L6 Regulation of Prokaryotic Transcription PDF
Document Details
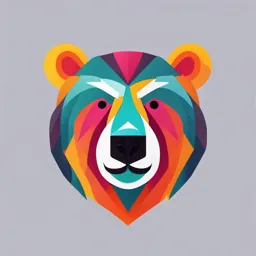
Uploaded by TimeHonoredLimerick2759
null
Tags
Summary
This document provides an overview of the regulation of prokaryotic transcription. It discusses different mechanisms for regulating gene expression, including operons, promoters, terminators, and other regulatory elements.
Full Transcript
L6 Regulation of Prokaryotic Transcription Lecture Aim The aim of these lectures is to learn different mechanisms responsible for regulating expression of genetic information in prokaryotes. Learning Outcomes...
L6 Regulation of Prokaryotic Transcription Lecture Aim The aim of these lectures is to learn different mechanisms responsible for regulating expression of genetic information in prokaryotes. Learning Outcomes By the end of the lecture successful student should be able to: 1. Name key mechanism responsible for regulating gene expression in prokaryotes 2. Sketch structural organisation of bacterial operon 3. Explain with examples how gene expression is regulated at the level of transcription initiation in prokaryotes 4. Describe known mechanisms that control the completion of transcription of prokaryotic genes Why do we need to know about transcriptional regulation? Knowledge of transcriptional and post-transcriptional gene regulatory mechanisms is not only of fundamental interest, but it may also lead to: economically/industrially relevant applications: the construction of well-characterized artificial gene expression systems for microbial synthesis of diverse (bio)chemicals from renewable resources. applications in human and animal health care, such as: diagnostics, therapeutics, bioremediation, and the development of specific gene silencing antibacterial drugs. Need for new antibiotics due to resistance. New antibiotics could inhibit gene transcription. Points to remember Transcription - is the process of synthesis of RNA molecule by enzyme RNA polymerase using DNA as a template, also a first step in gene expression. The DNA double helix must partially unwind for transcription to occur, this unwound region is called a transcription bubble. Transcription is asymmetric – only one strand of the DNA is used as a template. The RNA is transcribed continuously. Each new RNA molecule is called a transcript. The region of DNA from which RNA is transcribed is called a transcription unit. 2 Transcription in Prokaryote vs Eukaryote Histone-like Histones DNA proteins DNA Chromatin Nucleoid RNAP RNAP Transcription RNA Nucleus mRNA Ribosome Translation mRNA Protein Ribosome Cytoplasm Protein Cytoplasm Prokaryote Eukaryote Transcription regulated differently In eukaryotes: transcription in nucleus, translation in cytoplasm. In prokaryotes: transcription and translation both in cytoplasm Eukaryotes have histone proteins which bind DNA in the nucleus to form chromatin. Prokaryotes have histone like proteins, but the interaction is not as strong in euk cells. Nucleoid region weakly formed and easily dispersed by other proteins. Transcription easier in prokaryotes than in eukaryotes. 3 Operon Upstream Downstream RNA-coding region Nontemplate strand UP element Promoter Operator Structural gene-coding region Terminator 5’ 3’ 3’ Gene 1 Gene 2 Gene 3 5’ Template strand Transcription Transcription start site termination site Prokaryotic genes are in a unit -operon. A few genes are regulated by one promoter. Whole thing = transcription unit (Operon + UP) Transcription steps The mechanism of transcription completes in three important steps: Transcription unit Initiation – after RNA 1. Initiation 1 5’ 3’ Promoter Terminator 3’ 5’ polymerase binds to the promoter, the DNA strand RNA polymerase Template strand unwinds and the enzyme Formation of closed complex Transcription start point, (+1) initiates RNA synthesis at the start point on the template Formation of open complex strand Formation of tertiary complex Elongation – the RNA 2 5’ 3’ 3’ 5’ polymerase moves Direction of transcription downstream, unwinding the 2. Elongation Unwound DNA RNA Transcript DNA and elongating the RNA transcript 5’ – 3’. In the wake of transcription, the DNA strands 3. Termination re-form a double helix. Rho factor depended 5’ 3’ 3’ 3’ 5’ Termination – eventually, the Rho factor independent Unwound DNA 5’ RNA Transcript 3 RNA transcript is released and the RNA polymerase detaches from the DNA. 5’ 3’ 3’ DNA 5’ 5’ 3’ Complete RNA transcript RNA polymerase 4 Key points The first nucleotide in the transcript is defined as position +1 of the transcription unit. Enzyme which catalyses transcription is called DNA-dependent RNA polymerase. Bacteria and archaea only have one multi-subunit RNA polymerase. Successful transcription requires RNA polymerase to be recruited to a cis- acting promoter site upstream of the RNA-coding region. Prokaryotic RNA polymerases bind to DNA promoter region directly, unlike eukaryotic RNA polymerases. An operon is a functioning unit of DNA containing a cluster of genes under the control of a single promoter. Eukaryotic cells have 3 RNA polymerases compared to 1. Cis acting – promoter sits on same strand as the rest of the template DNA from which RNA is transcribed. The bacterial RNA polymerase exists in two states. Core enzyme, can catalyse RNA synthesis, but is unable to bind to promoter targets in DNA. Holoenzyme, is capable of both RNA synthesis and promoter recognition. Both forms of RNA polymerase posses two α subunits, and the β and βʹ and ω subunits. The holoenzyme form contains an additional subunit, σ, and it is this subunit that facilitates promoter DNA recognition. 2 alpha subunits, 1 beta, 1 beta prime, 1 omega Holoenzyme also contains sigma, which facilitates DNA recognition 5 β β α α + α σ α σ ω ω β' β' Core enzyme Holoenzyme Subunit structure α2ββʹω Subunit structure α2ββʹωσ No specific promoter binding but Specific promoter binding but tight non-specific DNA binding weak non-specific DNA binding Core sub units of E.coli RNA polymerase Subunit (gene) Size (MW) Functions α (rpoA) 329 aa RNAP assembly (NTD), interactions with DNA and (36.5 kDa) transcription factors for transcriptional regulation β (rpoB) 1342 aa DNA and RNA binding, RNA synthesis, NTP binding, RMP (150.4 kDa) binding site, σ factor binding 2+ β’ (rpoC) 1407 aa DNA binding, RNA synthesis, catalytic Mg coordination, (155.0 kDa) ppGpp binding site 1, σ factor binding ω (rpoZ) 91 aa RNAP folding, ppGpp binding site 1 (10.2 kDa) A core polymerase (with subunit structure α2ββʹω) can transcribe DNA into RNA inefficiently and nonspecifically. σ Factors (α2ββʹωσ) recruit the core RNA polymerase to recognize promoters with specific DNA sequences Difference in subunits due to molecular weight 6 Sigma (σ) factors Sigma (σ) factors are bacterial transcription factors that reversibly bind RNA polymerase (RNAP) and mediate transcription. σ Factors play 3 major roles in the RNA synthesis initiation process: target RNAP holoenzyme to specific promoters, melt a region of double-stranded promoter DNA and stabilize it as a single-stranded open complex, and interact with other DNA-binding transcription factors to contribute complexity to gene expression regulation schemes. Bacterial σ factors are classified into two evolutionarily distinct families: one σ54 factor and several members of σ70. Members of the σ70 family in E. coli are σ24, σ28, σ32, σ38 and σ70 Interestingly, E.coli has 7 different σ factors, Bacillus subtilis 18 and myxobacterium Sorangium cellulosum - 109. Sigma factors/subunits enable binding of RNA polymerase to DNA Sigma 54 = 1 sigma 54 Reversibly bind to core RNA polymerase Regulate gene expression. Different prokaryotic cells have different levels of transcription regulation – shown by different number of sigma factors and transcription factors. E.Coli sigma ( factors Number of amino acid Size Factor Gene Consensus binding site Genes regulated residues (kDa) σ70(σD) rpoD 613 70 TTGACA–N17–TATAAT Housekeeping rpoN σ54(σN) 477 54 CTGGCAC–N5–TTGCA Nitrogen metabolism (ntrA) rpoS TTGACA–N12– σS 362 38 Stationary phase (katF) TGTGCTATACT rpoH σ32(σH) 284 32 CTTGAA–N14–CCCCATNT Heat shock (htpR) σF(σ28) fliA 239 28 TAAA–N15–GCCGATAA Flagellar proteins σE rpoE 191 24 GAACTT–N16–TCTGA Extreme heat shock σfecI fecI 173 19 GGAAAT–N17–TC Iron transport 7 Sigma ( factors The seven σ factors of E. coli all bind Factor Consensus binding site competitively to similar regions of the σ70(σD) TTGACA–N17–TATAAT unique core RNAP but interact with σS TTGACA–N12–TGTGCTATACT specific promoter sequences around σ32(σH) CTTGAA–N14–CCCCATNT positions –10 and –35 for the six σF(σ28) TAAA–N15–GCCGATAA members of the σ70 family and around σE GAACTT–N16–TCTGA –12 and –35 for σN , the sole σfecI GGAAAT–N17–TC representative of the σ54 family. σ54(σN) CTGGCAC–N5–TTGCA All sigma factors in sigma 70 group recognise -35 and -10 groups Sigma 54/N recognises -12 and -24 RNA polymerase and σ factor cycle β σ + α α ω β' Core enzyme Regulatory σ factors Regulatory factors cycle cycle factors cycle cycle β β β 5’ α σ α α α α α 3’ DNA 3’ Structural gene-coding region Terminator 5’ ω Promoter ω ω β' Transcription β' β' Transcription start site termination site Holoenzyme Core enzyme RNA Initiation Elongation Termination Sigma factor only important when guiding RNA polymerase to promoter region. Sigma factor released after binding during initiation. Transcription can be blocked or enhanced by regulatory factors for short periods of time. 8 RNA polymerase interactions at promoter ω αNTD β' αNTD β αCTD α CTD σ 2.1 σ σ2.3 σ 4.2 2.4 σ3.0 σ1.2 σ1.1 TTGACA 17(+-1) bp EXT TATAAT DISC +1 DS DNA UP element -35 Box Inter-base distance -10 Box Discriminator Transcribed region Each α-subunit consists of independently folded amino-terminal (NTD) and carboxy- terminal (CTD) domains that are joined by a flexible linker. The β-subunit and βʹ-subunit are assembled by binding to the N-terminal domains of the α-subunits, and form a cleft that contains the active site, whereas the ω-subunit binds. EXT = extension region TATAAT box = Pribbenow/-10 box. Alpha subunits have N terminal region which interacts with beta prime C terminator domain recognises up elements and contributes to specificity. Alpha and sigma subunits directly bind to DNA Beta and beta prime are active site subunits with enzymatic functions e.g., cutting, forming new RNA. Omega subunits helps hold nucleotides. 9 Initiation of transcription from a prokaryotic promoter Initiation of transcription from a prokaryotic promoter occurs in several steps: binding of RNA polymerase to the promoter to form a closed complex; isomerization of the closed complex to an open complex; conversion of the open to an initiating complex and formation of an elongating complex. Beginning: sigma factors recognise promoter region and bring RNA pol to DNA 2 strands separated and transcription bubble formed. RNA polymerase starts making new mRNA. Sigma factor released. Guiding initiation of transcription from a promoter The amount of productive initiation of transcription from a promoter is guided by the presence of a combination of distinct DNA sequence elements in the promoter: the UP element (AT-rich), the −35 element (TTGACA), the ex-10 element (TG), the −10 element (TATAAT), the discriminator (Dscr) element (G/C- or A/T-rich, −6 to −1), and the transcription start point (+1) What guides initiation? Promoter elements. Stronger promoter = more mRNA 10 Bacterial promoter architecture Consensus Consensus Transcription start site UP Element TTGACA 17(+-1) bp Ext TATAAT Dscr +1 Transcribed region -35 Box Inter-base distance -10 Box Discriminator Transcribed region The principal DNA elements that are recognized by RNA polymerase at bacterial promoters include: the UP element (positions −37 to −58, from the transcriptional start site denoted +1), the −35 Box (positions −35 to −30), the extended −10 element (Ext; positions −17 to −14), the −10 Box (positions −12 to −7) and the discriminator element (Dscr; −6 to −4) Promoter region needs to be close to consensus region to be considered a strong promoter unless it has a specific sigma factor to recognise it. **Remember promoter architecture to predict how strong the promoter is. Strength of a promoter Strength of a promoter depends on promoter sequence and its architecture. Promoters can be: constitutive (regulated by RNA polymerase levels or sigma factors), positively regulated (increased promoter activity based on some transcription (trans- activating) factor, also known as an activator, increases in cellular concentration), and negatively regulated (decreased activity as a repressor protein (trans-activating element) is present). Promoters are often referred to as being inducible - that means there is always some repressor present to inhibit the promoter activity, or that a promoter has low activity (RNA polymerase binding) until an activator is present. Some promoters have both activators and repressors protein binding region. Both repressor and activator proteins are often regulated by a ligand (small molecule) created by the bacteria or exogenously added to the bacteria's environment. Genes that are regulated by constitutive promoters are expressed constantly within the cell. Positively regulated – requires factors to activate transcription. 11 Negatively regulated – not activated until there are some trans activating elements are removed. Inducible promoter – negatively regulated and needs inducer or promoter to become positively regulated. Some promoters can have activators and repressors or be regulated by environmental factors END OF VIDEO 1 Mechanisms regulating RNAP activity Subcellular localisation Proteolytic turnover Limited proteolysis Covalent modification Rate of synthesis Sequestration Strength of a promoter Presence of activators, repressors, operators and inducers Subcellular localisation – RNAP close to where transcription could happen therefore more mRNA can be made Proteolytic turnover – how quickly RNA is degraded Proteolysis – factors that affect RNA degradation Covalent – factors modify RNA Sequestration – factors inhibit activity of RNA polymerase by adding new nucleotides 12 Key points to remember Activators - gene products that increase transcription, indicating that their function is to enhance promoter activity. Repressors - gene products that decrease transcription, indicating that their function is to hamper promoter activity. Operator - a genetic entity adjacent to a group of genes that regulates their expression and is sensitive to a repressor. Inducers are small molecules that either activate or repress transcription. Inducers basically help by binding to a repressor or activator, they don’t work alone. Regulation of transcription initiation Transcription initiation can be regulated at the level of: the formation of RNA polymerase holoenzyme, promoter recognition by RNA polymerase or RNA polymerase activity. Transcription initiation can be mediated by various factors, which can either: modulate the function of RNA polymerase itself, or can modulate the accessibility or affinity of promoters for RNA polymerase. Regulation factors can bind directly to the RNA polymerase or can target the promoter DNA Can be regulated through different steps 13 RNA polymerase-centred regulation Many factors interact directly with bacterial RNA polymerase to influence its activity at different promoters. These factors include sigma factors and various other proteins and ligands that regulate either the formation of RNA polymerase holoenzyme or its activity or promoter preferences. Regulation by direct interaction with RNA polymerase often by small or medium proteins. This includes sigma factors Role of sigma ( factors The role of sigma factors is to guide the positioning of RNA polymerase molecules at promoters and then to orchestrate the formation of the open complex. The housekeeping sigma factor and alternative sigma factors bind to the same site on the surface of the RNA polymerase core enzyme but the housekeeping sigma factor is more abundant and thus able to outcompete alternative sigma factors Role = to guide RNA polymerase to the promoter region Enables RNA polymerase to transcribe DNA Housekeeping sigma factors more abundant so outcompete alterative sigma factors 14 Sigma factors transcription initiation Unlike the complex that is formed by σ70, RNA polymerase holoenzyme that contains σ54 is unable to proceed to the open complex and requires activation by a special class of ATP-dependent activators. Some sigma factors do not need any help (sigma 70) Some need ATP or another energy source Regulators of holoenzyme activity Some of these regulators are restricted to particular groups of bacteria For example, RbpA and CarD in Mycobacterium tuberculosis bind to and stabilise RNAP open complexes. In E.coli extended coiled-coil protein motif in DksA in cooperation with ppGpp, selectively stabilizes or destabilizes open RNA polymerase–promoter complexes Proteins can regulate holoenzyme activity in specific activity. Has potential for new antibacterial drug 15 Regulators of holoenzyme activity cont. Other factors decrease the number of RNA polymerase molecules that are available for transcription by sequestering the holoenzyme. One example is 6S RNA, an approximately 180-nucleotide non-coding RNA that is synthesized in response to slow growth forms a 1/1 complex with the RNA polymerase holoenzyme. In E. coli, 6S RNA is a mimetic for the DNA of promoters that are targets for the housekeeping RNA polymerase holoenzyme that contains σ70. Prevents enzyme from functioning Regulators of holoenzyme activity cont. Some phages have factors that inhibit the activity of bacterial RNA polymerases to favour the activity of their own bespoke RNA polymerases. For example, during infection of E. coli cells, the Gp2 protein of phage T7 induces a conformational change in a part of domain 1 of σ70 that blocks the access of template DNA to the active site. The P7 protein of the related phage Xp10, which infects Xanthomonas oryzae, inhibits the activity of RNA polymerase by displacing the sigma factor from the holoenzyme. Phages can inhibit the activity of RNA polymerases e.g., by displacing sigma factors. 16 Appropriation of RNA polymerase Some regulators of RNA polymerase holoenzyme, known as ‘appropriators’, remodel parts of the polymerase to alter promoter preferences. The T4 phage AsiA and MotA proteins redirect the housekeeping RNA polymerase holoenzyme from the transcription of host genes to the transcription of phage genes Appropriators model the polymerase to alter promoter preferences. Appropriation of RNA polymerase cont. Early during infection, the phage T4 protein Alt ADP-ribosylates amino acid residue R265 on the carboxy-terminal domains of the α-subunits of E. coli RNA polymerase (αCTD). This modification modulates the recognition of UP element sequences in bacterial promoters, thereby increasing the availability of RNA polymerase for transcription of phage genes. Takes ADP ribose and adds it to R265 on alpha subunit so the RNA cannot recognise that specific area of the promoter. 17 Appropriation of RNA polymerase cont. In E.coli SoxS targets RNA polymerase holoenzyme to promoters that have upstream Sox-box sequences and is essential for the induction of dozens of genes in response to the sensing of oxidative stress by the SoxR repressor, which triggers an increase in the abundance of SoxS. SoxR repressor binds to Sox box and Sox S brings RNA polymerase and induces transcription of genes responsible for sensing oxidative stress. Appropriation of RNA polymerase cont. In Bacillus subtilis, the Spx protein is activated in response to thiol- oxidative stress by binding with the C-terminal domains of the α- subunits of RNA polymerase, which modulates the binding preferences of RNA polymerase and increases expression of genes such as trxB used to combat thiol-oxidative stress. Spx protein, in response to thiol oxidative stress, binds to C terminal domain and modulates binding site of RNA polymerase and increases expression of genes to combat thiol oxidative stress. 18 Regulators of holoenzyme activity by (NTP) substrates In addition to regulation by proteins, the activity of RNA polymerase holoenzyme can be regulated by fluctuations in the levels of its four nucleoside triphosphate (NTP) substrates. The required concentration of the initiating NTP is higher than that of subsequent NTPs, which means that the concentration of the initiating NTP is most crucial to the activity of the RNA polymerase. As the initiating NTP for rRNA transcripts in E. coli is ATP, transcription initiation at rRNA promoters is expected to be sensitive to the cellular concentration of ATP. Higher NTP concentration increases RNA synthesis RNA promoters sensitive to concentration of ATP e.g., sigma 54. Not enough ATP = low transcription rate. Genes regulated by sigma 54 will not be expressed. Anti-σ factors Some σ factors are controlled by specific anti-σ proteins that impede RNAP binding. Anti-σ factors stabilise the σ in a form that is incompatible with RNAP binding by occluding the key RNAP binding determinants. Anti-σ factors are often modular, consisting of a σ-binding domain and a sensory/signalling domain that responds to a signal either within or outside of the cell. Anti-σ factors are often co-transcribed with σ factor genes, which might help to ensure that stoichiometric levels are maintained. Proteins that bind to sigma factors directly and destabilise their interaction with RNA polymerase or stop sigma factor binding to promoter region. 19 Promoter-centred regulation Regulation by factors that bind directly to the RNA polymerase is complemented by factors that directly target the promoter DNA. Promoter regulation by transcription factors generates a complex regulatory network in which the concerted activities of specific, global and master regulators orchestrate the distribution of RNA polymerase to the various transcription units that are present in the genome. It is important to appreciate that transcription initiation occurs in the context of the bacterial nucleoid, and that the compaction of the bacterial chromosome that occurs during the formation of the nucleoid is thought to have an overall negative effect on promoter activity. Class I activation Class I activators bind to upstream locations, usually near position - 61, -71, -81 or -91, and function by making a direct interaction with the carboxy-terminal domain of the RNAP a subunit (α CTD). This interaction recruits αCTD, and hence the rest of the RNA polymerase, to the promoter. The location of activator binding at promoters subject to Class I activation is variable because the linker between αCTD and the α amino terminal domain is sufficiently flexible to permit the binding of αCTD at different positions. Regulators divided into different classes Activators: Bind upstream from -35 region e.g., 61-91. Function: make direct interaction with DNA and C terminal domain of alpha subunit. This interaction helps the rest of the RNA polymerase find the promoter region and activate transcription. 20 Class II activation Class II activators bind to sites that overlap the target promoter -35 region and, in most cases, activate transcription by making a direct interaction with domain 4 of the RNAP σ subunit The binding location of Class II activators cannot be varied because of constraints in the location of σ domain 4. In some cases, Class II activators make contacts with α CTD and other parts of the RNA polymerase (for example, αNTD). Class 1 can interact with another class 1 or class 2 Class 2 very close to -35 because they bind to sigma subunits to activate transcription. Complex promoters Complex promoters activated by independent contacts with two activators that recruit RNAP. In most cases the binding of one activator is dependent on the binding of the second activator. Class I + Class II Class I + Class I Good example of complex promoter is the melAB promoter, at which CRP (activator I) is unable to bind unless MelR (activator II) is already bound. 21 Activation by DNA conformational change The activator binds at, or near to, the promoter elements and realigns the –10 element and the –35 element so that the RNA polymerase holoenzyme can bind to the promoter. For example, in some promoters when the spacing between the –10 and –35 elements is not optimal for RNA polymerase binding, MerR-type activators bind to the ‘spacer’ sequence and twist the DNA to reorientate the -10 and -35 elements so that they can be bound by the RNA polymerase σ subunit. Activator protein binds to region between -10 and -35 and pushes them apart or brings them closer together and allows sigma subunits to recognise the correct region. Activation by repressor modulation In E. coli the nir promoter is dependent on activation by FNR that acts via a class II mechanism Activation by FNR is suppressed by the upstream-bound nucleoid-associated proteins, Fis and IHF. NarL binding (in response to the presence of nitrite or nitrate ions) displaces IHF from the IHF-I site, thereby relieving the suppression of FNR-dependent activation of the nir promoter. FNR is an activator, but it is inhibited by 2 repressors NarL binds in response to nitrite or nitrate ions 22 Anti-repression In some promoters binding of the secondary activator is required to counteract the inhibitory effects of a repressor, to allow the primary activator to function. Some activators are anti repressors Repression by steric hindrance The repressor- binding site overlaps core promoter elements and blocks recognition of the promoter by the RNA polymerase holoenzyme. For example the phage λ cI repressor when binding to the OR1 operator of the viral pR promoter, the LexA repressor when binding at the uvrA promoter, the B. subtilis phage φ29 protein p4 when binding at the viral A2b promoter, and LacI when binding to the O1 operator of the lac promoter REPRESSION Repressor binds between -10 and -35 and prevents RNA polymerase binding to the promoter. 23 Repression by DNA looping Repressors bind to distal sites and interact by looping, repressing the intervening promoter. For example, the gal promoter, which is repressed by GalR54 — multiple repressor molecules bind to promoter-distal sites, and repression is caused by DNA looping, which shuts off transcription initiation in the looped domain. 2 or more repressor proteins bind together and bend DNA in a way that prevents the RNA polymerase from reaching the -10 and -35 regions on the promoter. Repression by activator modulation The repressor binds to an activator and prevents the activator from functioning by blocking promoter recognition by the RNA polymerase holoenzyme. For example, repression by CytR is through direct interactions between CytR (the repressor) and CRP (the activator) that prevent CRP-dependent activation. Repressor binds to activator and changes its protein structure which prevents it binding to the alpha subunit so cannot bind to the promoter. 24 Cooperative repression Bacterial chromosomes are highly compacted by supercoiling with nucleoid proteins. The binding of these nucleoid proteins to DNA, results in folding of the bacterial chromosome and affect the distribution of RNA polymerase between promoters. For example, at the nir regulatory region, Fis, IHF and H-NS sequester the DNA into an ordered nucleoprotein complex, which consequently represses transcription. There are also many examples of promoters at which specific nucleoid-associated factors, such as Fis and IHF, have been recruited as activators of transcription initiation. Promoter escape regulation Promoter escape is the last stage of transcription initiation when RNA polymerase, having initiated de novo phosphodiester bond synthesis, must begin to relinquish its hold on promoter DNA and advance to downstream regions (DSRs) of the template. A general correlation has been found that the stronger the promoter DNA-polymerase interaction, the poorer the ability of RNA polymerase to escape the promoter. In gene regulation, promoter escape can be the rate-limiting step for transcription initiation. An increasing number of regulatory proteins are known to exert their control at this step. 25 Promoter escape regulation lacUV5 promoter is repressed by the LacI protein. Unlike other repressors that regulate transcription by occluding the binding of polymerase or interfering with isomerization, LacI and RNA polymerase can bind simultaneously at the promoter. When both proteins are bound, only abortive RNA is synthesized LacUV5 promoter LacI 5’ 3’ 3’ 5’ RNA RNA LacO +1 Terminator Polymerase Transcript Lac, I bind before +1 and stops transcription from proceeding beyond the promoter region Repression by roadblock During transcription, cellular RNA polymerases have to deal with numerous potential roadblocks imposed by various DNA binding proteins. Many such proteins partially or completely interrupt a single round of RNA chain elongation. For example, in Bacillus subtilis, CodY binding to the ybgE downstream site, as well as to downstream sites of the bcaP and yufN genes, contributes to transcription regulation by preventing elongation of transcription. Regulation during elongation process by roadblocks. Roadblocks recognise regions along the DNA transcript, and they prevent movement of the RNA polymerase. This leads to shorter RNA formation. May regulate which genes from the operon are expressed e.g., those close to the promoter CodY prevents elongation. 26 END OF VIDEO 2 START OF VIDEO 3 DNA methylation Adenine methylation can alter the interactions of regulatory proteins with DNA, either by a direct steric effect or by an indirect effect on DNA structure. In E.coli methylation of a GATC site(s) within the consensus RNA polymerase binding site inhibits (trpR and Tn10 transposase) or enhances (dnaA) transcription, by altering the interaction with the transcription apparatus. Phase variation of the outer membrane protein Ag43 in E. coli requires deoxyadenosine methylase (Dam) and OxyR. Methylation can alter regulatory proteins If GATC is not methylated the oxyR repressor can bind 27 Riboswitch mediate transcription One example of this is rli39, a vitamin B12 riboswitch (a). Depending on the vitamin B12 concentration, this riboswitch controls the transcription of an asRNA (aspocR) that overlaps pocR mRNA. In Clostridium acetobutylicum (b) cysteine conversion from methionine is produced by the proteins encoded in the ubiGmccBA operon, whose expression is controlled by two functional convergent promoters associated with transcriptional antitermination systems, a cysteine-specific T-box and S-box riboswitch, respectively. RNA is complementary to mRNA, and this is regulated by riboswitch Riboswitch: RNA can form a loop when there is a repeat in the sequence. This loop binds to small molecules. ** Research riboswitch Transcriptional interference Transcription interference in coliphage 186 arises when the elongating RNA polymerase complex that was initiated at the strong lytic promoter (pR) displaces the slow ‘sitting-duck’ RNA polymerase (RNAP) of the weak lysogenic promoter (pL), resulting in downregulation of the cI gene. Transcription interference in coliphage 186 arises when the elongating RNA promoter ** Sitting duck displaced 28 Transcription attenuation Binding of the asRNA RNAβ to the growing fatDCBA mRNA in Vibrio anguillarum induces transcription termination after the fatA gene, resulting in differential expression of the fatDCBA–angRT operon, with high expression of fatDCBA and low expression of angRT. Interfering RNA prevents continuation of elongation Regulation by DNA inversion Through the utilization of specific recombinases, a particular DNA sequence is inverted, resulting in an ON to OFF switch. Fimbrial adhesion by the type I fimbriae in E. coli undergoes site specific inversion to regulate the expression of fimA. The inversion is mediated by two recombinases, FimB and FimE, and regulatory proteins H-NS, Integration Host Factor (IHF) and Leucine responsive protein (LRP). The FimE recombinase has the capability to only invert the element and turn expression from on to off, while FimB can mediate the inversion in both directions. Promoter focuses on left side Proteins can remodel promoter to make different protein. E.g., regulation of fimA. Recombinase – cut and join back RNA 29 DNA supercoiling DNA in bacterial cells is maintained in a negatively supercoiled state. Supercoiling arises as a result of changes to the linking number of the relaxed double-stranded DNA molecule and is set and reset by the action of DNA topoisomerases. This process is subject to a multitude of influences that are usually summarized as environmental stress. Tightly coiled DNA is more difficult to transcribe The intrinsic terminator RNA polymerase U stretch Hairpin Intrinsic transcription termination signal appears in RNA as a hairpin followed by approximately eight uridines (U stretch) at the 3ʹ terminus. This signal leads to rapid dissociation of the ternary elongation complex (TEC) into RNA, DNA, and an RNA polymerase. Mechanism that regulates termination The quicker termination occurs, the faster RNA polymerase can be reused, therefore the greater the level of transcription 30 Rho-dependent termination RNA polymerase mRNA Rho Rho-dependent termination occurs by binding of Rho to ribosome-free mRNA. Rho's ATPase is activated by Rho-mRNA binding, and provides the energy for Rho translocation along the mRNA. When a polymerase pause site is encountered, the actual termination occurs, and the transcript is released by Rho's helicase activity. Rho = protein that forms a complex that recognises a region on the DNA and follows RNA polymerase until it catches up and terminates transcription. Mfd-dependent termination Mfd RNA polymerase mRNA Mfd is a highly conserved ATP-dependent DNA translocase in bacteria. It recognizes stalled RNA polymerase (RNAP) and removes it from DNA, leading to Mfd-dependent transcription termination. Mfd = highly conserved ATP dependent DNA translocase 31 Antitermination Antitermination is used as a control mechanism in phages to regulate the progression from one stage of gene expression to the next, and in bacteria to regulate expression of some operons. Antitermination occurs when RNAP reads through a terminator into the genes lying beyond. The terminators that are bypassed can therefore be defined as conditional terminators. Antitermination is not a general mechanism that can occur in all terminators, but is, rather, dependent on the recognition of specific sites in the nucleic acids. RNA doesn’t stop at the termination region and continues transcription. Antitermination For example, the N protein of bacteriophage λ mediates antitermination necessary to allow RNAP to read through the terminators located at the end of the immediate early genes in order to express the delayed early genes. The recognition site needed for antitermination by N, termed nut (for N utilization), lies upstream from the terminator at which the action is eventually accomplished. 32 Catabolite repression The presence of preferred carbon sources prevents the expression, and often also the activity, of catabolic systems that enable the use of secondary substrates. This regulation, called carbon catabolite repression (CCR), can be achieved by different regulatory mechanisms, including transcription activation and repression in different bacteria. Moreover, CCR regulates the expression of virulence factors in many pathogenic bacteria. cAMP-CAP regulated promoters 33 Inducible promoters Used in biotechnology Tryptophan regulated promoter When tryptophan is added it activates a repressor an inhibits transcription 34 Arabinose-regulated transcription In the presence of arabinose, arabinose binds to the arabinose binding pocket sites of AraC, causing AraC to dimerize at the I1 and I2operators. This allows access for CAP to bind to the CAP-binding sites, which in turn helps recruit RNA Polymerase to both PBAD and PC promoters and activates transcription. In the absence of arabinose, AraC dimerizes while bound to the O2 and I1 operator sites, looping the DNA. The looping prevents binding of CAP and RNA Polymerase, which normally activate the transcription of both PBAD and PC. Conclusion The DNA of prokaryotes is organized into a circular chromosome, supercoiled within the nucleoid region of the cell cytoplasm. Prokaryotic transcription regulatory mechanisms are diverse and may operate at different levels and stages in the transcription process. There are three types of regulatory molecules that can affect the expression of operons: repressors, activators, and inducers. Repressors bind to an operator region to block the action of RNA polymerase. Activators bind to the promoter to enhance the binding of RNA polymerase. Inducer molecules can increase transcription either by inactivating repressors or by activating activator proteins. 35 References Dale, Jeremy, and Simon F. Park. Molecular Genetics of Bacteria. 4th ed. Chichester: John Wiley & Sons, 2004. Pages 67-102 Snyder, Larry, and Wendy Champness. Molecular Genetics of Bacteria. 3rd ed. Washington, D.C: ASM Press, 2007. Pages 74-86 and 115-117 Ebright, Richard H, Finn Werner, and Xiaodong Zhang. “RNA Polymerase Reaches 60: Transcription Initiation, Elongation, Termination, and Regulation in Prokaryotes.” Journal of molecular biology 431.20 (2019): 3945–3946. Web. 36