L3. Polynucleotide Structure PDF
Document Details
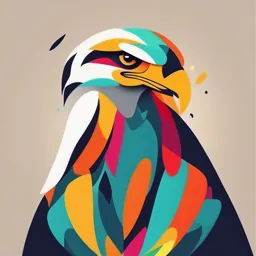
Uploaded by ExultantOliveTree
Dalhousie University
2024
Tags
Related
- A1.1 Notes Ch10,11,13,14 (1) Biology Notes PDF
- DNA Supercoiling Lecture Notes PDF
- Correction to Lecture 3, Polynucleotide Structure (2), PDF
- M 3.2 Biological Molecules - Lipids, Nucleic Acids PDF
- DNA Structure, Replication, and Manipulation - Genetics - 2024 PDF
- Molecular Basis of Inheritance Notes PDF
Summary
This document provides a detailed explanation of polynucleotide structure including DNA and RNA. It describes the components, formation, and important features such as the backbone, base pairs, and different conformations. The document also discusses the stabilizing and destabilizing forces of DNA structure.
Full Transcript
L3. Polynucleotide Structure Polynucleotide structure Nucleic acid: string of nucleotides (e.g. shown to the right) Is it a strand of DNA or RNA? (how to tell?) Check if it is deoxy, or not, at 2’ position! Phosphodiester bond: linkage between two nucleotides Linking the 3’ and 5’ positio...
L3. Polynucleotide Structure Polynucleotide structure Nucleic acid: string of nucleotides (e.g. shown to the right) Is it a strand of DNA or RNA? (how to tell?) Check if it is deoxy, or not, at 2’ position! Phosphodiester bond: linkage between two nucleotides Linking the 3’ and 5’ positions of adjacent nucleotide monomers formed during DNA and RNA synthesis The backbone is uniform : phosphate and sugar parts of the nucleotides Primary phosporyl groups are deprotonated at pH 7 à negatively charged). The bases are different: A, C, G, T in DNA; A, C, G, U in RNA. 5’ terminus (end) and 3’ terminus (end) of a DNA (or RNA): How to tell? Look for the 5’ and 3’ positions on the sugar part. DNA and RNA sequences are synthesized and read (decoded) in the 5’ to 3’ direction. Structure of double-stranded DNA DNA double helix shown on the right as the physical model developed by Watson and Crick (published in a paper in Nature in 1953) used also James Watson Francis Crick 3-changel's Watson and Crick used experimental evidence and nice clues from other researchers, especially Rosalind Franklin’s X-ray diffraction images X-ray diffraction images strongly indicated a double helical structure with phosphates on the ‘outside’ of the helix (see next slide) Base pairing biochemistry: Two strands are complementary to each other and were “anti-parallel”. This structure is required for DNA replication by templated synthesis 2 Who gathered the critical X-ray diffraction evidence needed to solve DNA structure? Rosalind Franklin and Maurice Wilkins were working at King’s College London ‘under’ John Randall in the 1950s Randall directed Franklin to investigate DNA fibres in 1950 using X-ray crystallography and assigned Raymond Gosling to be her assistant Wilkins had been working on this topic, but while he was away on holidays Randall told Frankly that Wilkins wouldn’t be working on Rosalind Franklin DNA anymore – but Wilkins was never informed and continued to work on it upon his return Rosalind Franklin developed new X-ray chamber setup with controlled humidity that generated better images of diffraction patterns (like Photo 51) and different experimental conditions could be tested In the end Franklin and Gosling’s data was critical to solving the structure of the double helix (the B-DNA structure) and was published in Nature in the same issue as Watson and Crick’s paper. X-ray crystallography But Franklin died in 1958 of ovarian cancer prior to the 1962 awarding diffraction pattern from of the Nobel prize which was awarded to Watson, Crick and Wilkins ‘Photo 51’ of B-DNA (Wilkins kept refining the structure for years after Franklin stopped produced in 1951 by working on it) Raymond Gosling Maurice Wilkins The above story is for your information only and you do not need to (supervised by Rosalind memorize it!!! Franklin) ‘Rules’ of the DNA double helix Base-pairing rules: A and T base pair, G and C base pair band stronger if more Hydrogen bonding in base pair: TGC 2 hydrogen bonds in A/T base pair conten ↑ t in 3 hydrogen bonds in G/C base pair. ( 10 close. 8 A together The two strands must be anti-parallel: Strand on the left has 5’ at the top, 3’ at bottom, the other strand must have 3’ at top, 5’ at bottom. Occurs in double-stranded DNA, double-stranded RNA (e.g. rRNA, some viral RNA), and DNA/RNA Nearly uniform width of hybrid (one strand each) double-stranded DNA (11.1 – 10.8 angstrom) (purine/pyrimidine pairing) 3 Exercises Base-pairing rules for DNA A and T base pair, G and C base pair DNA strand: 5’ CACTGC 3’ Write its complementary strand. DNA strand: 3’ GTGACG 5’ during Number of H-bonds? For DNA/RNA hybrid or double- , transcription] 4 G/C pairs and 2 A/Tpairs = 4x3 + 2x2 = 16 stranded RNA G and C base pair (DNA or RNA) A (DNA or RNA) and T (DNA) base pair A (DNA or RNA) & U (RNA) base pair DNA stand: 5’ CACTGC 3’ Write its complementary RNA strand. RNA strand: 3’ GUGACG 5’ No. of H-bonds? Note: For the A/U base pair there are two Also 16! hydrogen bonds (just like A/T) Double-stranded DNA structure Sugar- Base pairs (yellow) (a) ribbon representation phosphate (b) stick representation backbones (c) space-filling representation (blue) betweena a Major grooves and minor grooves (Between strands) Major groove exposes bases more (1 helical Major groove is often where the turn) DNA sequence can be recognized by proteins 10.5 bp (Between helical turns) Yea (e.g. by transcription factors). Each helical turn has 10.5 base pairs e.g., number of helical turns in a 1000 bp DNA: 1000/10.5 = 95.2 (in structurally relaxed DNA) 5 Stabilizing forces of DNA structure: Sugar-phosphate backbones (blue) Base pairs hydrogen bonds in base pairs (yellow) base stacking interactions àhydrophobic and electrostatic 10.5 bp forces iS + Pi orbita favorable : 6 Sugar-phosphate backbones (blue) Base pairs (yellow) Stabilizing forces of DNA structure: hydrogen bonds in base pairs base stacking (hydrophobic and electrostatic forces) Destabilizing forces: electrostatic repulsion between the two strands 10.5 bp Why? Both strands are negatively charged. (phosphate groups in the backbone) 6 Denaturing DNA: heat to 95 Heating disrupts base stacking à destabilizes double stranded structure I strong base ( OR Alkaline (OH-) conditions disrupts the hydrogen bonding b/w basepairs on complementary strands à destabilizes double stranded structure Three structural forms of DNA: A form, B form, Z form B form (B-DNA) is the common form i.e it’s the structure of most DNA, under normal conditions) can Turn B-DNA.... A-DNA by )... C A form (A-DNA) is formed in: (less common than B-DNA) high salt and low humidity, in DNA/RNA hybrid, Double stranded (ds) RNA at active site of DNA polymerase. Z form (Z-DNA): Left-handed helix Formed by sequences of alternating purines and pyrimidines (e.g. GCGCGCGC…) Z-DNA binding proteins in cells exist. These forms of DNA differ in their preferred confirmations of the nucleotides! (like a Sugar pucker tube) Cribbon) Syn vs. Anti Sugar pucker conformations Position the sugar pentose on a level plane: (a) C3’-endo: C at the 3’ position protrudes to the upside (same side as the base) (b) C2’-endo: C at the 2’ position protrudes to the upside (same side as the base) Full atomic structure Simplified structure for easier view Sugar pucker conformation affects the distance between neighboring phosphate groups in DNA. Glycosyl bond conformation: syn vs. anti On the nucleoside below draw a dividing line on the glycosyl bond and through the base: Syn-conformation: larger part of the base and the sugar are on same side of the dividing line. -spatially more crowded (structurally unfavorable), found only with purines in Z DNA. Anti-conformation: most the base is on the opposite side of the dividing line from the sugar. -spatially less crowded, therefore favored in B DNA (the common form of DNA) on same of base is if most side as sugar , syn. Note that Glycosyl Glycosyl pyrimidines Glycosyl bond bond (C, T and U) bond always adopt the anti conformation Three forms of DNA: B-DNA, A-DNA, and Z-DNA. Differ in helix sense (right vs. left-handed helix), sugar pucker conformation, and glycosyl bond conformation. B-DNA is the normal (common) form: Ø It is a right-handed helix, its glycosyl bond conformation is anti and its sugar pucker is C-2’ endo : In the table shown above, you need to memorize only points underlined in red.