here the Q-point is somewhere, well so you see this is your saturation region, this is your active region by the definition we are handling and below this you have got cut-off, right? And this is the Q-point which you see for various values of IB, right? It is actually VBE because IB will depend upon also the value of VBE. Now IC is by this and therefore the slope is equals to minus 1 by RC and therefore this is known as load line but you go on changing the load here, right? You go on changing the load here and you automatically get… So if the RC value is now smaller the slope will change drastically, right? The slope will be something like this now. It might be something like this, right? So if you make your VCC larger and larger the load line will move to the right. If you make it smaller and smaller it will move to the left. So it will move like this, if VCC is made high, right? And it will move to this side if VCC is made lower, right? And depending on the value of RC the slope of the load line will go on changing, fine. So we have therefore found out a graphical method by which we can predict what should be the Q-point or the bias point where my device should be sitting, so that in the active region so that a proper amplification is available to me, right? That is very-very important here I wanted to tell you. Ahh.. This is the, therefore this is the graphical method of determining the VTC the voltage transfer characteristics. And this gives me a quite a nice idea about or a decent idea about where should the bias point or the Q-point should be kept in general. (Refer Slide Time: 7:32) With the same concept we have learned till now, let me show you a proper amplification here. Now as I discussed with you that this is the common emitter mode configuration transistor which you see, this is my NPN transistor. VCE is this voltage is VCE, this is VBE which you see, right? And this is your VCE, right? Now if you, as I discussed with you for low values of VBE typically your, the device will be cut-off because base to emitted junction will not be forward biased for silicon it is 0.7. So anything below 0.7 cut-off, cut-off means this is zero. Zero means all VCC appears across the output voltage and this is what you see here. So this part is that discussion which you will see. Similarly if your input voltage VBE is very large as compared to the cut-in voltage of the base emitter junction which is 0.7 for silicon, I would expect to see the transistor to get on, so I will get from off-state, so this is cut-off state and I will get on-state, right? This is the on-state, right? And I get saturation here, right? I get saturation. So saturation is there and the output voltage will certainly fall off. Why it will fall off because if you remember, 𝑉𝐶𝐶 = 𝐼𝐶 𝑅𝐶 + 𝑉𝐶𝐸 , right? So if you take it this side and do sort of try to find out the value of 𝑉𝐶𝐸 = 𝑉𝐶𝐶 − 𝐼𝐶 𝑅𝐶. So when your IC is zero, you get VCE equals to VCC and that’s what you get here. When you have large values of IC this quantity becomes large and what you get is VCESat or the saturating value of VCE which is approximately 0.1 to 0.2 volts typical value, so this value is approximately equals to 0.1 to 0.2 in saturation, fine? Now you see if you bias it here point ‘A’ let us suppose or you bias it here let me say here, right? Point ‘A’ and point ‘B’ if you bias it obviously point ‘A’ and point ‘B’ will give you a DC bias, external DC output voltage. Point ‘A’ will give you output 1 and point ‘B’ will give you output 0 as you can see from the definition of output here but let’s concentrate on the part where this active region is there or active mode is there, right active mode? In the active mode if you see the voltage is drastically falling VCE with the small change in the value of VBE. So this is basically VCE vs VBE, so this is the output voltage and this is the input voltage. So this is my input voltage, right? And this is my output voltage so you see, so I define my voltage gain AV to be equals to 𝜕Vout to 𝜕Vin, right? This we have already discussed, so if you look at point ‘A’ my 𝜕Vout is 0, so my AV is zero because there is no change in the output voltage is constant, independent of the input voltage. Similarly at point ‘B’ again my output is constant, independent of input voltage. So in both the cases my overall gain (AV) is zero, voltage gain is zero but the voltage gain in the active mode which is this one is pretty high because you see a very small change in input gives you a large change in the output, right? But the change is what? Negative change. So I get a negative gain sort of concept which means that for an increase in value of input, I get a decrease in the value of output, right? And the decrease is not equal but it is a very large decrease, so you get a large voltage gain. So higher the slope of this curve from ‘Y’ to ‘Z’ through ‘Q’, more will be the gain or more will be the slope and more will be the gain of the transistor, right? So if you want to operate the device as an active device then please operate within this region which is dotted here and if you don’t want to operate and let it work as a digital world you operate in these two positions. So when you use TTL logic, you use this right transistor-transistor logic. When you want to use it as an amplifier you use this, right? So we have made one thing very clear here that for sure therefore, that if you have a BJT working in the active mode of operation and I am biasing my device in the active mode I am surely getting a large level of amplification to me, right? And is amplification will be linear amplification, right? And I will also find, will show, I would like to convey to you why is it like that also. (Refer Slide Time: 12:12) The reason is something like this. The reason is that for equal change in the value of base current, I should get an equal change in the value of a collector current, that is what linearity means. That if I do similar changes in IB, I should expect to see a similar change in IC also, right? So you see if you change IB by this much amount, right? Such that the Q-point shifts from here to here from this point and then goes from here to here, right? But the change in IC is linear, so this distance is exactly equals to this distance, right? And therefore we safely say that the amplification is linear in nature, so the amplification which you find out amplification is primarily linear in dimension, sort of linear in nature, right? But the reason being that you are able to, because the rate of change is almost constant for both the cases, fine. And that makes our life easier as far as understanding the graphical analysis is concerned. So you see I gave an input, so my input will be given, so…so… let me therefore recapitulated, so what you do? (Refer Slide Time: 13:26) You first insert the DC source, right? You bias the device where, so that it is in the Q-point in the active region for amplification purposes, right? And then superimpose the AC signal, right? Which you want to amplify, right? So you superimpose the AC signal which you want to amplify. So what is the AC signal here? The blue one if you see here, this is my input AC signal and we are giving it to as VBE, so I am giving as VBE base to emitter voltage change, so I have a DC bias, say 2 volts and 2 volts DC bias is kept superimposed on that I have my AC signal, small signal AC signal, fine. One important point which I should point out here is that there is a limit of the input AC signal. You just cannot give any AC signal you want and it will work fine for the BJT for example, specially the peak-to-peak value, right? If the peak-to-peak value, suppose you are biasing Q- point here and your peak value is so large that it makes this Q-point go somewhere here in the non-linear region or even here or on the negative side get somewhere on the non-linear region or even here, then you enter into non-linear amplification domain. Although your amplification fall down, not only that it will depend upon the value of input not a good idea. So you see therefore, we always consider this to be as a small signal analysis, right? What is the meaning of small signal analysis? It means that, that your input signal is so small peak-to-peak that I can sufficiently state that the amplification is linear because if the input signals are too large then the Q-point will move so much away from its basic VCE value which is kept here, right? That it might even go up to this much point, here. Here if you see it is something like this, it is coming like this and then it fell like this and then it became linear and then it became like this, so if you are somewhere working here or here, it is basically non-linear and as a result you will get a non-linear amplification. So you need to bias the Q-point such that you are just restricting your peak-to-peak between this point and this point, right? So you should be very careful how you are biasing you device, depending on that will get the output. So depending on that you are getting the output here which is 180 degree phase shifted as well as amplified by a factor which is equal to value known to you. So what will be the small signal gain which you will get? Av will be equal to VCE, right? Divide by VBE typically that will be the voltage gain which you will see out of the signal, right? (Refer Slide Time: 16:31) So with this we have understood the basic amplification process of a BJT, so as I discussed with you earlier also, in the earlier module as well as in this module the most commonly used BJT amplifier is the common emitter amplifier, right? So common emitter amplifier or common emitter circuit is the most commonly used amplifier design. Now, my input signal as I discussed with you, will always be a sum of the DC bias plus the signal which you applied, right? So, Vin is the input signal will be a sum of the AC signal plus the DC bias. So DC bias will fix up the Q-point and AC signal will be a signal which will be given, which will shift the Q- point so that you are able to amplify the signal between input and output. Now the output range is VCE as I discussed with you, right? And it cannot go beyond a particular region. VCE should also be limited by certain things. Just as I should make it a point that VCE cannot be more than of course VCC I will give you an example. (Refer Slide Time: 17:36) Let us suppose you have an input signal which is 1 volt peak-to-peak, right? You have Vin which is 1 whole peak-to-peak your Av is 100, right? And your VCC is let us say, 20 volts for a BJT, right? So what will be your Vout peak-to-peak? It will be 100 multiplied by 1 which is equals to 100 volts, fine. So your output is 100 volts but your VCC is only 20 volts, so you see that in no way we can get 100 volts output. So what will happen is that it will go up to 20 volts, it will then clip back here, because this is +20 this is -20 whatever +20 and so on and so forth, because zero it will go to zero and not go further down, right? So you see therefore that the output of a BJT will depend not only on the gain, small signal gain of the amplifier but it will also depend upon the VCC which you are using or the amount of bias you are using to get those functions. (Refer Slide Time: 18:54) The resistor Rc has two functions. We have already discussed this point Rc will help you to establish a DC bias, so higher is the value of Rc slope will be lower but with a negative sign, right? And lower the value of RC higher will be the slope with a negative sign. The slope is from the side, it is not like this, it is like this. So you will always understand that the slope is negative in the sense. And second is that more important point is that, IC multiplied by RC is the output voltage, now it is the voltage across the value of, so this is the reason why we convert collector signal current to, so ICRc, right? So VCC minus icRc, is the output voltage. So RC has two components or two issues, one is to establish a DC biased and the collector and the second portion is to convert a signal current, collector signal current Ic to its output value voltage V0.This gives me a basic idea about the basic amplification process of BJT. (Refer Slide Time: 19:51) Let me give you the mathematical treatment here. So if you look very carefully V0 which is in front of you, right? V0 is there, right? If you look at V0, this V0 is the output voltage and is equals to obviously VCE must be equal to VCC minus ICRC, remember? We have already discussed this point. My yesterday’s talk or previous modules talks we have seen that IC was 𝑉𝐵𝐸 equal to 𝐼𝑠 𝑒 𝑉𝑇. IS is the saturation current this is the saturation current which you see, this is the saturation current, right? This is the collector current and VBE is the input voltage in the case of common emitter. In case of common emitter amplifier and therefore I get ICsat to be equals to VCC minus VCEsat by ICRC and I think this is pretty clear. So VCC minus VCEsat by RC gives you ICsat. Now since IC therefore is equal to IS, so VBE is now replaced by VI, we have just discussed this point why? Because I can safely say that the total voltage is a sum of the DC bias which you are giving and the AC bias of the signal which you want to amplify, right? So these are the two important voltage sources which you are trying to do. So I get V0 therefore this statement 𝑉𝑖 it goes to VCC minus RC times IC, IC can be written therefore 𝐼𝑠 𝑒. right? Is it okay? Now 𝑉𝑇 therefore you see very interestingly that the output voltage V0 has an exponential dependence on the value of your input voltage Vin, right? Which means that if Vin increases even by a small amount, I would expect to see a drop in V0 by a large amount because this is an exponential drop which you see. VT is the thermal equivalent voltage which is given as KT by q and this equals to 25 mVs at 300 K. So at 300 K, VT equals to 25 mVs, right? So if your VI is let us suppose is, so if my VI is 25 mVs, right? So e1 will be equals to 0 and therefore I get V0 equals to VCC. Remember as long as VI crosses 0.7, I will expect to see V0 to be equals to VCC because this will effectively go to zero, if not exactly go to zero, right, in the saturation region, which is the saturation region after the active region expands. (Refer Slide Time: 22:50) So, I have got if you look carefully I have got this thing that I have got cut-off region which is this one, right? This is the cut-off region then we have the amplification region with the active mode and I have got a saturation region here. So cut-off region, active mode and saturation it will vary depending on the value of VBE which you are choosing. So depending on the value of VBE we can think of active and saturation region, right? (Refer Slide Time: 23:12) With this knowledge we have gained till now, let me see as what we are looking into. So we are looking into the fact that therefore to operate the BJT as a linear amplifier it must be biased at a point in the active region, we have discussed this point already time and again that we need to bias it, amplifier. And then VI is therefore superimposed on VBE. We again discussed this point in a much more detail manner. So I get 𝑉𝐶𝐸 = 𝑉𝐶𝐶 − 𝐼𝐶 𝑅𝐶 , so I get Av to be equal to 𝜕V0 𝜕VI which means the rate of change of output voltage with respect to change in the input voltage for a fixed VI comes out to be 𝑉𝐵 VBE, right? So Av is equals to VBE. Av also comes out to be equal to -1 by VT, Is 𝑒 𝐼𝑅𝐶 IC. Please do it yourself you’ll find this answer very straightforward and simple, right? And I will therefore get a negative sign expected also because of slope is negative and therefore I should get a negative gain. Negative gain primarily means with increasing voltage, input voltage the output voltage is decreasing and that is the reason we define that to be as a negative voltage. (Refer Slide Time: 24:13) Therefore I get Av to be equals to minus, why did you get Av equals to this thing whole quantity. Now this quantity is basically IC, so I get ICRC by VT. So this is basically the voltage drop across the resistance at the top, right? This is the resistance Rc, so this voltage is basically the drop at this resistance Rc and that is equals to minus VRC by VT, right? Now VRC is equal to VCC by minus VCE, remember why? Because you had a resistance here and you had a, this thing therefore we get VCC is this one, right? And so VRC is this voltage, between these two points and this is VCE, right? So I can write down VCC to be equals to VRC plus VCE, fine. And therefore we can write down VRC to be equals to VCC minus VCE, right? So in saturation this VCEsat will be typically very small and VRC will be approximately equals to VCC and you get a very high gain, right? So, But then you have to ensure that you don’t go into saturation because then VCE sat will be typically very small. So where do you say? You stay within the active region of the operation of the device. So therefore I get Av to be equals to minus VCC minus VCEsat by VT and therefore the maximum value of AVmax is VCEsat, so if I assume this to be equal to 0 approximately I get - VCC by VT as the AVmax. So you see even if I use VCC of 1 volt and if 25 mVs you are getting here, so I will get 1 by 25x10-3, so I get 10+3 here, so this is basically thousand by 25 which is basically 40, right? So even if you don’t do much manipulation and incidences, what you get is primarily that the voltage gain of a common emitter mode configuration at T equals to 300 K happens to be maximum value attainable is basically 40. But then this is assumption that VCEsat equals to 0 which is never. So actually I will get a drop in the value of VCE in the AV value. In reality AV will be much smaller than 40 even, right? And that you have to take care of when you’re actually designing a system. As I discuss with you therefore, the gain is negative, right? The gain is negative which signifies that the amplifier is inverting amplifier. So I have an inverting amplifier available with me and the gain is negative this thing. Further the gain is proportional to the collector bias current IC and a load resistance RC. This we have already seen and you know the reason why the gain is like the collector current and basic reason is there. So before we finish this module of lecture let me recapitulate what we did. We had looked into BJT as an amplifier. We have also understood the various parameters which we need to fix from DC domain in order to bias it in the active region of operation. You cannot bias it in a saturation or in the cut-off region, you have to bias it only in the active region. When you bias it in the active region you automatically get a very large gain. We also saw that theoretically speaking the maximum gain available to us in a common emitter mode configuration is minus 40 times, right? Provided you are giving a VCC of 1 volt, I get VCC by VTS the maximum theoretical limit which you get in the voltage gain. In reality, obviously we will not get because VCEsat will never be equal to 0 it will have some finite value and therefore your AVmax will reduce drastically in that case, right? So this takes care of the basic understanding of our design and in the next turn we will be looking into small single models BJT, right? Thank you very much.