PHYL 2041 Lecture 10: Auditory System PDF
Document Details
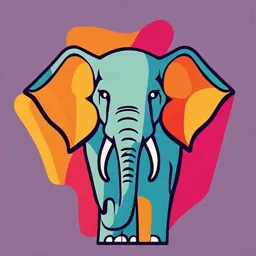
Uploaded by AwedNephrite
Dalhousie University
Dr. Stefan Krueger
Tags
Summary
This document is a lecture on the auditory system from Dalhousie University. It covers topics such as sound transmission, transduction, and central processing in the auditory system. It contains diagrams, explaining details in a way that is ideal for teaching and learning.
Full Transcript
PHYL 2041 -- LECTURE 10 Auditory System Dr. Stefan Krueger Dept. of Physiology & Biophysics [email protected] Auditory System: Topics 10.1. Sound & Its Transmission in The External and Middle Ear 10.2. Sound Transduction in...
PHYL 2041 -- LECTURE 10 Auditory System Dr. Stefan Krueger Dept. of Physiology & Biophysics [email protected] Auditory System: Topics 10.1. Sound & Its Transmission in The External and Middle Ear 10.2. Sound Transduction in The Inner Ear 10.3. Central Processing of Auditory Information Sound & Its Transmission in The External and Middle Ear 10.1.1. Sound: Nature & Attributes 10.1.2. Audible Range 10.1.3. Pure Tones and Natural Sounds 10.1.4. Role of The External Ear 10.1.5. Function of The Middle Ear 10.1.6. Regulation of Sound Transmission Ef ciency Back to main index fi 10.1.1. Sound: Nature & Attributes Sound = Waves of compressed and rari ed air and can be represented by a sinusoidal function. Generated by vibrating object, sound waves propagate in three dimensions The wave amplitude, i.e. the magnitude of pressure change, determines the loudness. The frequency, i.e. the the speed with which pressure changes oscillate, determines the pitch. Two waves of same pitch can differ in their phase, i.e. the exact timing of their wave peaks and troughs. Phase differences of sounds arriving at either ear are important for sound localization. Next slide fi 10.1.2. Audible Range The audible range of frequencies for humans is between 20 and 20,000 Hz (1 Hertz = 1 wave cycle per second). The detection threshold (minimal sound pressure necessary to detect sound) depends on its frequency. Audible range changes with age Next slide 10.1.3. Pure Tones and Natural Sounds Most naturally occurring sounds are not pure tones but complex waves composed of many pure tones. Sounds can be decomposed mathematically into constituent pure tones (Fourier transformation). Auditory system: Ear decomposes natural sounds into constituent pure tones. Cortex analyzes decomposed information to extract information. Back to index 10.1.4. Role of The External Ear Sound waves funnelled by pinna and External ear external auditory canal onto tympanic membrane. Asymmetrically shaped pinna re ects high-frequency sounds based on elevation of sound source and contributes to sound localization along the vertical axis. External auditory canal ampli es sound pressure 30- to 100-fold. Sound waves cause tympanic membrane to vibrate. Vibration frequency is Pinna External Tympanic auditory canal membrane dependent on sound pitch, vibration amplitude dependent on loudness. Adapted from: Derrickson Human Physiology 2nd Ed. Wiley. Back to index fi fl 10.1.5. Function of The Middle Ear Middle ear Sound transmission in middle ear: Vibration of the tympanic membrane causes Ossicles movement of auditory ossicles: Malleus (connected Incus to tympanic membrane), incus, stapes (connected Malleus Stapes to oval window). Stapes transmits vibrations to oval window. Vibrating oval window elicits pressure waves in uid- lled inner ear Purpose Conversion of airborne pressure waves into pressure waves within the uid- lled inner ear complicated by larger resistance of uid to movement compared to air. Pressure boosted ~200-fold by: Focusing force Tympanic Middle ear Oval window impinging on large tympanic membrane onto much membrane cavity (below stapes) smaller oval window; lever action of ossicles. Back to index fl fi fl fi fl 10.1.6. Regulation of Sound Transmission Ef ciency Inner ear exquisitely prone to damage by loud noises To protect ears from damage, the ef ciency of sound transmission in the middle ear can be decreased Flexion of the tensor tympani muscle attenuates vibration of tympanic membrane, exion of stapedius muscle reduces movement of stapes => reduction in sound transmission Acoustic re ex: Flexion of muscles is auto- matically triggered by loud noises via cranial nerves V (Tensor tympani) and VII (Stapedius) Back to index fi fl fl fi Sound Transduction in The Inner Ear 10.2.1. Inner Ear Structure: Cochlear Ducts 10.2.2. Inner Ear Structure: Organ of Corti 10.2.3. Sound Transmission: Basilar Membrane 10.2.4 Sound Transmission: Organ of Corti 10.2.5. Sound Transduction: Receptor Potentials 10.2.6. Sound Transduction: Molecular Mechanisms 10.2.7. Functional Differences Between Inner & Outer Hair Cells 10.2.8. Hearing Loss: Causes, Diagnosis, Treatment Back to main index 10.2.1. Inner Ear Structure: Cochlear Ducts Cochlea (lat. “snail”) is hollow tube wrapped up into spiral. Walls made of bone. Cochlea Cochlea partitioned into three uid- lled chan- (uncoiled) nels: Scala vestibuli and scala tympani on either Sound waves side of the partition, scala media within. Two membrane-covered holes at base of Oval cochlea: Oval window connects middle ear Scala window vestibuli to scala vestibuli, round window connects middle ear to scala tympani. Scala media Tip of cochlea (apex) lacks partition. Organ of Corti Scala Scala vestibuli and scala tympani lled with tympani Basilar perilymph, scala media with endolymph. membrane Auditory nerve Round Scala tympani and scala media separated by window basilar membrane. On top of basilar membrane within scala media is Organ of Corti containing sensory receptors. Next slide fl fi fi 10.2.2. Inner Ear Structure: Organ of Corti Organ of Corti contains auditory receptor cells: Hair cells. Hair cells have stereocilia (apical processes) which contact the tectorial membrane. Tectorial membrane and basilar membrane connected to modiolus, the bony core of Tectorial membrane cochlea. Stereocilia Modiolus Hair cells divided into single row of inner hair cells and three rows of outer hair cells. Hair cells form synapses onto afferents of primary sensory neurons Axons of these sensory neurons form A erents the cochlear portion of vestibulocochlear Basilar Outer Inner of sensory neurons nerve (cranial nerve VIII). membrane hair cell hair cell Back to index ff 10.2.3. Sound Transmission: Basilar Membrane Vibration of oval window due to movement of stapes Low-pitched sound create pressure waves in perilymph in scala vestibuli. Basilar Pressure waves propagate in scala vestibuli and membrane into scala tympani to round window which also moves with pressure waves. Apex wide Pressure waves cause displacement of basilar membrane. Base narrow and sti and oppy Basilar membrane properties change over its length: High-pitched Narrow and stiff at base, wide and oppy at apex. sound Region of the basilar membrane that is displaced most is dependent on sound pitch. High-pitched sounds create movement at stiff base end, low-pitched sounds at oppy apex end of basilar membrane. Tonotopy = topographical mapping of sound frequency onto basilar membrane. Next slide fl f fl fl 10.2.4. Sound Transmission: Organ of Corti Tectorial membrane Stereocilia Structure supporting hair cells moves with the vibrating basilar membrane. In contrast, tectorial membrane is immobile (held in place by bony modiolus). Hair cells Upward de ection of basilar membrane causes Stereocilia bent stereocilia of hair cells to be bent away from the center of the cochlea. Conversely, downward de ection causes stereocilia to be bent in the other direction. Basilar membrane de ected upward => Vibration of the basilar membrane are Stereocilia bent to other direction translated into back-and-forth motion of hair cell stereocilia Basilar membrane de ected downward Back to index fl fl fl fl 10.2.5. Sound Transduction: Receptor Potentials De ection of hair cell stereocilia Hair cells generates receptor potentials: Bundle of Bending of cilia in the direction of stereocilia largest stereocilium causes membrane Glass pipette depolarization to de ect cilia Depolarization increases with increased de ection of cilia Bending of cilia in the other direction causes membrane hyperpolarization Receptor potentials are very fast, can follow pure tones up to 1000 Hz (= 1000 oscillations per second!) Note: No action potentials generated in hair cells (do not have voltage-gated sodium channels) Back to index fl fl fl 10.2.6. Sound Transduction: Molecular mechanisms Stereocilia contain mechanically gated cation Endolymph (high [K+]) Stereocilia channels connected by tip links. Tip links Bending of stereocilia opens or closes cation Mechanically gated channels, modulating in ux of K+ (endolymph cation channel has high potassium concentration), depolarizing the membrane. Membrane depolarization causes opening of voltage-gated calcium channels. Membrane Depolarization Calcium in ux triggers the release of the Voltage-gated neurotransmitter glutamate at synapses with Ca2+ channel sensory afferents. Voltage-gated K+ channel Glutamate binds to ionotropic receptors on Synaptic vesicle sensory afferents, causing the depolarization of Glutamate sensory neurons. (neuro- transmitter) Action potential generation in sensory neurons. Perilymph (Low [K+]) Back to index fl fl 10.2.7. Functional Differences Between Inner & Outer Hair Cells Inner hair cells: Outer Receive 95% of the innervation from hair cell sensory neurons provide bulk of information relayed to Inner hair cell auditory cortex E erents Outer hair cells: Sensory neurons contact many outer hair cells A erents A erents Receive efferents from the superior olivary Spiral ganglion neurons complex in the brainstem Can rapidly change cell length in response to Auditory nerve depolarization and amplify movements of the basilar membrane in response to low-intensity sound stimuli: Cochlear ampli er E erents from superior olive Vibrations generated by outer hair cells can generate sounds: Otoacoustic emissions Back to index ff ff ff ff fi 10.2.8. Hearing Loss Causes: Conductive hearing loss involves external and middle ear. Can be due to ear canal occlusion, tympanic membrane rupture, arthritic ossi cation of middle ear bones. Sensorineural hearing loss is due to an inner ear defect (e.g. hair cell death). Congenital (defects in genes essential for auditory transduction or hair cell survival) or acquired (acoustic trauma, ototoxic drugs, presbycusis). Gradual hearing loss tends to affect high-frequency range rst. Diagnosis: Conductive vs. sensorineural hearing loss: Testing of bone conduction of sounds (unaffected by conductive hearing loss): Weber or Rinne tests. Degree of hearing loss: Detection thresholds for pure tones. In patients unable to report measurement of otoacoustic emissions Treatment: External hearing aids, bone anchored hearing aids & cochlear implants Back to index fi fi Central Processing of Auditory Information 10.3.1. The Auditory Pathway 10.3.2. Encoding of Sound Intensity in The Auditory Nerve 10.3.3. Encoding of Sound Frequency in The Auditory Nerve 10.3.4. Sound Localization 19.3.5. Response Properies of Neurons in the Auditory Cortex Back to main index 10.3.1. The Auditory Pathway Bipolar neurons forming auditory nerve have soma in spiral ganglion; afferents innervate cochlear hair cells, efferents project ipsilaterally to cochlear nuclei. Neurons in ventral cochlear nucleus project bilaterally to the superior olive (important in sound localization). Neurons in dorsal cochlear nuclei and su- perior olive project to the inferior colliculus. Neurons in the inferior colliculus project to the medial geniculate nucleus in the thalamus. Neurons in the medial geniculate nucleus projext to the primary auditory cortex. Back to index 10.3.2. Encoding of Sound Intensity in The Auditory Nerve Louder sound Amplitude of basilar membrane Greater section of the basilar movements larger membrane activated Hair cell stereocilia Stereocilia of more de ected more hair cells de ected Hair cells depolarize more and More hair cells depolarize and release more neurotransmitter release neurotransmitter Sensory neurons receiving synaptic input from hair cells More sensory neurons re re action potentials with action potentials Adapted from: Rose et al. (1971) J Neurophysiol 34, 685-699 higher frequency Back to index fi fl fl fi 10.3.3. Encoding of Sound Frequency in The Auditory Nerve (1) Each spiral ganglion neuron inner- Spiral ganglion neurons Anterior vates only one inner hair cell. Apex of basilar membrane Consequence: Frequency sensitivity of neuron determined by location of inner- Cochlear vated hair cell on basilar membrane; Posterior Nucleus captured by its by its characteristic Base of basilar membrane frequency and tuning curve. Neurons innervating base of basilar Tuning curves of neurons innervating membrane are tuned towards high- di erent regions of the basilar membrane frequency sounds, neurons innervating apex sensitive to low-frequency sounds. Efferents of spiral ganglion neurons to cochlear nucleus are ordered => Cochlear nuclei also have tonotopic organization. Back to index ff 10.3.3. Encoding of Sound Frequency in The Auditory Nerve (2) Neurons innervating apex of basilar membrane Neurons tuned to low frequencies: Exhibit phase locking have lower ability to discriminate differences in sound frequency based on hair cell location alone. Low frequency-tuned neurons ( 5kHz) too fast to allow for phase locking: Frequency information only via tonotopy. In intermediate range, neurons do not re on Neurons tuned to high frequencies: No phase locking every cycle, but are still phase locked. Aggregate activity of a group of neurons with same frequency dependence still carries exact information on frequency. Back to index fi fi 10.3.4. Sound Localization Occurs in the superior olive ( rst center in auditory Sound frequency ≤ 2kHz: Comparison of timing pathway to receive input from both ears). Two different Sound mechanisms for localization in horizontal plane: source Low-frequency sounds: Comparison of timing of input coming from either ear. Phase of sound coming from ear more distant to sound source is slightly delayed. High-frequency sounds: Comparison of intensity of input coming from either ear. Head casts sound shadow. Input coming from ear more distant to sound source is slightly less intense Sound frequency > 2kHz: Comparison of intensity Information about stimulus delay and intensity differen- ces is relayed to inferior colliculus, which generates a topographical representation of auditory space by integrating input from the superior olive (horizontal information) and the dorsal cochlear nucleus (vertical information) Back to index fi 10.3.5. Auditory Cortex Located in temporal lobe of cortex, adjacent to central sulcus. Subdivisions: Primary auditory cortex (A1) receives projections from the medial geniculate nucleus (MGN) of thalamus. Tonotopic map (topo- graphic representation of cochlea). Patch-like distribution of neurons excited by input from both ears (EE) or excited by input from one ear and inhibited by other (EI). Belt and parabelt. Surround A1 and receive projections from MGN and A1. Contain many neurons responsive to spectral combinations of sounds which often need to be in precise temporal order for maximal activation. Some of these “combination-sensitive” neurons already found in A1, MGN. Back to index