Lecture 1: The Founding of Modern Science PDF
Document Details
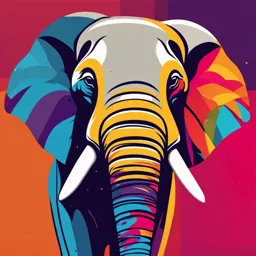
Uploaded by BlamelessRhodonite
NUS
Tags
Summary
This document is a lecture on the founding of modern science. It covers the scientific method, highlighting the roles of scientific observations in this process, discussing the importance of anomalous phenomena, and looking at the Industrial Revolution. It emphasizes the crucial nature of testing explanations within science.
Full Transcript
Lecture 1 HSI1000 1 The Founding of Modern Science Intended Learning Outcomes for Lecture 01 You should be able to do the following after this lecture. (1) Describe what is science and explain the scientific method “in a nutshell”, illustrating your explanat...
Lecture 1 HSI1000 1 The Founding of Modern Science Intended Learning Outcomes for Lecture 01 You should be able to do the following after this lecture. (1) Describe what is science and explain the scientific method “in a nutshell”, illustrating your explanation with a straightforward example. (2) Describe the roles scientific observations play in the scientific method. (3) Explain what are the main concerns that should be addressed when making scientific observations. (4) Explain why anomalous phenomena are important for science, illustrating your explanation with some examples from the scientific revolution. (5) In the context of the scientific revolution, discuss the difference between an evidence-based understanding of the natural world versus one based on authority. (6) Discuss the steam engine’s contribution to the Industrial Revolution and its impact on population growth in industrialized nations. 1.1 What is Science? Hi all, welcome to the first video in this series. This lecture, which is made up of several videos, is about what science is and is a cut-down bare-bones explanation of the scientific method “in a nutshell” – which we will see illustrated with a few examples. We will take a closer look at the first step in the scientific method (again illustrated with an example) and then briefly review the founding of modern science and what one could say was a direct consequence of that – the Industrial Revolution, and it’s here we’ll see the beginning of mankind’s dependence on fossil fuels. I hope you have had a look through the intended learning outcomes for this lecture. They are listed right before this video, so let’s get straight into addressing our first learning outcome, which is to answer the question: “What is science actually?” I bet most people think of science in terms of “subjects”, like Chemistry, Physics, Biology, Medicine, and Pharmacy, just to name a few. But is this actually science? Knowledge in textbooks? Where did this knowledge come from anyway? Most people would say that the facts, ideas, and concepts in science textbooks is true to the best of our knowledge, but how do we know it’s true? In fact, just how do we know what we currently know, at all? By answering these questions, we get closer to figuring out what science is. 1.1.1 What is Science and the Scientific Method in a Nutshell At this point, we’ll take a look at what science is from our course textbook A Beginner’s Guide to Scientific Method. Right here in Chapter 1, page 5, we read: “Science is that activity which aims to further our understanding of why things happen as they do in the natural world. It accomplishes this goal by applications of the scientific method.” So, what exactly is the scientific method? Our textbook goes on to explain, “…it is the process of observing nature, isolating a facet that is not well understood, and then proposing and testing possible explanations.” Observe. Explain. Test the explanation. 1 Lecture 1 HSI1000 The facts, ideas, and concepts we find in textbooks have all been put through this process. The knowledge in textbooks is really only one part of science – it’s essentially the explanation part with some observations usually thrown in here and there. Rarely do we read about the rigorous testing the explanations went through. Nor do we hear much about those wrong explanations given earlier, and how testing, or experimentation, was used to eliminate them and home in on what now lies within science textbooks. 1.1.2 Science is Self-Correcting You see, science is self-correcting. The vast majority of the knowledge found in today’s textbooks was all hard won, with multiple wrong explanations being proposed and then discarded until arriving at the current version. This may yet be undone if some new test of that understanding reveals it's lacking in some way. This testing and refinement of our knowledge and understanding is the nature of Scientific Inquiry, the main topic of this course. The best way to understand scientific inquiry is through examples and application. The topic we’ve chosen to look at to gain an understanding of scientific inquiry is perhaps the single most important problem facing our species today – that is, climate change and loss of biodiversity. It is science that offers us our best chance at figuring out how we can get out of this mess – and what all of us need to do to get there. OK, before we get into this serious problem, we need to get a good understanding of this “observe, explain, test” approach to knowledge discovery. Did you know that any time you troubleshoot something you’re actually applying the scientific method? For example, let’s take a look at troubleshooting a laptop that doesn’t bootup in our next video. 1.2 A PC Won’t Work 1.2.1 Troubleshooting a Laptop We find that our laptop doesn’t boot, so we troubleshoot it, which is an example of a straightforward application of the scientific method. Observation Laptop doesn’t boot Explanation Battery dead Test the explanation Plug in external power Result of test Laptop seems to boot, so the battery must have been dead But now there’s a new problem. Re-running through our “observe, explain, test” steps again we find: Observation Laptop seems to boot, but there’s nothing on the screen Explanation Laptop monitor not working Test the explanation Try connecting the external monitor with HDMI cable 1 Laptop seems to boot, but there’s nothing on the screen. (a) Either the graphics card or motherboard has issues, Result of test or (b) Something was wrong with our test. 2 Lecture 1 HSI1000 Perhaps there is something wrong with our test. The external monitor clearly worked because we used the external monitor before conducting this test, albeit with a different cable, and it worked! We change our HDMI cable and retry the “Test the explanation” step below. Observation Laptop seems boot, but there’s nothing on the screen Explanation Laptop monitor not working Test the explanation Try connecting the external monitor with HDMI cable 2 Result of test Laptop definitely boots and the external monitor shows the start screen. After performing a series of tests in the “real world”, that is, by testing our explanations, we discover the following things we didn’t know before performing any tests: We had a dead battery. The graphics card and motherboard are working fine. The laptop’s monitor doesn’t work. A bad connection? Screen broken? We have a faulty HDMI cable. 1.2.2 Troubleshooting and the Scientific Method Troubleshooting is an example of the scientific method. It is in a sense a trivial example of it – the scientific method is a lot more powerful than that. The scientific method can be used to probe and discover brand new things about nature. This, of course, relies on our explanations and then testing those explanations. If our explanation ends up not being falsified, then we have support for our explanation and the more we test our explanation the more confidence we have that our explanation is, in fact, true. This is how things are discovered using the scientific method, and this is the way we have discovered many things about the world today. All the content in science textbooks have been subjected to this procedure. This example illustrates how science is self-correcting, where our steps taken above can be summarized in the following flow chart. We also note that every time we loop through this chart, regardless of whether the test is consistent with the explanation or not, we gain new information about the world around us. 3 Lecture 1 HSI1000 1.3 Teabag Experiment In the previous video, we saw that troubleshooting a laptop was an example of the scientific method. In fact, troubleshooting anything in general represents an application of the scientific method. You observe that there is clearly something wrong. You come up with possible explanations as to what might be the cause of the problem. Finally, you go through the process of checking if the thing you thought might be wrong is indeed the cause of the problem. If it isn’t then you try something else, i.e., you toss aside your previous explanation of what the problem was and make a new explanation for the cause. You continue doing this until you can at least narrow down what’s the cause of the problem. Observe, explain, and test the explanation – the scientific method in a nutshell. The point is by running through this process you will discover things you didn’t know before. Now I’ll illustrate this again, but in the household environment. This time we’ll use the scientific method to discover something new about nature, or at least something that might be new to you. (1) Tea bag bloats and floats on top of the water when boiling water is poured directly on top of it. Observations (2) Tea bag doesn’t bloat and sinks in the water when boiling water is poured on the side and not directly onto it. Water poured on top of the tea bag fills the pores of the teabag itself, trapping any gas inside before it can escape. The hot water heats the trapped air, causing it to expand. The trapped air prevents the tea bag from being dunked. Explanation Pores of the tea bag can get sealed up with water and prevent air from escaping. Quickly seal the tea bag in cold water, trapping the air, then pour boiling water near Test the explanation to but not directly onto it. Result of test Tea bag bloats and floats on top of the water supporting the explanation. The test supports our explanation, rather than confirm it. If we really wanted to check whether this explanation is correct, we would need to do a lot more tests. Compared to troubleshooting the laptop in the previous section, the results of the test with the tea bag are a lot more tentative since we generally know more about the way a laptop works and our tests are more directly indicative of the explanation being correct (or not) than the tea bag. We don’t know that much about what’s going on exactly. We have this speculative 4 Lecture 1 HSI1000 explanation that water is somehow filling up pores, sealing that tea bag, and our test is supportive of this explanation. But ideally, we would find out a lot more about this phenomenon before we can say for sure that this explanation is correct. There are lots of additional interesting questions we can now ask about this phenomenon of liquid water stretching across tiny gaps in the teabag and effectively sealing the air inside: If there really is water sealing the pores, then can we see it? How thick is the film of water sealing the pores of the teabag? How long does this film last? How strongly does the water seal in the air? i.e., what level of air pressure is needed to break the seal? How big do the pores need to be so that the water can’t seal air inside them? Does adding other substances to the water change this behavior? What about other liquids? Do they behave in the same way? In science, answering one question often leads to more questions, and an investigation can sometimes take wild and unanticipated twists and turns that lead to new knowledge and understanding of nature. 1.4 Cadaverous Poisoning You may not have realized it, but in the previous video we were just starting to scratch the surface of two entire branches of science: “Interface Science” and “Colloidal Science” – extremely important areas within chemistry, food science, biology, and physics, etc. A good understanding of these areas is also of great importance to industry and for most of the products industry produces. 1.4.1 Semmelweis and Childbed Fever I have one last example of a fairly straightforward application of the scientific method, taken from our textbook and drawn from the annals of science. Our example will be from the area of medicine and we’re going to wind the clock back to 1846, to a time when science had yet to discover the “germ” – that is, those nasty little disease-causing organisms so small they are invisible to the naked eye. Dr. Ignaz Semmelweis (Figure 1) was hired on a three-year contract into the Vienna General Hospital's maternity clinic from 1846 – 1849. At the time “childbed fever”, aka puerperal fever, was running rampant in hospitals all over Europe and the US. This disease affected mothers after they gave birth or had a miscarriage. Without going into details, it was a particularly nasty disease with a number of easily recognizable signs and symptoms. It usually occurred after the first 24 hours and within the first ten days following delivery. The fever was deadly, killing up to 80% of those diagnosed and sometimes reached as high Figure 1 Semmelweis from as 40% of all mothers admitted to the hospital. As with all physicians, Dr. Semmelweis was Wikimedia: Jenő Doby, particularly concerned, so he studied the data on mortality rates within his hospital dating Public domain, via Wikimedia Commons from 1841 – 1846 looking for something, anything, that might give a clue on how to stop the fever. 5 Lecture 1 HSI1000 He noticed something when comparing the mortality rates between two different clinics in his hospital (Figure 2). Now there was no obvious difference between the two sets of mothers in each clinic, so why were a lot more women dying in his clinic where doctors attended to mothers (orange curve in Figure 2)? He showed the findings to his colleagues, but everyone was at a loss as to why this should be so. Was it something the doctors were doing wrong? or perhaps something the midwives were doing right? Maybe it Figure 2 Mortality rates in two clinics in the Vienna General Hospital from 1841 was due to a difference in the clinical - 1846. environments? Perhaps the patients in his clinic were somehow more prone to illness? At this time, in the middle of the 19th century, the “germ” or pathogen, was not known to be the cause of disease. Germs had not been observed, and any speculation of their possible existence was not taken seriously. The reining theories for how disease was spread, caused, and treated at the time were quite wrong. 1.4.2 A Key Observation Then a rather unfortunate incident occurred in 1847 in an anatomical pathology lab. Dr Semmelweis’ friend, who he greatly admired, died after being accidentally pricked by a scalpel being used by a student doctor while he was assisting in performing an autopsy. Professor Kolletschka (Figure 3) suffered identical signs and symptoms as the mothers who died of childbed fever. Dr Semmelweis wrote about the incident: “Day and night I was haunted by the image of Kolletschka's disease and was forced to Figure 3 Kolletschka recognize, ever more decisively, that the disease from which Kolletschka died was from Wikimedia: Unknown author, Public domain, identical to that from which so many maternity patients died.” via Wikimedia Commons There is another very pertinent fact, or observation, in this case. Right after the student doctors attended the anatomical pathology lab, where they dissected badly infected corpses, they would go to Dr Semmelweis’ maternity clinic to assist in the births of expectant mothers. Do remember that the way disease was spread, caused, and treated in those days was completely misunderstood. No one knew about germs, so there certainly were no antibiotics and there was no disinfecting of anything – no hand washing of one’s hands especially when, by just looking at them, they were quite obviously clean. So now we have our careful observations, or facts, (1) The mortality rate of mothers due to childbed fever in a clinic attended by doctors was, on average, five times higher than what appears to be a similar clinic with similar mothers but attended by midwives instead of doctors. (2) Doctors attend to mothers in the clinic directly after having been engaged in autopsies of infected corpses in the anatomical pathology lab. (3) A doctor dies from identical signs and symptoms to childbed fever after having been stuck with a scalpel used to dissect infected corpses in the anatomical pathology lab. 6 Lecture 1 HSI1000 1.4.3 A Possible Explanation Following the scientific method: observation, explanation, testing, what could be a possible explanation for these observations? Semmelweis felt that the only sensible explanation must have something to do with the corpses. Something unseen. He called this something “cadaver matter”, so he speculated that invisible cadaver matter was picked up by the student doctors touching corpses while working in the anatomical pathology lab. This matter wasn’t visible to the naked eye, but it was deadly if it entered the body via a wound. When these same doctors assisted mothers while giving birth, they transferred it to them through the usual wounds suffered during such a process. 1.4.4 Testing the Explanation At the moment, this explanation is pure speculation. It could certainly be wrong, with some other unknown explanation being the right one. Following the scientific method, he needed a means of testing this proposal to see if he was indeed wrong. If his explanation was right, and cadaver matter existed, he decided that, quite obviously, it needed to be removed from the student’s hands before attending to the mothers in his maternity clinic. Therefore, he instituted a policy of using a solution of chlorinated lime (calcium hypochlorite, or swimming pool chemical in water) for washing hands between autopsy work and the examination of patients. He chose this chemical because he found that the same solution worked best to remove the putrid smell of infected autopsy tissue. Now if his explanation was correct and the chlorinated lime solution was effective, then the mortality rates of childbed fever should drop to the same levels as the midwife’s clinic or lower. Despite some resistance to the hand washing, and whispers that he had instituted a waste-of-time hand washing for crazy reasons (like a theory that corpse particles could turn the living into a corpse!), the mortality rates in his clinic dropped remarkably, as shown in the following graph. Figure 4 Monthly Mortality Rates 1841-1849 from Wikimedia: Power.corrupts, Public domain, via Wikimedia Commons 7 Lecture 1 HSI1000 If you’d like to read more about Dr. Semmelweis and this interesting case, just follow the link in the box below. https://en.wikipedia.org/wiki/Ignaz_Semmelweis 1.4.5 In Conclusion To summarize, Dr. Semmelweis made observations and provided an explanation for those observations. Based on that explanation, he performed a test of it with the results supporting his explanation. Of course, there was no explanation of what was “cadaver matter”. Furthermore, since his explanation ran against the generally accepted scientific theories of the time and it wasn’t completely accepted until 30 years later when the cadaver matter was actually discovered by Louis Pasteur in 1879. A revolutionary new theory of disease was then established which remains as the prevailing explanation for diseases to date – germ theory. 8 Lecture 1 HSI1000 1.5 Observation Now that we have a handle on what science is and an overview of the scientific method, let’s address our second intended learning outcome for this lecture, which focuses on the first step of the scientific method – “scientific observation”. Observation is absolutely critical to conducting any kind of scientific inquiry. In fact, without any observations, or experiments – which are just observations themselves – the inquiry isn’t even considered scientific! Observation fulfills the following crucial roles in any scientific inquiry: 1. To identify and focus on the relevant facts about the phenomena under investigation. 2. Provide clues as to what might explain the phenomena – this one’s really important if the phenomenon you’re investigating is really mysterious. 3. Provide the evidence by which we can determine whether various explanations succeed or fail. However, making useful observations can sometimes be tricky. For example, we might not know which data will be relevant to the solution to the problem at hand. Even if this is known, we can run into trouble just gathering the necessary data in the first place! The second chapter of our textbook discusses everything we need to know about making useful observations. I suggest you read it in combination with this lecture. The chapter has several accessible real-life examples illustrating five main concerns that need to be addressed when making useful scientific observations. Pay close attention to the “Concept Quiz” on page 25 and try and answer the 9 questions asked there. In fact, I’ve given you a head start by answering the first question for you here. To illustrate most of these five concerns, we’ll use an important example from one of the greatest scientific minds that ever lived. This scientist was a pioneer of the scientific method and instrumental in ushering in a permanent change in the way in which knowledge and understanding of nature were acquired. The next video will show how the one and only Galileo Galilei figured out the law of inertia, which Newton later incorporated into his first law of motion. It’s important to note here that it’s not the actual law of motion we are particularly concerned with – I won’t be asking you to do any problems using it. What we’re interested in in our next video is how Galileo worked it out from his observations of motion. In particular, how he addressed the following concerns when making his observations: 1. Do we have a clear sense of what the relevant phenomena are? 2. Have we found a way to ensure we have not overlooked anything in the process of making our observations? 3. Do we know for sure what is based on fact and what on conjecture or assumption? 4. Have our observations been contaminated by expectation or beliefs? The 5th concern is – “Have we considered any necessary comparative information?” We have already seen a nice example of this through the observations made by Dr. Semmelweis. You should be able to recall what those comparative observations were and why they were critical to his investigation. 9 Lecture 1 HSI1000 1.6 The Keen Eye of Galileo When a whole bunch of different objects are spilled across the floor, we notice something. Some come to rest almost immediately, while others continue to move, then come to rest, and a few take quite some time before inevitably ceasing to moving. Observing such motion with an Aristotelian worldview simply reinforces the idea that all objects when given a push will come to rest because coming to rest is the nature of solid objects. This is because solid objects are of the Earth, and the Earth doesn’t move, in the Aristotelian world view. In this worldview, pushing an object and making it move is against its nature, so it will come to rest as soon as the pushing stops. Because of this (wrong) idea, in order to have an object continue to move forever, it would need to be continually pushed forever. The thing is, when Galileo observed this exact same thing he came to the exact opposite conclusion, and this was because of his objective, careful, and thoughtful observations of motion. In what follows we will discuss just what Galileo discovered and how he did it. 1.6.1 Galileo’s Key Observation and Realization Galileo was somehow able to ignore the reining Aristotelian worldview and look more closely at the behavior of objects slowing down and coming to rest. His keen eye and intellect were not contaminated by expectation or belief in the Aristotelian worldview. He realized that an object’s nature being supposedly stationary was pure conjecture, a belief, and not a fact. This enabled him to notice something that others completely overlooked, and that was this: Depending on the type of contact that an object made with a surface it could move more, or less, further. Figure 5 illustrates the tiny contact made between a ping-pong ball and a hard, flat surface. This contact had nothing to do with the nature of the object itself. In fact, it was the nature of the contact between the object and the surface that caused the object to slow down, not the nature of the object itself. The influence of the contact on the motion of the object was an extraneous effect - a separate effect, that hid the true nature of what was happening. By realizing that the slowing down was due to an extraneous effect that was interfering with something about nature that was more fundamental was sheer genius. In doing so, Galileo had gained a very clear sense of what the relevant and irrelevant phenomena were. He then set about designing and performing experiments that could reveal what this underlying Figure 5 The tiny contact made between a ping-pong ball and a hard flat surface. fundamental truth was about the motion of objects in the world. 1.6.2 Galileo’s Experiments with Inclined Planes Galileo set up some experiments not too unlike what is shown in Figure 6. We have a track that is inclined at the ends, and a ball. He released the ball a certain distance up one incline and watched carefully how far the ball rolled up the other incline before stopping and heading back down again. Figure 6 Experimental setup to study the motion of objects 10 Lecture 1 HSI1000 He found that as he reduced the influence of the contact between the ball and the track, that the ball could all but reach the same height up the other incline, and no further (Figure 7). He found that this remained true as he reduced the angle of the incline the ball ran up on Figure 7 After releasing the ball show in Figure 6, the ball almost reaches the same height up the other side. the other incline But here’s the really interesting thing. As shown in Figure 8, the lower he made the angle, the further the ball traveled before achieving virtually the same height on the other side of the track. The ball only lost height because of the extraneous effect due to the contact it was making with the track. Figure 8 A ball released from the red line travels a greater and greater distance (indicated with an orange arrow) as the incline angle is reduced before turning back and heading downslope again. 1.6.3 Galileo’s Conclusions What if the angle was reduced all the way to flat, i.e., horizontal? It was the influence of the contact that slowed the ball, and not the ball itself that caused it to slow down. If the influence of this contact were made 11 Lecture 1 HSI1000 zero, then it would mean that the ball would continue rolling - continue moving forever. It would never slow down and stop. No force was needed to keep such an object moving forever. It would simply continue moving forever of its own accord, not speeding up, nor slowing down. In this way, he discovered a fundamental law of nature. Any object, if given a push, will continue moving forever at the same speed unless something acts to slow it down, like a hillside, or the slowing-down contact it is making with the surface. The worse the contact, the greater the slowing down. 1.6.4 Addressing Important Scientific Observational Considerations Galileo deduced a fundamental law of nature from his experiments, and it was the opposite result from the Aristotelean view about the motion of objects. Galileo was only able to do this because he (1) had a very clear sense of what the relevant and irrelevant phenomena were, (2) he didn’t overlook anything when carefully observing how objects moved on a surface, (3) he knew all too well what was based on fact and conjecture in the Aristotelian view of how objects moved, and he certainly made sure his observations were (4) not contaminated by expectation or belief. These were the 4 important considerations that must be taken into account when making careful scientific observations that I wanted to illustrate for you with this example. There is a 5th one, which I mentioned to you already in the last video. 12 Lecture 1 HSI1000 1.7 The Scientific Revolution Now that we have an idea of what science is, the scientific method and making useful scientific observations, we shall look at how this method of knowledge discovery came to be developed and accepted within a fledgling scientific community in the first place. By briefly looking at some of the history of the scientific revolution, we’ll be able to address the 4th and 5th intended learning outcomes of this lecture. Here, I am adopting the traditional view of the Scientific Revolution, i.e., when and where it began and ended. There are certainly other perspectives on this revolution, just take a look at Wikipedia on the Scientific Revolution as an example. If you scroll down the page, under the “Criticism” section, you’ll find something there on the “Continuity thesis”. We also need to realize that other earlier scientists had also used the scientific method in discovering new knowledge, like Ibn al-Haytham (“the father of modern optics”, Figure 9) who, at around 1000 A.D. during the Islamic Golden Age, made significant contributions to the principles of optics and visual perceptions. If you’d like to get a more fully appreciate the scientific revolution, there are several reasonable videos on YouTube on this topic, and even videos on the entire history of Figure 9 Hazan from science. I’ve included some of these links below if you’d like to dig deeper. Wikimedia: artwork drawn by Adolph Boÿ, engraved by Jeremias Falck, Public domain, via Wikimedia Commons From “Crash Course” The Scientific Revolution https://youtu.be/vzo8vnxSARg https://youtu.be/w70BkCqgyyI The History of Science Many videos here. Here’s just the preview… https://youtu.be/-hjGgFgnYIA To be honest guys, I haven’t watched all 47 videos introduced above by Hank Green. I’m just pointing you towards this additional material if you’re really keen. I do enjoy listening to Hank Green, although I might not always agree with him. Anyway, take a look and see what you think. 1.7.1 Revolutions Within Scientific Subdisciplines There’s also one more point I’d like to make before our brief account of the scientific revolution- there are also revolutions within each of the various subdisciplines in science, like Chemistry, Medicine, Biology, Physics, etc., each of which totally changed the way people thought about and treated the subject. But it is the Scientific Revolution we’re discussing now, i.e., the fundamental change in the way people thought about knowledge acquisition as a whole and the subsequent adoption of this approach by newly formed scientific societies. 13 Lecture 1 HSI1000 It is the American Physicist, historian, and philosopher of science, Thomas Kuhn (Figure 10) who wrote about scientific revolutions, in general, in his book “The Structure of Scientific Revolutions” (Figure 11). This book was extremely influential, but we won’t be covering his ideas here. Instead, I’ve added two excellent 9 and a half minute videos from Leiden University on Thomas Kuhn’s ideas. I recommend that you watch both of them because they are quite Figure 10 Thomas interesting, informative and definitely thought-provoking as they Kuhn from deal with the nature of scientific revolutions, “normal science” and Wikimedia anomalies. Figure 11 Kuhn's Book from Wikimedia From Leiden University – Faculty of Humanities https://youtu.be/sOGZEZ96ynI (Normal Science) https://youtu.be/JQPsc55zsXA (Scientific Revolutions) I personally think the presenter overstates things, but I’ve added these videos above for you nevertheless. There are also other videos on that channel that discusses Karl Popper, whose ideas are subtly embedded into this course (falsifiability), but this is getting off track for this course. The key idea in the videos above is the role anomalies play in scientific endeavor, and that many subdisciplines of science have their own “revolutions” – forever changing the way people thought about the topic, or even creating entirely new fields of knowledge and ways of understanding of the natural world. 1.7.2 The Beginning of the Scientific Revolution – Copernicus First up we have Nicolaus Copernicus (Figure 12) who, in 1543, published an astronomy book, known in short form as De Rev (Figure 13), on how the planets orbited the Sun and NOT the Earth, and that the Earth itself rotated. This proposal was in direct opposition to the accepted idea that the Earth was the center of the universe, and that everything rotated about the Earth. Figure 12 Nicolaus Copernicus from “Accepted idea” meant that the state religion of the time Wikimeaia: Toruń Regional Museum, Public domain, via Wikimedia simply stated that this must be so. They were an authority – Figure 13 De Rev from Commons meaning that their word could not be argued with, and it was Wikimedia: derivative work of Johannes Petreius 1543 edition of a crime to do so – a crime called heresy. File:Nicolai_Copernici_torinensis_De_rev olutionibus_orbium_coelestium.djvu, This authority considered astronomy and the motion of the Sun, moon, and planets Public domain, via Wikimedia Commons to be part of their “turf”, so to speak. The reason for this was that it was literally believed that the stars and planets were heavenly bodies and a part of heaven. This is why they considered astronomy as their “turf”; so there was no questioning what they said, it was just a simple fact not to be argued with. Now Copernicus knew his idea that the Sun, and not the Earth, being the center of the Universe could get him executed, so he made sure his book was published posthumously so that there was no way they could get to him. Secondly, he stated in his book that his proposal shouldn’t be thought of as real, but was just another, simpler, way of predicting where the planets would be during the year. You see, being able to make these predictions was considered particularly important to many powerful and influential people at the time. 14 Lecture 1 HSI1000 For 1500 years, an extremely complicated system was devised for the motion of the planets where the Sun and the moon orbited around the Earth. This has been attributed to Ptolemy (Figure 14), a second century astronomer of Alexandria under the rule of the Roman Empire. Copernicus’s view of how the planets moved replaced the complex Ptolemy system shown in Figure 15 with what is Figure 14 Ptolemy from given in Figure 17. Wikipedia: Unknown authorUnknown author, Public It’s important to realize that Copernicus’s newer domain, via Wikimedia Commons and simpler system wasn’t any better at predicting where the planets Figure 15 Epicycles for Mars about the Earth would be than the Ptolemy system, mostly because Copernicus from Wikipedia believed, as Ptolemy did, that the planets and moon must move in perfect circular orbits with uniform speed. But nonetheless, Copernicus’ proposal was definitely a step in the right direction. 1.7.3 Galileo Next, we have Galileo Galilei, who in the late 1500s and early 1600s, studied the motion of objects with experiments to test current explanations of how things moved. He also performed astronomical observations to test Copernicus’ suggestion of a Sun-centered universe instead of an Earth- centered one and to test the Aristotelian view of the heavenly bodies. Figure 16 Galileo Galilei from It was Galileo’s work in astronomy that really got him into Wikipedia: Justus trouble. You see, Galileo built powerful telescopes Sustermans, Public domain, via Wikimedia Commons Figure 17 Copernicus' Sun-Earth-Mars possessing a magnification of 30 times which was much more powerful than System the telescope of that time which only had 3x magnification. With this telescope, he tested the Aristotelian view of the heavenly bodies and a whole bunch of new phenomena could be plainly seen by any who cared to look. This is another key feature of science. Some new instrument is invented that allows us to make observations that couldn’t be made before. These new observations can turn up “anomalies”. An anomaly being something or some state of affairs that can’t be explained with the current understanding of nature. Anomalous phenomena and observing anomalies are discussed in our textbook in Chapter 2 pages 18 – 23. So, with Galileo’s much more powerful telescope than anything that was available at the time he saw a whole bunch of anomalous phenomena. The moon was clearly bumpy and cratered (Figure 19). Obviously, it wasn’t a perfectly smooth sphere, which heavenly bodies were supposed to be according to the Aristotelian view. Also, he observed moving sunspots on the sun (Figure 18), so it became obvious that the sun was (a) NOT a perfectly clean sphere, it had these spots on it, and (b) it rotated and wasn’t stationary! He saw 4 Figure 19 Galileo's moons orbiting around Jupiter (Figure 20). Figure 18 Galileo's drawing of our sketchs of sunspots from moon from This was a real problem. Up until this point, all heavenly bodies Wikipedia: Galileo, Public domain, via Wikimedia Commons Wikipedia: Galileo, CC BY 4.0 observed appeared to be orbiting the Earth, but now this planet, , via Jupiter, had its own moons and they are orbiting it, not the Earth! Wikimedia Commons 15 Lecture 1 HSI1000 He also recorded all the phases of Venus (Figure 21), which was a prediction and a test of a Sun-centered universe. Seeing all the phases of Venus proved it orbited the sun because in an earth-centered universe, you wouldn’t be able to see all the phases of Venus. All these observations led Galileo to realize that the Aristotelian worldview must be wrong and that the Sun, not the Earth, was the center of the universe. But Galileo, who based his theories on experiments and not simply the word of the ancients, flew directly in the face of authority. To cut a long story short, Galileo published a book stating his position clearly and heavily criticizing the Aristotelian worldview. His book was immensely popular. He was arrested and charged with heresy, but “got off” on a Figure 21 Galileo's phases of venus - Figure 20 Galileo records Jupiter's moons - public lesser charge of “vehemently public domain image domain image suspect of heresy” when he recanted and said he must have made a mistake. Of course, he hadn’t, but house arrested for the rest of his life rather than being tortured and burnt at the stake seemed like a better option to him. 1.7.4 Kepler Our third participant is Johannes Kepler (Figure 22), who was also working at around the same time as Galileo, proposed an improved model for how the planets moved over Copernicus’ model. Kepler, who had access to some of the most accurate measurements of planet positions during the year, simply could not get the positions of the planets to match with a model like Copernicus’s, where the orbits were perfectly circular executing uniform circular motion. Figure 22 Johannes So, following the scientific method, he completely abandoned this explanation of how the Kepler from planets moved and came up with the idea Wikimedia: Unknown author, Public domain, via of the planets orbiting about the Sun in Wikimedia Commons ellipses, rather than circles. Along with his other laws of planetary motion, he produced the most accurate predictions, up until that time anyway, of where all the planets would be at any time of year (Figure 23). However, there was still no explanation as to why the planets, sun, and moon should behave in such a manner, but considering the accuracy of the model, it did seem that this is just what they did. Figure 23 Kepler's planetary orbit from Wikimedia So, why should the heavenly bodies behave in such a manner? The answer to this question marks the traditional view’s end of 16 Lecture 1 HSI1000 the scientific revolution. But before ending our recount of the scientific revolution, we need to learn about the then-highly influential English politician and philosopher, Sir Francis Bacon – our fourth participant. 1.7.5 Bacon Bacon (Figure 24) is commonly accepted for articulating the scientific method we have been discussing in 1620. Sometimes called the father of empiricism, Bacon convinced the fledgling scientific community that the only way to get to the truth of some explanation was by testing it through observation or experimentation. A scientist should not simply accept an explanation as true but should doubt it and try to disprove it through observation and experimentation, i.e., empiricism. Only then, when all possible testing has been conducted, could one hope that the explanation may indeed be true. Without Figure 24 Francis Bacon from Wikipedia: Paul van this skepticism then there is no basis, or evidence, that the explanation is correct, we are Somer I, Public domain, via Wikimedia Commons forced to conclude that there’s no way of knowing if it’s true or not. This testing of explanations – the weighing of the truth – as articulated by Bacon, remains absolutely foundational to the scientific method today, and it was during the scientific revolution that this approach was crystalized. For now, let us finish our account of the scientific revolution by considering the work of Sir Isaac Newton. 1.7.6 The End of the Scientific Revolution – Newton Our final participant is Isaac Newton who integrated the earlier work of Galileo on how objects move and Kepler’s work on how heavenly bodies move, along with the work of others and his own experimentations. Isaac Newton published his famous book Philosophiæ Naturalis Principia Mathematica, in English “Mathematical Principles of Natural Philosophy”, or just Principia for short, in 1687. The book represented a stellar breakthrough in the understanding of how all objects moved. Newton presented his three laws of motion, which were Figure 25 Isaac figured out by observations and experimentation on how Newtom from Wikipedia: Godfrey Kneller, objects moved on Earth. He also presented the law of Public domain, via Wikimedia Commons universal gravitation which described how all objects with mass attracted each other. But when you combined these two things you could mathematically derive Kepler’s laws of motion of the planets. It was truly shattering because, until this time, the motion of heavenly bodies and the motion of things on Earth were thought to be entirely unrelated. Indeed, the Aristotelian worldview required that they were entirely different because the planets were literally thought to be a part of heaven. How could anything figured out on Earth be used to describe heaven? Well, evidently, the planets weren’t part of heaven. Figure 26 Principia from Wikipedia: The original uploader was Zhaladshar at English Wikisource., Public domain, via Wikimedia Commons Newton’s single law of universal gravitation combined what was considered totally separate types of motions, the motions taking place on Earth and those taking place in the heavens, into one unified whole. The laws of motion allowed the exact mathematical description of the motion of all objects familiar to humans at the time. The new mechanics, called classical mechanics today, accurately describes the motion of all projectiles, parts of machinery, and astronomical objects. It was only until the extremes of nature were encountered, using new instruments of observation, like the very tiny atoms or the very dense and massive stars, or the very fast, moving near the speed of light, was there a need to improve on his physical theory of classical mechanics, which didn’t happen until the 20th century. 17 Lecture 1 HSI1000 1.7.7 The Scientific Community This marks the end of the traditional view of the scientific revolution. At the conclusion of the 17 th century, with the incredible success of Principia in explaining so much, the last vestiges of the Aristotelian worldview were swept away into the annals of history, at least in the realm of physics. But medicine, chemistry, and biology would soon follow with their own revolutions. A brand-new era was ushered in. One in which not just individuals practiced the scientific method, but an entire community of like-minded scientists worked together for the betterment of humankind. Scientific societies were established, and discoveries discussed, debated, and put under the microscope of rigorous scientific testing. The importance of a community in science cannot be understated. Individual scientists may be blinded by their own expectations or beliefs, or perhaps they are unable to differentiate facts from conjecture or assumption. But with a scientific community, individual work can be checked, cross-checked, and closely examined by all, accelerating the pace of knowledge acquisition manyfold. Let’s end this section with the motto of the Royal Society (established in late 1660) “Nullius in verba”, which means “take nobody’s word for it”. 18 Lecture 1 HSI1000 1.8 The Industrial Revolution The development of the scientific method, well established and accepted by the end of the scientific revolution with the formation of a fledgling scientific community, directly resulted in further great discoveries which in turn produced the industrial revolution. The industrial revolution was when great technological innovation literally changed the world – where we transited as a species from relying on muscle power to get things done, to machines doing the work. It set us on a course towards overpopulation, climate change and biodiversity loss we have today. Addressing the 6th and final intended learning outcome for this lecture involves an appreciation of how scientific discoveries can transform society. In this case, it’s the discovery of energy, heat, work, and the second law of thermodynamics and their application in the construction of an efficient and practical steam engine. The invention of such a machine drove the industrial revolution. While an entire course could easily be spent discussing the Industrial Revolution, here we’ll just focus on those aspects of it directly relevant to us. If you’re keen to learn more about it, just look it up on Wikipedia, or even check out some of the videos on YouTube linked below. So, what is the industrial revolution? From “Crash Course” Well, it commonly refers to a period of great societal change that took place, The Industrial Revolution first in Britain, Western Europe, followed https://youtu.be/zjK7PWmRRyg (And note the importance of by the US and Canada and later Japan the steam engine) and other countries as they too https://youtu.be/FCpqN7GmLYk (Again, notice the importance industrialized. of the steam engine) So, the industrial revolution is when Wikipedia industrialization first took place. It https://en.wikipedia.org/wiki/Industrial_Revolution occurred around the late 1700s up until about the early 1800s in Europe and Northern America. Before the Industrial Revolution, most labor was performed by people and animals – muscle power was relied upon to make things and get things done. However, this all changed during the Industrial Revolution with the invention of a practical steam engine to power machines to do the work much more effectively and efficiently. Of course, people still needed to run the steam engine-powered machines, but the machines could be used to manufacture items in far greater numbers than people could without them. Textiles were the primary products impacted by the revolution in terms of employment, value-added, and capital investment. As an example of just how much more effective the machines were - mechanized cotton spinning, powered by the steam engine increased the output of a worker by a massive factor of 500! Iron production was also greatly enhanced by using the steam engine to power blast air into the furnaces. This was done using coal, which was much cheaper than the previously used charcoal. Nevertheless, industrialization is all about manufacturing on a large scale in factories, which are in cities, and powered mostly by steam engines. With this manufacturing taking place in cities, replacing the “old ways” of producing things, i.e., by hand and in cottages, a lot of work was to be found in urban centers. City populations increased rapidly. During the industrial revolution, almost every aspect of daily life was influenced in some way. Average income exhibited 19 Lecture 1 HSI1000 unprecedented, sustained growth. The standard of living for the general population increased consistently for the first time in history, and overall, there was a massive population explosion. The invention of an efficient and practical steam engine (one example shown in Figure 27) was a result of understanding what energy, heat, work and the second law of thermodynamics are through scientific investigation. Engineers were then able to apply this knowledge to construct them. These machines used steam to produce power, and to make steam you had to heat water. To heat the water, you required an effective fuel, and that was coal (Figure 28). Charcoal is also good, but that needs trees, and if you’ve run out Figure 27 Steam engine action from Wikimedia: Panther, CC BY-SA 3.0 , via Wikimedia Commons of those then you need something else. In Britain, where the Industrial Revolution started, coal is abundant, and it’s here that we see the start of our reliance on fossil fuels. The demand for coal to power steam engines increased dramatically. Now to get coal, you have to mine it, and Britain had lots of it. The thing is, as demand increased, mines needed to be able to go deeper to get the large quantities available to satisfy this demand. The problem was that the deeper you dig a mine, the more likely it fills with water, making the lower parts of the mine inaccessible. How was this problem solved? By pumping the water out, and that was achieved with the steam engine itself, fueled by the very coal it mined. Figure 28 Coal from Wikimedia Coal needed to be transported to the machines located in city factories. For this to happen effectively, and for the goods made in the factories to be moved out easily, roads needed to be significantly improved and transportation itself made more reliable and efficient. Steam engine-powered trains and ships were invented, vastly increasing the efficiency of moving materials around, including food which now was in great demand in the rapidly growing cities. The improvements to agriculture, just prior to the Industrial Revolution, coupled with new machinery now available to assist in farming and effective means of getting it to where it was needed (steam engine) significantly reduced famine across Western Europe. Conditions in the now extremely crowded cities were pretty bad though. Pollution from coal burning and the simple throwing of human waste directly out of windows into the streets, as you can imagine, was absolutely disgusting. Also, remember, no one knew about germs, and germ theory didn’t exist yet, so hygiene was nonexistent. You can imagine, these are the perfect conditions for disease to spread, so outbreaks of cholera, typhoid and typhus were common and devastating, but the greatest killer in the cities was tuberculosis. Some relief was achieved with Figure 29 Pollution during the industrial revolution from the construction of sewer systems in cities to carry Wikimedia away wastes. 20 Lecture 1 HSI1000 Although conditions could be horrible, there was a substantial overall drop in the mortality rate of the population. People, on average, survived longer – long enough to have children of their own. So, while the birth rate was high, roughly 6 babies per woman on average across the world, the mortality rate in the industrialized countries decreased markedly. This was because of 1. The improvements in agriculture allowing more food to be produced. 2. There were improvements in distributing that food, Figure 30 Pollution during the industrial reducing the likelihood of food shortages. revolution from Wikimedia: Henry Linton, Public domain, via Wikimedia Commons 3. Improvements in sanitation with sewage systems put in place in cities. As a result of the fall in mortality rate during the industrial revolution, the population surged. For example, examine Figure 31 from “Our World in Data” for the population of England from 1086 until the end of the Industrial Revolution. Other industrialized nations experienced similar rapid population growth leading to a surge overall in the world’s population during this time. And all of these large numbers of people needed powered machines for food production and distribution as well as for factories and transportation. That power came from the steam engine initially, followed by the internal combustion engine later, and both these types of Figure 31 Population of England before then during the industrial revolution period indicated with a left-brace. machines all used fossil fuels to run. No one at the time could have possibly anticipated that these changes would lead to the crisis we find ourselves in today. Who could imagine that by simply burning fossil fuels with reckless abandon we could end up altering an entire planet’s climate? After all, the Earth is huge, and there is no doubt that if the world’s population remained relatively small there wouldn’t be enough pollution to terraform the entire planet. But the world’s population didn’t remain small. In fact, there was another even more incredible population surge just around the corner, in the 20th century. And it’s this surge in world population that truly takes us into the climate crisis and loss of biodiversity at rates usually only associated with extinction-level events. We will be exploring this population surge in our next lecture. 21