Lab Notes CHEM 2740 PDF
Document Details
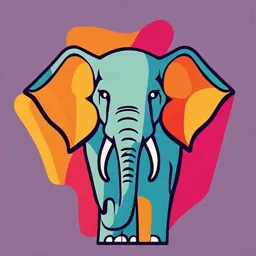
Uploaded by AccurateAgate4174
Tags
Summary
These lab notes cover pH and buffers, including the Henderson-Hasselbalch equation and its implications. They discuss buffer capacity, method considerations for selecting and preparing a buffer, and provide some examples. The document appears to be classroom notes rather than a formal exam or textbook.
Full Transcript
pH and Buffers hydrogen ion concentration and pH water dissociates according to the equation not a complete dissociation. Therefore, equilibrium is established. ion product of water (Kw): pH=...
pH and Buffers hydrogen ion concentration and pH water dissociates according to the equation not a complete dissociation. Therefore, equilibrium is established. ion product of water (Kw): pH= -log [H+] pH of electrically neutral water at 25ºC is : -log [1 x 10^-7] = 7 solutions containing ions are capable of carrying an electrical current in natural biological systems pH is maintained by the activity of many of the other molecules that are present and one of the names for these molecules regulating pH is BUFFERS. Buffers are classes of molecules whose presence in a system minimizes the pH changes when an acid of base are introduced into the system. when studying biometrics in vitro the biochemical buffering process that provide a stable pH environment in Vito are absent, and therefore the researcher must artificially control pH to ensure that any results that are obtained are not artefacts that have arisen be cause of pH-dependant effects. How buffers control pH buffers are usually weak acids and as such do not fully dissociate in a solution but rather exist as a mixture at equilibrium. WA equilibria are often denoted as: where HA is the weak acid and A- is its conjugate base. If an acid were added to a buffer solution to would be neutralized by the presence of its conjugate base: similarly, the addition of a base would be neutralized by its reaction with HA Henderson-hasselbalch equation: implications of the Henderson-hasselbalch equation 1. The pH of the buffer is determined by the ratio of the concentrations, not just the concentrations alone. pKa is 7, HA (weak acid) was [20mM], A- (conj. Base) was [30mM], the pH would be 6.82. but if pKa is 7, HA was [40mM], A- was [60mM], the pH would still be 6.82. 2. If HA and A are present in equal concentration; the pH of the solution will be equal to the pKa. This is because the log of 1, (A/HA in this case is 1), equals 0. If the conc. Of the conjugate base is greater than the weak acid (HA) the value of the ratio will be greater than 1 and therefore the pH will be greater than the pKa. Similarly, if more acid form is present the pH will be less than the pKa. 3. It’s very important to be aware that the Henderson-hasselbalch equation implies that buffers do not prevent change, but rather, they simply function to minimize change. 4. The H-H equation also implies the ability of a buffer to resist changes in pH upon the addition of acid or base diminishes precipitously at pH values + or - 1 pH unit of the pKa. important considerations for selection and preparation of a buffer selecting a buffer: the first most obvious selection criteria for choosing a buffer in a biochem lab is its pKa. The ability to resist changes of pH upon the addition of acid or base was strongly influenced by how close the pH is to the pKa of the weak acid form of the buffer. typical rule of the thumb is to select a buffer that has a pKa NO MORE than 1 pH unit of the pH at which you wish to perform the experiment. Ideally, you would want the pKa of the buffer you choose to be the same as the pH. A compromise is choosing a buffer with a pKa within about 0.5 pH units of the intended working pH. buffer capacity: the pH does in fact change upon the addition of an acid or a base, even when the appropriate buffer is present. 2nd important variable that determines how much the pH changes is the absolute amount of buffer that is present, this property of a buffer is known as its buffer capacity (B). Buffer capacity is defined as the number of moles of [H+] that is required to lower 1L of the buffer 1 pH unit. consider a situation the pKa is 8.0, the pH is also 8.0. This means there is equal HA and A present. To make it easier to understand assume there is only 10 molecules of the buffer present which means there must be 5 molecules of HA and 5 of A. Now say you are going to put 4 molecules of NaOH into the solution which will ionize into Na+ and OH-. You know that those 4 molecules of HA will be converted to A. Now A has 9 molecules and HA has 1. You can calculate the new pH using the HH equation: this is a substantial change in pH if you repeat the same scenario with 100 molecules and add the same 4 molecules of NaOH. The new pH of this scenario would be: the buffer with 100 molecules has greater buffer capacity than the one with 10 molecules. Therefore, the greater the moles of buffer, the greater the buffering capacity. For buffers of equal volume and pH the higher the concentration of the buffer, the greater its buffering capacity (remember molarity x volume = moles). Preparing buffers using the Henderson-hasselbalch equation dissolve the buffer to the working concentration in water then adjust the pH with NaOH until the desired pH is attained. This requires that one has functional and reliable pH meter available. as an example for how to prepare a buffer using the HH equation, assume you have been asked to make 1L of 50mM HEPES buffer to a pH of 7.3 and you have the following reagents available: -HEPES (weak acid form—HA), MW (molecular weight): 238.3 g/mol -HEPES sodium salt (conjugate base form — A-), MW: 260.3 g/mol - 1M NaOH - 0.4M HCl note: HEPES has a pKa of 7.55 start by calculating how much of the 2 forms of HEPES you require. To determine this use the HH to calculate the ratio of A/HA that will give you the desired pH start by rearranging the equation to solve for the ratio of the 2 forms of HEPES this ratio tells us the relative amount of HA we need to its A, but does not tell us how much of each needed to make the buffer. we know that [HA] + [A] = 50mM and we know that [A]/[HA] = 0.562, we can rearrange the second expression as: and since [HA] + [A] must equal 50mM, this means that the concentration of A- much equal 50mM minus 32.01mM which gives 17.99mM. To verify you are correct you can plug in these concentrations into the HH equation and solve for pH. remember the concentration of a buffer is the sum of the concentration of HA and the concentration of A. now that you know the concentration of the weak acid (HA) and conjugate base (A) required to make a 50mM HEPES buffer for pH 7.3. How do you make it ? There are 3 methods Method 1. Weigh out the correct amounts of HEPES and HEPES sodium salt and dissolve them in a final volume of 1L of H2O. mass of HEPES required = 238.3 g/mol x 1L x 32.01mM =7.628g Mass of HEPES sodium salt requires = 260.3 g/mol x 1L x 17.99mM = 4.683g dissolve the 2 together to a final volume of 1L method 2. Weigh out enough HEPES, (HA), to give 50mM, dissolve it in H2O and then add NaOH to generate the required amount of the conjugate base form fo HEPES. You can then bring the final volume to 1L mass of HEPES required = 238.3 g/mol x 1L x 50mM = 11.915g M1V1=M2V2 (1M)(V1) = (0.01799M) (1L) (V1) = 17.99 mL 3. Absorption, Absorbance and the beer lambert law theory of absorption electromagnetic radiation is composed of electric and magnetic vectors oscillating at right angles to each other in the direction of their propagation. magnetic and electric components of light the distance between two consecutive peaks is called wavelength. absorption is the interaction of electromagnetic radiation with matter, and is a quantum phenomenon (the details far beyond scope of the course). Absorption can be explained by envoking the photon, which is the elementary particle responsible for carrying electromagnetic radiation. Absorption can be conceived of as a transfer of the energy carries by the photon to the molecule that absorbed it. The energy of photons (E) can be described as: since h and c are constants, the energy of a photon is inversely proportional to its wavelength: this means that light of shorter wavelengths is of higher energy than light of longer wavelengths. jablonski diagram shows the different energy states in which a hypothetical molecule can exist. Horizontal lines represent different energy levels. And each line represents a very specific energy state that is determined by the rules of quantum mechanics. The S0 level represents the lowest energy state of the molecule or the so-called ground state. The other S levels (S1,S2,S3) represent different energy states with each successive S-level representing higher energy states. within any particular energy level (S1,S2, etc.) there are additional different energy levels arising from different vibrational states. These are indicated as V1,V2,V3 etc. in the diagrams. note that each of the higher S energy levels has within its own vibrational states (these are not numbered, but are displayed as the thinner horizontal bars). electrons can exist in many different energy states within a single molecule, and that these states exist at very specific and defined energy levels. when a photon strikes a molecule there are 2 possible fates: it can either be scattered away from the molecule in some manner or it can be absorbed. what determines if the photon is absorbed? If the energy of the incoming photon exactly matches the energy different between two distinctive energy levels, the photon can be absorbed and an electronic transition takes place whereby an electron in ground state is excited to a higher energy orbital. This entire process is what is know as absorption. absorption is a process by which the energy of a photon is transferred to a molecule inducing electronic rotational or vibrational transition in the molecule. This process is very rapid and takes approximately 10^-15 seconds to occur. the molecule only remains excited for a very short period of time (roughly 10^-12 seconds), after which internal processes in the molecule and interactions with the solvent results in the return of the excited electron to one of the various vibrational rotational levels associated with the ground state. The return of the excited electron to a lower energy state is couples with the loss of heat. direction of absorption for absorption to be truly useful to us in the lab we must have a way of measuring and quantifying it. The easiest way is to shine a light on something and see how much light gets through the substance.the technical challenges of controlling and measuring this are of course quite complex and some of the details that one must consider are listed below: 1. What is your source of light? 2. How do you select the correct wavelength 3. How do you direct and control the light shining on your sample? 4. How do you measure the light that goes through the sample? 5, how do you quantify the process of absorption into something useful? below is a brief description of spectrophotometers the most commonly used instrument to measure absorption of light by substance. light source there are a variety of light sources, and the type of light used depends on the wavelength that the instrument is designed to operate at; the consistency of the light source intensity and wavelength output; durability and cost. examples of these light sources and their primary light emission ranges include halogen lamps 350-2500nm deuterium lamps