Lec 8 Updated Gene Expression Control PDF
Document Details
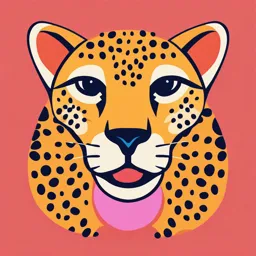
Uploaded by ClaraJeniffer1
University of Silesia in Katowice
2024
Dr. Michelle Kuzma
Tags
Summary
These lecture notes cover the control of gene expression in eukaryotes, focusing on aspects like different types of cells, functions, gene expression processes and mechanisms. The document uses diagrams and examples to enhance understanding of the subject.
Full Transcript
Control of gene expression Lecturer: Dr. Michelle Kuzma Adapted from: Dept. Head, Dr. Danuta Mielżyńska-Švach Molecular biology , 2024/2025 Book Reference Essential Cell Biology by Bruce Alberts, 6th Edition. Focus on what is discussed in the lectures ⚫ Chapter 8: Con...
Control of gene expression Lecturer: Dr. Michelle Kuzma Adapted from: Dept. Head, Dr. Danuta Mielżyńska-Švach Molecular biology , 2024/2025 Book Reference Essential Cell Biology by Bruce Alberts, 6th Edition. Focus on what is discussed in the lectures ⚫ Chapter 8: Control of Gene Expression ⚫ Chapter 9: How Genes and Genomes Evolve Gene expression in eukaryotes Cells in multicellular organisms have lost their independence at the expense of common interest Each cell has its own role to play at a specific time and place The activities of multicellular organism resemble the structure of large metropolis In the case of a prokaryotic cell, however, it resembles small cottage in the middle of nowhere Human genome Eukaryotic gene Gene is a fragment of DNA (a sequence of nucleotides) that contains information about the encoded product Gene is a fragment of DNA that carries information about the structure of: ❑ one polypeptide (mRNA) ❑ one type of functional [non-coding] RNA (rRNA, tRNA, snRNA, miRNA, etc.) Eukaryotic gene A gene consists of the following sequences: ❑ coding - exons ❑ non-coding - introns ❑ regulatory; ❑ promoter ❑ enhancing sequences (enhancers) ❑ silencing sequences (silencers) Eukaryotic gene structure Gene expression in Eukaryotes Genetic material of all somatic cells of multicellular organisms is identical In a differentiated human cell, between 5.000 to 1.000 genes can be expressed, or transcribed, out of a total of about 25.000 protein-coding genes Only about 1.5% of DNA consists of protein-coding genes, the remaining 98.5% is non-protein-coding DNA Gene expression in Eukaryotes Gene expression is the process by which cells selectively synthesize many thousands of proteins and RNA molecules encoded in their DNA In each type of differentiated cell, a specific group of genes is expressed, or transcribed, the set of these genes is constant Cells can change the pattern of gene expression in response to both intracellular and extracellular signals Expression of genes in Eukaryotes In somatic cells of multicellular organisms, most proteins are the same They are called "housekeeping proteins” and include: ❑ DNA and RNA polymerases ❑ DNA repair enzymes ❑ ribosomal proteins ❑ enzymes associated with basic metabolic processes Gene expression in Eukaryotes In mammals, different types of cells perform a number of specialized functions, for example: ❑ pancreatic β-cells produce the hormone, insulin ❑ pancreatic α-cells produce the hormone, glucagon ❑ B cells (lymphocytes) produce antibodies ❑ red blood cells synthesize haemoglobin Differences in structure and function of neurons, white blood cells, red blood cells and pancreatic β cells depends on the process called gene expression Gene expression in Eukaryotes Gene expression in Eukaryotes Cells can regulate its protein set by: ❑ controlling when and how often a given gene is transcribed ❑ controlling steps in the transcript maturation process ❑ selecting which mRNAs will be exported from the nucleus to the cytoplasm ❑ regulating how quickly given mRNA molecules will be degraded ❑ selecting which mRNAs will be translated by ribosomes ❑ regulating the rate of protein degradation Types of expression regulation Eukaryotic gene expression consists of multiple steps including transcription and translation, which are separated in space and time The key regulatory step of most genes is transcription Transcription begins when RNA polymerase and transcription factors bind to the gene promoter In addition to the promoter, most genes also contain DNA regulatory sequences used to turn on or off gene transcription Types of expression regulation Two classes of regulatory sequences are involved in the regulation of gene expression: ❑ cis-type sequences occur on the same chromosome as the gene being regulated ❑ trans-type sequences occur on a different chromosome than the gene being regulated Cis-regulatory sequences Depending on their distance from the transcription start site cis-regulatory sequences can be divided into: ❑ short-range sequences ❑ long-range sequences Short-range cis-regulatory sequences are: ❑ promoters ❑ enhancers and silencers occurring in the 5’UTR region Cis-regulatory sequences Long-range cis-regulatory sequences can be from several dozen to several hundred nucleotides long, which can act: ❑ from distance of several thousand base pairs (700 - 1.000 bp) ❑ regardless of their position (upstream or downstream of the transcription start site) ❑ regardless of their orientation relative to the promoter Regulatory sequences DNA regulatory sequences do not act on their own To act, they must be recognized by proteins called transcription regulators It is the binding of the transcription regulator protein to a DNA regulatory sequence that acts as a switch regulating transcription Transcription regulators can be: ❑ activators that promote gene transcription ❑ repressors that inhibit gene transcription Models for action „at a distance” An activator or repressor protein often binds to enhancer or silencer sequence thousands of base pairs upstream or downstream of the gene promoter. The section of DNA between an enhancer or silencer and promoter forms a loop Creation of a loop in the so-called spacer DNA allows the activator or repressor protein to contact transcription pre- initiation complex associated with a promoter Models for action "at a distance” Often, additional proteins serve as adapters and are termed mediators A mediator acts as linker and allows for proper localization to the promoter of general or specific transcription factors and RNA polymerase (transcription pre-initiation complex) Mediator is absolutely essential for regulating transcription of most eukaryotic genes Models for action "at a distance” Transcription factors In eukaryotic organisms, transcription factors that initiate gene transcription are divided into two groups: ❑ general transcription factors (GTFs) ❑ specific transcription factors (STFs) GTFs remain active at all times, as they are responsible for the correct course of transcription in the basic state STFs are activated or inhibited depending on the cell's demand for specific proteins Transcription factors General transcription factors associated with a promoter are same for all genes transcribed by RNA polymerases Specific transcription factors and the location of their DNA binding sites relative to the promoters vary for different genes Combinatorial controler Trans sequences Trans regulatory sequences are sequences encoding transcription factors that act through interactions of the type: ❑ DNA-protein ❑ protein-protein ❑ sometimes RNA-protein Transcription regulators DNA regulatory sequences, in order to function: must be recognized by transcription regulator proteins when bound to transcription regulator, act as switch regulating transcription There are about 2.000 DNA regulatory sequences in human cells Transcription regulators Transcription regulators are proteins whose surface must fit tightly to the surface of DNA Regulatory proteins: ❑ recognize specific nucleotide sequence and bind to the surface of DNA ❑ form noncovalent bonds with nucleotides within major groove of DNA, but some proteins also interact with bases in the minor groove ❑ DNA-protein interactions are among strongest and most specific molecular interactions Transcription regulators Transcription regulators ad (A) Regulatory proteins recognize DNA using at least three α helices They fit into the major groove and form base pair connections on a short stretch of DNA This structural motif is called homeodomain ad (B) Most protein-base connections in DNA are formed via the third α helix ad (C) Asparagine side chain of the third α helix forms two hydrogen bonds with adenine attached to thymine Transcription regulators The DNA-protein interface may contain 10-20 such connections, each involving different amino acid Regulatory proteins form with DNA: ❑ hydrogen bonds ❑ ionic bonds ❑ hydrophobic interactions Many regulatory proteins interact with DNA as dimers, because dimerization increases the contact area of the DNA-protein interaction Dimerization Combinatorial control Most eukaryotic transcription regulators act as part of large complex in which they cooperate to express gene: ❑ in the right cell type ❑ in response to specific conditions ❑ at the right time ❑ at the required level The term „combinatorial control” refers to the way in which group of proteins, acting together, determines the expression of specific gene. Combinatorial control In eukaryotes, typical gene is controlled by multiple transcription regulators that collectively direct: ❑ mediator assembly ❑ general or specific transcription factors ❑ RNA polymerase ❑ histone-modifying enzymes ❑ chromatin-remodeling complexes Combinatorial control Single transcription regulator can coordinate expression of multiple genes encoding different proteins Different genes can have regulatory sequences: ❑ recognized by same transcription regulator ❑ they will be turned on and off together Example of such coordinated regulation in humans is the response to cortisol Combinatorial control Combinatorial control The ability to turn on and off many different genes using a limited number of transcriptional regulators is useful during embryonic development After each cell division, decision is made to synthesize new regulatory protein Combinations of several transcriptional regulators that arise during embryonic development give rise to many cell types Differentiated cells give rise to all of the daughter cells Cell differentiation Gene expression control Gene expression control can be carried out at the stage: ❑ transcription using; ❑ alternative promoters ❑ post-transcriptional using; ❑ alternative splicing ❑ alternative polyadenylation ❑ mRNA editing ❑ synthesis of non-coding RNAs Alternative promoter Many eukaryotic genes have alternative transcription start sites - alternative promoters They are often tissue-specific Therefore, in different cells and tissues different transcripts and then different proteins are produced from one gene Alternative promoters Dystrophin synthesis in different tissues Alternative promoters In the case of the gene AMY1 on chromosome 1 (1q21) encoding α-amylase: ❑ in salivary glands, specific transcription factor causes transcription to be controlled by first promoter, located upstream of second one ❑ in pancreas, the lack of specific transcription factor causes transcription to be controlled by second promoter, located downstream of the first one Alternative splicing Alternative splicing of pre-mRNA allows for the creation of alternative copies of final transcript by: ❑ incomplete excision of introns ❑ changing the location of exons ❑ creation of different combinations of exons that produce different transcripts of the same gene This allows for creation of many different forms of protein using single gene Alternative splicing Alternative splicing Alternative poly(A) sites Some pre-mRNAs contain more than one set of sequences where cleavage and polyadenylation occurs termed poly(A) sites Specific proteins can bind near the poly(A) sites, activating or inhibiting the use of a particular polyadenylation site Single pre-mRNA can therefore give rise to mature mRNAs with identical coding sequences but differing in stability or location Alternative poly(A) sites Sometimes, in given tissue, one type of cell uses only one poly(A) site, while another type of cell uses a different one poly(A) site In some cells, two poly(A) sites may be used with different frequencies, reflecting their efficiency („strength”), so cell may contain two types of mRNA Alternative poly(A) sites Regulatory elements Alternative poly(A) sites In the case of immunoglobulins, the use of: distal poly(A) site (located distal) results in the formation of membrane form of immunoglobulin M proximal poly(A) site (located proximal) results in the formation of soluble form of immunoglobulin M RNA editing RNA editing involves changing the sequence of the primary transcript as a result of: ❑ insertion or deletion of nucleotides (Ins/Del) ❑ changing nucleotide In mammals, two types of RNA editing have been observed as result of changing nucleotides: ❑ cytosine to uridine (C → U) ❑ adenosine to inosine (A → I), which behaves like guanosine RNA editing Apo-BEC family genes encode deaminase proteins: ❑ in liver cells, mRNA is unedited and is responsible for the synthesis of apolipoprotein B100 ❑ in intestinal cells, mRNA after editing is responsible for the synthesis of the shortened polypoprotein B48 Complete form of ApoB is involved in cholesterol transport Shortened form of ApoB is involved in lipid transport RNA editing Liver Intestine Regulatory RNA Many types of noncoding RNA play important roles in regulating gene expression and are called regulatory RNA Examples of regulatory RNA include: ❑ microRNA (miRNA) ❑ small interfering RNA (siRNA) ❑ long noncoding RNA (lncRNA) miRNA miRNA Precursor of miRNA is short hairpin RNA molecule (shRNA), which undergoes post-transcriptional processing Single-stranded miRNA (about 22 nucleotides) together with proteins forms nuclease complex RISC (RNA-Induced Silencing Complex) RISC-miRNA complex binds to complementary bases in the miRNA sequence to those in the mRNA miRNA Depending on length of the complementary sequence, the target mRNA is degraded: ❑ quickly by the RISC complex nuclease ❑ slowly by cytosolic nucleases The result of these processes is inhibition of translation siRNA siRNA siRNA (small interfering RNA) is formed from long double- stranded RNAi (RNA interference) of exogenous or endogenous origin Long RNAi are cleaved by protein called DISCER siRNA can bind to the RITS (RNA-Induced Transcriptional Silencing) protein complex RITS-siRNA complex attaches to complementary pre-mRNA sequences as soon as they emerge from the RNA II polymerase conducting transcription Transcription elongation is inhibited at this site IncRNA IncRNA Long non-coding RNAs (lncRNAs) are molecules that are more than 200 nucleotides long One of the best known lncRNAs is Xist (17.000 nucleotides), which plays key role in inactivating one of the two X chromosomes in female mammalian cells Some long non-coding RNA molecules: ❑ fold into specific spatial structures ❑ can place proteins on specific DNA and RNA sequences Epigenetyka Epigenetics - science that studies changes in gene expression that are not related to changes in DNA nucleotide sequence In the term epigenetics, where the prefix „epi” means „beyond something” or „in addition to” Gene expression via epigenetic mechanisms can: ❑ be subject to inheritance ❑ be modified by outside factors Epigenetic regulation DNA together with proteins creates chromatin, which can be condensed to varying degrees Degree of condensation affects the availability of proteins associated with DNA transcription: ❑ heterochromatin, that is, highly condensed chromatin, is transcriptionally inactive ❑ euchromatin, i.e. loose chromatin, may be transcriptionally active Epigenetic regulation Epigenetic regulations change structure of chromatin, which affects the regulation of transcription process without changing DNA sequence and include: ❑ DNA methylation ❑ histone modification ❑ chromatin reconstruction (remodeling) ❑synthesis of non-coding RNAs DNA methylation NH2 NH2 CH3 N N N N O O ~ ~ cytosine 5-methylcytosine DNA methylation The formation of 5-methylcytosine occurs by methylation of cytosine in DNA with the participation of DNA methyltransferase In vertebrates, this modification is limited to specific cytosines (C), located next to guanines (G), in the sequence 5’-CG-3’ (CpG) In mammalian DNA, about 60% of cytosine nucleotides are methylated 5-methylcytosine in DNA is recognized by proteins from the family MBD (Methyl-CpG Binding Domain) DNA methylation Attaching methyl group to cytosine: ❑ causes closure of given area of c hromatin ❑ blocks gene transcription DNA methylation DNA methylation is responsible for phenomena such as: ❑ cellular identity ❑ X chromosome inactivation ❑ gene reprogramming ❑ genetic imprinting Cellular identity DNA methylation patterns are inherited during cell division in S phase After DNA replication, each double-stranded daughter helix contains: ❑ one methylated DNA strand (inherited from the parent helix) ❑ second unmethylated DNA strand (newly synthesized) Conservative methyltransferase: ❑ interacts with daughter strand that is unmethylated ❑ participates in methylating only those -CpG- sequences that are methylated in the parent strand Cellular identity X chromosome inactivation In female mammals, one copy of X chromosome in each cell is epigenetically disabled by cytosine methylation X chromosome inactivation The fur color of certain species of cat is determined by the orange gene, located on the X chromosome Female that is heterozygous for the orange gene exhibits black and orange fur, which correspond to cells with gene disabled on one or the other X chromosome X XX XX XX XX XX XX XX XX XX XX XX XX Epigenetic reprogramming cycle In the fetal germline all DNA methylation patterns are erased (gray line) and then paternal (blue) and maternal (red) methylation imprints are established during gametogenesis The two germline genomes that are combined at fertilization undergo parent-specific genome reprogramming in the early embryo, during which most germline patterns are erased again and somatic patterns (green) are established Only imprinted genes maintain their germline patterns during development of the new organism Epigenetic reprogramming cycle Chromatin structure Histone structure Histone proteins (H2A, A2B, H3 and H4) are composed of globular part and the so-called tail extending beyond the nucleosome H4 H4 H3 H3 H2A H2A H2B H2B Histone modification Histones that are part of nucleosome are subject to reversible modifications Each histone tail can be modified by covalent attachment of many different chemical groups Most common modifications are: ❑ phosphorylation and dephosphorylation (P) (serine, threonine) ❑ acetylation and deacetylation (Ac) (lysine) ❑ methylation and demethylation (M) (lysine, arginine) ❑ ubiquitination Histone modification Histone modification Modified amino acids (letter abbreviations) in the histone tail are marked with numbers For example, histone H3 contains 135 amino acids, most of which are located in globular part 36 amino acids located on tail, mainly near the N-terminus, are subject to modification Lysines can be modified with one, two or three methyl groups Symbols of amino acids Histone modification Histone acetylation causes loosening of chromatin (euchromatin) and increased gene expression Histone deacetylation causes tighter packing of chromatin (heterochromatin) and inhibition of gene expression Histone modification Methylation of lysine in histones has various effects Euchromatin formation and increased gene expression: ❑ H3 K4 me3 ❑ H3 K36 me3 ❑ H3 K79 me3 Heterochromatin formation and decreased gene expression: ❑ H3 K9 me3 ❑ H3 K27 me3 ❑ H4 K20 me3 Histone modification Histone modification During DNA replication, its histones are randomly distributed to daughter chromosomes Each daughter chromosome inherits half of the set of modified histones The remaining sections of DNA receive newly synthesized, as yet unmodified histones Proteins responsible for histone modifications: ❑ bind to the modified histones ❑ introduce the same modifications to new histones located nearby Histone modification Chromatin remodeling Chromatin remodeling involves the condensation and loosening of specific areas as result of the action of: ❑ histone-modifying enzymes ❑ chromatin-remodeling complexes Chromatin remodeling Chromatin remodeling Many gene activators and repressors pull proteins to promoters by recruitment, e.g.: ❑ histone acetyltransferase, which causes the attachment of acetyl groups to tails of histone proteins, which stimulates gene transcription ❑ histone deacetylase, which removes acetyl groups from the tails of histone proteins, which limits gene transcription Chromatin remodeling Histone modifications cause chromatin condensation wave to propagate (spread out) until it encounters barrier, i.e., an appropriate DNA sequence Some barrier sequences contain binding sites for enzymes that add acetyl group to lysine 9 of the histone H3 tail This modification blocks methylation of lysine 9, preventing further spreading of heterochromatin formation Chromatin remodeling Histone octamer shift One mechanism of chromatin remodeling involves shifting histone octamer along DNA strand Protein complexes use energy from ATP hydrolysis to loosen nucleosomal DNA and push it out of the nucleosome In this way, the DNA sequence can be exposed, making it more accessible to proteins that enable transcription of given gene Histone octamer shift The blue bars show how the DNA changes position Interference RNA RNAi most likely arose as defence mechanism against viruses and is present in all eukaryotes Some RNAi can inhibit transcription of specific genes through: ❑ DNA modifications ❑ RNAi-dependent DNA methylation ❑ histone protein modifications Literature