DNA Replication: Steps of Formation PDF
Document Details
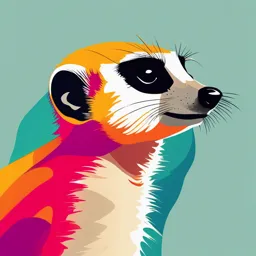
Uploaded by SpiritualHonor
College of Medicine
Tags
Related
- Lecture 2.1 Molecular and Cellular Biology (DNA Replication) PDF
- Molecular Biology Instant Notes PDF
- Molecular Biology Lv2 DNA Replication Lecture 4 PDF
- Cell Cycle & DNA Replication Lecture 5 PDF
- Fundamentals in Biology 1: From Molecules to the Biochemistry of Cells PDF
- LF130 Cellular and Molecular Biology Lecture 9, 2024 PDF
Summary
This document details the steps of DNA replication in eukaryotes, including the semiconservative nature of the process, the role of multiple origins and replication forks, and the involvement of various enzymes like DNA helicases, topoisomerases, and polymerases. It explains the concept of leading and lagging strands and Okazaki fragments.
Full Transcript
10 DNA REPLICATION I : STEPS OF FORMATION ILOs By the end of this lecture, students will be able to 1. Interpret the importance of DNA replication rules in preserving human body 2. Identify the requirements of DNA replication 3. Discuss process of DNA replication 4....
10 DNA REPLICATION I : STEPS OF FORMATION ILOs By the end of this lecture, students will be able to 1. Interpret the importance of DNA replication rules in preserving human body 2. Identify the requirements of DNA replication 3. Discuss process of DNA replication 4. Correlate the benefits of altering specific steps of DNA replication by drugs to control certain disease states What is meant by replication? Replication is the process whereby DNA makes a copy on itself each time the cell divides, in order to supply daughter cells with a typical copy of DNA present in parent cells. In Eukaryotes, DNA replication occurs in the nucleus during S-phase of interphase (interval between two cell divisions).(Refer to cell cycle lecture) Rules of DNA replication in eukaryotes 1. DNA replication is semiconservative: Each DNA strand serves as a template for synthesis of a new strand producing two double-stranded DNA molecules, each with one new strand and one old strand. 2. Replication begins at multiple origins and usually proceeds bidirectionally. Having multiple origins of replication provides a mechanism for rapidly replicating the great length of eukaryotic DNA molecules. 3. Replication exhibits polarity: DNA synthesis proceeds in a 5` 3` direction and is semi- discontinuous (What do you think this means?) 4. Replication is very accurate: replication proceeds with an extraordinary degree of fidelity. Identification of origins of Replication A human chromosome is tens to hundreds of millions of base pairs long. Logistically, this means that replication would be far too slow if it proceeded from only a single origin. In reality, replication starts at a number of different sites, termed origins of replication, spaced 30,000 to 300,000 base pairs apart. From each origin, a replication fork (the point at which DNA strands unwind) is formed, and proceeds in either direction until they meet, or until they reach the end of their chromosome. The number of active origins of replication is variable. At times when rapid 1 duplication of DNA is required (e.g., cell division of the early embryo), more origins of replication may be active. To identify the points in DNA strands where replication should start, certain proteins called origin recognition complex (ORC)proteins, scan the DNA strands for regions rich in Adenine and thymine complementary bases (Can you deduce the reason?). Once found, the proteins bind to those regions, tagging them as origins of replication. (Figure 1) Steps of DNA replication Step 1: DNA Unwinding To be copied, the DNA double helix must first be unwound and the strands separated by breaking the hydrogen bonds between the nitrogenous bases. The process is catalyzed by enzymes called DNA helicases (Molecular scissors). (Figure 1) To prevent unwound DNA double strands from rewinding back, certain proteins called single-stranded DNA binding proteins, bind to unwound DNA strands. Local unwinding can cause overwinding, or supercoiling, of DNA downstream, the thing that can prevent DNA unwinding downstream. This supercoiling is prevented by proteins called DNA topoisomerases, which create breaks between nucleotides, allow the DNA to uncoil, and then re-anneal the broken nucleotides. ⮚ Topoisomerase I makes single-stranded breaks. ⮚ Topoisomerase II makes double-stranded breaks. Unwinding of DNA, causes the formation of a structure called replication fork As a rule, DNA replication should proceed in the 5’>>3’ direction on the newly synthesized strand, and it should proceed AWAY from the origin of replication. Figure 1: Initiation of replication 2 Step 2: RNA Primer Synthesis DNA polymerases, the enzymes responsible for replication, cannot initiate synthesis of a new strand by linking free nucleotides together. In addition to a template, DNA polymerase requires a primer, a short piece of RNA with a free 3' hydroxyl, which the DNA polymerase can elongate. RNA primers are synthesized by an enzyme called RNA primase, which is a component of a DNA polymerase- α protein complex. The RNA primase synthesizes a short RNA primer (about 8-12 bp long) and the DNA polymerase-δ and polymerase-ε extend this primer by adding deoxynucleotides. Step 3: DNA Polymerization (Figure 2) The new daughter DNA strand is synthesized by adding nucleotides to the RNA primer. Nucleotides are joined together by phosphodiester bonds between the 3' hydroxyl group of the growing strand and the 5' phosphate of the next nucleotide. DNA polymerases are enzymes responsible for polymerization of newly added nucleotides. Newly added nucleotides are in the triphosphate form dGTP, dCTP, dATP, and dTTP. Energy for the formation of the phosphodiester bond comes from breaking the high- energy phosphate bonds on the nucleotide triphosphate. e.g. ATP >>>>>>AMP+ PPi, GTP>>>>>>GMP+ PPi…. etc Each newly added nucleotide should be complementary (i.e., G-C or A-T) to the corresponding nucleotide in the parental strand (template strand). GOLDEN RULE: Replication of new strands proceeds in the 5' >> 3' away from the origin of replication. On one strand, polymerization proceeds continuously away from the origin of replication. This is the leading strand. However, on the other strand, called the lagging strand, if replication is to proceed in the 5' > 3' direction, this means it will proceed towards the origin of replication which is against the rule. To overcome this, the daughter strand is synthesized in a discontinuous fashion in the form of short fragments of DNA that are approximately 150 base pairs long. These are called Okazaki fragments. Each fragment is synthesized in the 5’>3’ direction (i.e towards the origin of replication), however, as each new fragment is synthesized, the body perceives as if the synthesis of the new strand is proceeding away from the origin of replication. Okazaki fragments are later joined together through the action of DNA ligase. (Figure 2) Types of eukaryotic DNA polymerases DNA polymerase α: Priming and initial synthesis DNA polymerase β: DNA repair (refer to DNA damage and repair lecture) 3 DNA polymerase y: mtDNA replication DNA polymerase δ: Lagging strand synthesis, proof reading activity(Refer to DNA replication II lecture) DNA polymerase ε: Leading strand synthesis, proof reading activity Figure 2: Elongation of newly synthesized DNA strand and formation of leading and lagging strands Step 4: Degrading RNA Primers The RNA primers are removed by a 5' >>> 3' exonuclease. The resulting gap is filled in by a DNA polymerase. (Figure 3) Step 5: Ligation Ligation is the creation of phosphodiester bonds between individual DNA fragments so that the whole strand becomes one continuous strand. This is catalyzed by an enzyme called DNA ligase. (Figure 3) This process continues until the entire strand has been replicated to form two identical daughter strands. 4 Figure 3.Degradation of primers and ligation of strands Clinical implications: Different steps of DNA replication are target of action of many anticancer and antimicrobial agents: 1. Incorporate into DNA to interfere with chain elongation and induce defective ligation of fragments of newly synthesized DNA (especially in rapidly proliferating cells) in cancer cells by using anticancer; or in viruses by using antiviral; acyclovir against Herpes simplex virus. 2. Inhibit topoisomerase enzyme in cancer cells, by using the anticancer or in bacterial cells using the antibacterial agents; (Quinolone antibiotics). 5 9 NUCLEOTIDES & NUCLEIC ACIDS ILOs By the end of this lecture, students will be able to 1. Describe types & structure of nucleotides and nucleic acids 2. Explain function of nucleotides and nucleic acids 3. Interpret why the structure and organization of nucleic acids best fits their function What are nucleic acids? Nucleic acids are the most important of all biomolecules. They function to create and encode and then store information in the nucleus of every living cell of every life-form organism on earth. They represent the hard-disk of our body. The term nucleic acid is the overall name for Deoxyribonucleic acid (DNA) and Ribonucleic acid (RNA). They are composed of nucleotides, which are the monomers made of three components: 5-carbon sugar (pentose): If the sugar is ribose, the nucleic acid is termed RNA; if the sugar is deoxyribose, the nucleic acid is termed DNA. Phosphoric acid: Phosphoric acid is attached to OH group at 3′ carbon of deoxyribose sugar in one nucleotide and OH group at 5′ carbon of deoxyribose sugar in adjacent nucleotide. Nitrogenous bases: They are organic molecule with a nitrogen atom that has the chemical properties of a base. The main biological function of a nitrogenous base is to bond nucleic acids together. Nitrogenous bases are typically classified as the derivatives of two parent compounds: pyrimidines and purines (Figure1) Pyrimidines are formed of 1 cyclic ring. These include: Cytosine, thymine, uracil Purines are formed of 2 heterogeneous rings. These include: Adenine, Guanine Purines are present in both DNA and RNA. As for pyrimidines, the distribution is different. DNA contains cytosine and thymine while RNA contains cytosine and uracil. Thus, in summary, DNA and RNA are polymers of nucleotides. A nucleotide is composed of a nitrogenous base bound to a pentose sugar (both together form what is called NUCLEOSIDE), in addition to phosphate group. 1 Figure 1: Nitrogenous bases present in nucleic acids The number of phosphate groups can be either one group (nucleoside monophosphate), or 2 groups (nucleoside diphosphate), or 3 groups (nucleoside triphosphate) Formation of nucleotide polymers (Figure 2) Nucleotides are attached to each other through a 3’-5’ phosphodiester bond. This bond links carbon number 3 of the sugar and carbon number 5 in the sugar of the next nucleotide. Carbon number 5 links to this bond through sharing by its phosphate group. After polymerization, the formed chain will have a 3’ end (in which carbon number 3 in the sugar is free and not involved in any bond) and a 5’ end (in which carbon number 5 in the phosphate group of the sugar is free and not involved in any bond). Figure 2: Formation of Deoxyribonucleic acid (DNA) phosphodiester bond to link nucleotides to form nucleic acids To form DNA, the 2 strands of deoxyribonucleotides wind around each other in a clockwise direction, forming a structure called double helix. This structure is linear. In the double helix, the sugar and phosphates are located to the exterior, forming the backbone, while the nitrogenous bases are located to the interior for better protection from degradation. DNA is located within the nucleus of cell. It is associated with basic proteins and forms chromosomes. This is called nuclear DNA. 2 DNA is also located within mitochondrial matrix (mitochondrial DNA (mtDNA) and takes a circular double stranded form. Properties of DNA strands 1- Polarity Each DNA strand has polarity, with a 3′ End (3 prime) which is the terminal of DNA strand where C3 of deoxyribose sugar is not linked and is free, and 5′ End (5 prime) which is the end of DNA strand where C5 of deoxyribose sugar is not linked and is free. Therefore, the two DNA strands show polarity, where each strand shows runs either in 3′–5′ direction or 5′–3′ direction. 2- Anti-polarity (antiparallel strands) In DNA helix, two strands run in antiparallel direction; one strand runs from 3’ -5’ direction while the other runs from 5’-3’ direction. 3- Base Pairing Base pair signifies two nitrogenous bases held together through hydrogen bonding. Each pair of base consists of purine in one strand and pyrimidine in another strand. Figure 3: base pairing and antipolarity in DNA Particular purine always pairs with particular pyrimidine. This is called Complementary base pairing, where adenine pairs with thymine with double hydrogen bond and guanine pairs with cytosine with tripe hydrogen bond (A=T, G≡C) Eukaryotic DNA organization To fit the 2 meter long DNA in the nucleus, it is wrapped around the basic proteins called histones (5 types designated H1, H2A, H2B, H3, and H4) that, along with ions such as Mg 2+ , help neutralize the negatively charged DNA phosphate groups. The N-terminal ends of these histones can be acetylated, methylated, or phosphorylated. These reversible covalent modifications influence how tightly the histones bind to the DNA, thereby affecting the expression of specific genes. Histone modification is an example of epigenetics, or heritable changes in gene expression caused without alteration of the nucleotide sequence.(Refer to regulation of gene expression lecture) 3 Functions of DNA 1. DNA carries the genetic material of the cell and is responsible for transmission of this material to newly divided cells through making exact copies on itself. (Refer to DNA replication lecture) 2. DNA is responsible for protein synthesis through the process of gene expression. (Refer to transcription and translation lectures) Ribonucleic acid (RNA): RNA is present mainly in the cytoplasm of the cell and has three types: 1. Messenger RNA (mRNA): It constitutes 5% of all RNA. It is synthesized in the nucleus by DNA and then sent to the ribosomes in the cytoplasm. The mRNA carries a “coded” message from DNA in the nucleus, where it is synthesized, to the ribosomes in the cytoplasm, where it is going to direct the synthesis of a specific protein. The letters of the message are the nitrogenous bases, the sequence of which is complementary to that of the DNA from which it was copied. mRNA contains adenine, guanine, cytosine and URACIL. Each 3 successive bases in the mRNA are called a “codon” because they code for a specific amino acid. These codons are responsible for arranging the amino acids in proper order in the polypeptide chain to be synthesized. The process of synthesis of mRNA in the nucleus under the directions of DNA is called “transcription”. (Refer to transcription lecture) 2. Transfer RNA (tRNA): (Figure 5) It constitutes 15% of all RNA. It is present in the cytoplasm and may be also known as soluble RNA (sRNA).It is composed of 74 to 95 nucleotides. It has a hairpin structure and is stabilized by hydrogen bonds. tRNA molecules function as carriers for the amino acids and transfer them to the machinery site of protein synthesis in the cell ( Ribosome ). There is, at least, a specific tRNA for each amino acid. This means that there are, at least, 20 different tRNA molecules in every cell, each of which is specialized to carry one of the 20 different amino acids required for the process of protein synthesis. There is an amino acid binding site on the 3’ terminal. In addition there is an anticodon site for recognition of codons on mRNA. 3. Ribosomal RNA (rRNA): It constitutes 80% of total RNA. It is present in the ribosomes of the cytoplasm. 4 The ribosome is a cytoplasmic nucleoprotein structure which acts as the machinery for the synthesis of proteins. On the ribosomes, the mRNA and tRNA molecules interact to translate a specific protein. (Refer to the protein machinery lecture). Figure 5: Transfer RNA 5