Progressive Exercise Module 5 Notes PDF
Document Details
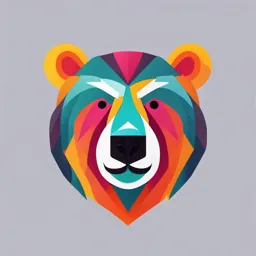
Uploaded by RevolutionaryLosAngeles7449
University of Washington
Tags
Summary
This document provides notes on progressive exercise, including definitions of VO2 max and a description of the progressive exercise tests used in Kinesiology at UW. It also discusses the metabolic and physiological responses to progressive exercise.
Full Transcript
Module 5 - Progressive exercise Cardiovascular, respiratory, skeletal, and metabolic response to progressive exercise Progressive exercise test - incremental exercise tease - graded exercise test are protocols that possess a systematic and linear increase in exercise intensity of a defined people o...
Module 5 - Progressive exercise Cardiovascular, respiratory, skeletal, and metabolic response to progressive exercise Progressive exercise test - incremental exercise tease - graded exercise test are protocols that possess a systematic and linear increase in exercise intensity of a defined people of time until the individual is unable to maintain or tolerate the workload. The progressive exercise test protocol used in Kinesiology at UW Start at rest then increase the rate at predefined times. Continue this until the individual can no longer continue. VO2 Max physiological definition - Maximum ability of the respiratory and cardiovascular systems to take up O2 from the atmosphere, deliver the O2 to the skeletal muscles (and other tissues/organs), and for the mitochondria to utilize the O 2 to produce ATP VO2 Max Graphical definition - VO2 max occurs when there is leveling off or plateau in VO 2 despite an increase in workload. VO2 response to progressive/incremental exercise is linear. Plateau is not defined as a straight horizontal line; it is an increase in VO 2 of less than or equal to 150 mL/min with an increase in WR What if VO2 does not plateau? ○ Achieving a plateau in VO2 is not seen in every test. This can be observed less than 20% of the time. Sometimes it occurs 100% of the time - depends on participants. Then use the tear ‘VO2’ peak instead of ‘VO2 max’ If we don’t see a plateau - Secondary criteria indicating a successful max test: ○ Volitional fatigue - the feeling that you absolutely cannot run/pedal anymore. In the case of cycling, you cannot maintain the cadence (RPM - revolution per min). In the case of treadmill running, you cannot maintain the pace. ○ RER greater than or equal to 1.15 RER can be greater than 1 due to non-metabolic CO2 that is produced due to buffering of acid; this increase VCO2 above VO2 ○ Acheiving HRmax = 220-age or HRmax = 208 - (0.7 x age) ○ RPE (rating of perceived exertion) greater than or equal to 17 (using Borg’s 6-20 scale) ○ Some people use blood lactate, but it is quite variable (because the more active you are, you can have low blood lactate even if you reach VO 2 max. VO2 max (peak) values attained on a treadmill tend to be greater than a bike ○ VO2 max (peak) observed on a treadmill can be up to 20% greater than that observed on a cycle ergometer ○ What contributed to the greater VO2 peak observed when running? Larger active skeletal muscle mass - leg, arms, postural, etc. A bike supports your body mass while on a treadmill you have to do that on your own Higher cardiac output Larger venous return (how much blood is actually returning to the heart) can influence SV and therefore CO Larger extraction of oxygen (a-v)O2 Greater vascular conductance (blood flow normalized to mean arterial pressure); more dilation occurring and more blood flow Force production during progressive exercise Linear increase of EMG Linear increase of VO2 is because of linear increase in oxygen demand We are looking at the recruitment of muscles ○ We know that alpha motor neurons that innervate type I fibers have a low threshold for activation and they are recruited first ○ During a GXT the work rate goes up every 2 minutes (ie the exercise intensity increases) and to push the pedals and maintain cadence (rpm) your muscles must generate more force ○ Eventually, you will recruit all type I fibers (remember this is not a hand-off, they still generate force) and get to a point to the recruitment of the highest threshold alpha motor neurons that innervate type IIx fibers. I → IIa → IIx ○ Think about the process that requires ATP - ATP demand increase; linear increase in VO2 means a linear increase in O2 demand and ATP demand. ○ When 100% of the VO2 max is reached, all the fibers are contributing to the movement. ○ As exercise intensity goes up, you need to generate more force to push those pedals. To generate more force you can do 2 things Can increase the action potential frequency Recruit more skeletal muscles Substrate utilization during progressive exercise: the crossover concept Where are we getting the energy from to produce ATP? Fat vs carbohydrate At light exercise intensity, relatively speaking, we are utilizing more fat. ○ Around 67% of ATP is coming from fatty acids and around 33% from carbohydrate oxidation As you perform progressive/incremental exercise, there is a cross-over in the substrate utilization; you will utilize less and less fatty acids to produce ATP and you will use more carbohydrates (glucose) to produce ATP. As exercise intensity increases there is an increase in reliance on glucose oxidation instead of free fatty acid oxidation. Why? ○ Recruitment of Type II fibers (fewer mitochondria, more glycolytic enzyme relative to type I) They are better built to perform glycolysis and to generate ATP glycolytically. ○ There’s also an increase in [Epi] epinephrine in the blood which stimulates phosphorylase (the enzyme that breaks down glycogen) and increases glycolytic rate due to higher substrate = increase lactic acid production ○ Increased lactate means increased acid [La- + H+] in the blood. Hydrogen ions ,H+ inhibits lipases, which reduces the rate of lipolysis = reduces FFA (free fatty acid) delivery to skeletal muscle. ○ Reduced blood flow to adipose tissue (blood flows to the muscles that need them instead) Blood lactate response to progressive exercise Lactate threshold - the work rate where blood lactate begins to increase exponentially (somewhat subjective, there are mathematical means to determine lactate threshold) The onset of blood lactate accumulation (OBLA) - the work rate where blood lactate equals 4mM (less subjective but still not ideal). ○ x-axis can be both work load or oxygen uptake What determines the concentration of lactate in the blood? Rate of appearance (Production of lactate) ○ Increases during GXT (graded exercise test) ○ recruitment of Type II fibers (better suited to produce ATP anaerobically) ○ increase in Epinephrine [Epi] = increase phosphorylase activity = increase glycolytic rate = increase lactic acid production Rate of disappearance ○ Removal of lactate from the blood by other tissues (eg. the Cori cycle in the liver) You will reach a point where the rate of appearance outweighs the rate of disappearance and as a result: accumulation of lactate in blood Relationship between VO2, VCO2, RER, blood lactate, and VE A higher number of active muscle fibers = a higher rate of CO 2 production (Kreb’s cycle is active in more cells) At some point, VO2=VCO2 which causes RER = 1.00 At a very heavy work rate, VCO2 will exceed VO2, which is what causes RER to be greater than 1. ○ At the point of RER being greater than 1, we are still utilizing 100% glucose to produce ATP but we have excess CO2 that comes from metabolic CO2 and H+ buffering in blood from lactic acid - recall bicarbonate buffering. CO2 and H+ are potent stimulators of ventilation - VE will increase disproportionately at high WRs. Note: linear at low-moderate WRs to match ventilation to metabolic demand RER reflects the shift in substrate utilization (link back to VCO 2) the point where VO2 = VCO2 is when RER = 1. And 100% of the energy used to produce ATP is provided by glucose VO2, VCO2, and VE (volume of inspired air), ATP demand, and Kreb’s cycle respond linearly to the progression of exercise, and blood lactate has an exponential increase. Metabolic response to progressive exercise 1) ATP + H2O → ADP + Pi + H+ + energy (7.3 kcal/molATP) 2) ADP + PCr + H+ (creatine Kinase) ATP + Cr 3) ADP + ADP (adenylate kinase) ATP + AMP 4) AMP (AMP deaminase) IMP + NH3 Response to progressive exercise (all relatively linear): ATP concentration is stable, with only a small decline Phosphocreatine (PCr), large depletion Increase in Creatine The rise in inorganic phosphate (from eq’n 1) ADP and AMP rise but maintain lower concentrations Interstitial metabolite concentrations increase in proportion to exercise intensity Tissue metabolites: during exercise, metabolites are produced in skeletal muscle cells. Some of these metabolites diffuse or are extruded (transported) out of the cell and the concentration of these metabolites increases in the interstitial fluid. *The concentration of metabolites in the ISF is proportional to metabolic rate (i.e. exercise intensity) *The vasodilatory effect is proportional to the concentration of metabolites in the ISF *This couples tissue metabolism to blood flow! A linear increase in the concentration of potassium as intensity increases. (left graph) → since potassium leaves the cell, the more muscles are recruited the more potassium exiting the cell (higher concentration of K in ISF) → will interact and cause relaxation of the vascular smooth muscle, vasodilation, resistance goes down and blood flow goes up. nucleotides also cause vasodilation (adenosine, ATP, ADP, AMP, and blood flow) Interstitial pH will decrease Metabolites that are produced in smooth muscle spill over into the ISF, interact with vascular smooth muscle on arterioles, the vascular smooth muscle relaxes and vasodilation, resistance goes down and blood flow goes up. What drives the increase in VO2? Examining the Fick Equation; Recall the Fick equation: VO2 = CO x (a-v)O2 VO2 = HR x SV x (CaO2 - CvO2) What is the HR response to progressive exercise? A linear increase At the onset of exercise and low to moderate workloads (i.e. from resting HR to ~100 bpm - intrinsic HR) the primary factor responsible for the rise in HR is the withdrawal of PNS stimulation. The Parasympathetic nervous system stimulation is the break HRmax = 220-age or HRmax = 208 - (0.7 x age) - for us it would be 200 From ~100 bpm (intrinsic heart rate) onwards the primary factor that increases HR is the release of NE from the SNS nerves that innervate the SA node. Overlapping on purpose Control of HR - resting membrane potential (Em) The electrical potential (voltage difference) across the cell membrane In a resetting cardiac myocyte, Em is -90mV Determined by: The concentrations of positively (cation) and negatively (anion) charged ions across the cell membrane The relative permeability of the cell membrane of these ions The ion pumps that transport ions across the cell membrane The presence of impermeable, negatively charged proteins within the cell that affect the passive distributions of cations and anions Control of HR - action potentials The electrical event that occurs when the membrane potential suddenly depolarizes (becomes less negative/more positive) and then repolarizes back to its resting state Initiated by changes in permeability and movement of ions across the cell membrane through specific ion channels There are two types of action potentials in cardiac myocytes: 1. Non-pacemaker action potential ○ Typical of most cells in the heart, they are triggered by depolarizing currents from adjacent cells ○ This connection of the cells through gap junctions allowed for this electrical conduction between cells - allows the spread of depolarization 2. Pacemaker action potential ○ Occur in the pacemaker cells of the heart only ○ These are spontaneously-generated action potentials (don’t have to be triggered by external stimuli). Very specialized that go through a continuous cycle of depolarization and repolarization. ○ The primary pacemaker cell concentration and activity is in the Sinoatrial (SA) Node, but there are also some pacemaker cells in the Atrioventricular (AV) Node ○ These control your heart rate and electrical activity of the heart Control of HR - the electrical conduction system of the heart (the pathway for the spread of action potentials to electrically excite the mechanical activity of the heart) 1. Action potentials originate in the sinoatrial (SA) node (the pacemaker) and travel across the wall of the atrium (arrows) from the SA node to the atrioventricular (AV) node. When it arrives at the atrioventricular node, it slows down. This is important because it allows the atria to contract and the electrical activity can pass. 2. Action potentials pass through the AV node and along with the AV bundle, which extends from the AV node, through the fibrous skeleton, into the interventricular septum. 3. The AV bundle divides into right and left bundle branches, and action potentials descend to the apex of each ventricle along the bundle branches. 4. Action potentials are carried by the Purkinje fibers from the bundle branches to the ventricular walls. → Purkinje fibers are specialized cells that have developed for the conduction of action potentials (electrical activity) and they will eventually interact with cardiomyocytes and cause the cardiac muscle cells to depolarize and contract There is an offset between atrial and ventricular contraction which is important for ventricles to completely fill and eject blood NOTE: electrical events happen before mechanical events (there is also a slight delay between the electrical and mechanical event) → The P wave is depolarization of the atrial and atrial contraction. The atria contract before the ventricles contract. → QRS complex if ventricular depolarization and Ventricular contraction → T-wave is ventricular repolarization Control of HR - the autonomic nervous system The intrinsic HR is approx. 100bpm - This is the HR if all the influences of the SA node are removed (i.e. it is the intrinsic rate of the SA nodal cell spontaneous action potential generation when there are no external influences). BUT normal resting heart rate is approx. 60-75bpm and HR can be lowered (during sleep) from normal resting levels or increased to up to 200bpm (i.e. emotional responses or exercise). Parasympathetic is what returns heart to resting heart rate. Terms describing heart rate and changes in heart rate Bradycardia - Low HR at rest (i.e. much lower than 60-75 bpm) Tachycardia - High HR at rest (i.e. > 100 bpm) Positive chronotropy - Any factors that cause HR to increase Negative chronotropy - Any factors that cause HR to decrease Control of HR - The autonomic nervous system (ANS) The ANS has SNS and PNS divisions; most organs receive a dual innervation from both SNS and PNS Antagonistic controls ○ SNS tends to activated organs (the heart to increase heart rate) ○ PNS tends to inhibit organs (the heart to decrease heart rate) Tonic Activity ○ There’s an overlap between SNS and PNS and also some sort of activity there (parasympathetic predominates) - even at rest ○ Not on/off, but the activity of the target organs can be adjusted to increase or decrease ○ The ratio of SNS/PNS activity that determines the net activity of the target organ/tissue Where is this balance/ratio coordinated for the cardiovascular system? The Cardiovascular control center (in the medulla oblongata) is a control center (comparator) for cardiovascular function. This center integrates a number of sensory signals with cardiovascular set points and balances the SNS/PNS output to cause appropriate responses of effector organs (the heart and blood vessels) Control of HR - The autonomic nervous system in cardiovascular control SNS accelerates HR, PNS decelerates HR via influences on the SA node firing rate (and also conduction velocity in the heart) The SNS innervates the SA node, AV node, the conduction system, and the cardiac muscle PNS SIDE OF STORY: PNS carried in Vagus nerve fibers to SA node and AV node (utilizes ACh) Postganglionic PNS fibers use neurotransmitter ACh ACh acts on muscarinic cholinergic receptors on the SA node cells to hyperpolarize SA node cells. Hyperpolarizations leads to a slow down rate of spontaneous action potentials and therefore decrease heart rate At rest, PNS tone is the predominant factor that reduces HR from its intrinsic value of ~100 bpm down to normal resting HR of ~60-75bpm Removing the PNS tone (PNS withdrawal) is the primary factor that elevates HR from resting HR up to ~ 100 bpm (intrinsic rate) SNS SIDE OF STORY: SNS carried in cardiac accelerator nerves to SA and AV nodes and conduction system and cardiac muscle cells Postganglionic SNS fibers use neurotransmitter NE (norepinephrine) NE binding to B-adrenergic receptors causes the rate of the spontaneous depolarization of SA node cells to increase and thus increases heart rate Increases in SNS tone is the predominant factor that elevates HR from ~100bpm (intrinsic rate) to up to HRmax Decreases in SNS tone is the predominant factor that reduces HR from high rates down to ~100bpm The SNS also innervates the cardiac myocytes (cardiac muscle cells) to affect the strength of their contraction (contractility, inotropy) so we can increase cardiac output Stroke Volume response to exercise. What drives the increase in VO2? Examining the Fick equation Recall the Fick equation: VO2 = CO x (a-v)O2 VO2 = HR x SV x (CaO2 - CvO2) What is the SV response to progressive exercise? SV typically plateaus at 40-50% VO2 max → the x-axis is relative to VO2 max (300W is 100%) → with endurance training, this can change Regulation of Stroke Volume VO2 = HR x SV x (CaO2 - CVO2) End diastolic volume (EDV): the volume of blood in the left ventricle at the end of diastole just before contraction (just before systole) 120mL End systolic volume (ESV): the volume of blood in the left ventricle at the end of systole 40 mL ○ There is still some blood left in the left ventricle at the end of the systole Stroke volume (SV) = EDV - ESV Regulation of stroke volume - factors determining SV: Stroke volume (SV) = EDV (preload +) - ESV (afterload +, inotrope/contractility -) + is a direct relationship and - is an indirect relationship 1. Preload: The stretch of the ventricle at the end of the diastole. EDV is a main determinant of preload. As left vertical fills with blood, muscles stretch to allow for that feel - that is preload. Increase EDV = Increase preload = increase SV 2. Afterload: The force/pressure that the ventricle must overcome to cause ejection of blood during systole. Aortic pressure or mean arterial pressure (MAP) is a main determinant of afterload Increase afterload (increase MAP) = increase ESV = decrease SV 3. Inotropy/contractility: The strength of the ventricular contraction during systole. Determined by cellular mechanisms controlling the excitation-contraction coupling process in ventricular myocytes. SNS/PNS activity is a major regulator of contractility. Increase contractility (SNS/PNS) = decrease ESV = increase SV How does preload affect SV? Length-dependent activation (Frank-starling law) of the heart [talking about sarcomere length]. The heart must pump all of the blood that is returned treturnedenous return; VR). Therefore, increasing venous return and thus, ventricular preload, must lead to an increase in SV. intrinsic. Venous Return (VR; the volume of blood returned to the heart by the veins each cardiac cycle) is the major determinant of the EDV (and therefore of the preload) By increasing venous return, you are increasing end-diastolic volume, you also decrease your end-systolic volume and increase SV. (dimensioning return - you start to plateau because you can only fill VR so much) These mechanisms work in the opposite way as well to accommodate decreases in VR, thus decreasing VR and ventricular preload must lead to a decrease in SV Factors regulating venous return (and therefore EDV and therefore preload) during exercise 1. Venoconstriction 2. Skeletal muscle pump 3. Respiratory 1. Venoconstriction (veno = relating to veins); increases venous return NOTE: One of the specialized functions of veins is that they are capacitance vessels - this means that they are well adapted to contain a lot of volumes; i.e. their walls are compliant and the level of basal tone (smooth muscle tension) in the venous smooth muscle is low. At rest, about ⅔ of your blood volume is somewhere in venous circulation (in veins). It is circulating. Venoconstriction will force blood back into the heart Venous Return: the volume of blood returned to the heart by the veins of each cardiac cycle; the major determinant of the EDV (and therefore of the preload) VR → EDV → preload (a stretch of the cardiomyocyte) → force production → SV Work in opposite way as well to accommodate decreases in VR Factors Regulating Venous Return During Exercise Veins are capacitance vessels – well adapted to contain a lot of volumes o Their walls are compliant o Level of basal tone (smooth muscle tension) in the venous smooth muscle is low Venoconstriction increases VR by reducing the volume capacity of the veins to store blood → move blood back toward the heart Occur via a reflex sympathetic constriction of smooth muscle in veins o Controlled by the cardiovascular control center 2. Skeletal Muscle pump - mechanical factor To be clear, vasoconstriction does happen during exercise. The skeletal muscle pump is strictly a mechanical effect associated with skeletal muscle contraction (enhance pressure) and relaxation. Veins have one-way venous valves Blood from tissue capillaries and venules drains into the veins Rhythmic contract/relaxation is critical to the muscle pump. skeletal muscle bulges laterally (if a cell shortens it has to get wider to even out) When skeletal muscle contracts it compresses the veins and forces blood to move towards the heart When the skeletal muscle relaxes the compression is removed, blood draining from the tissue vasculature refills, and veins Thus, during rhythmic dynamic exercise (repeated contraction/relaxation cycles), the skeletal muscle pump enhances VR, while during isometric contractions the muscle pump does not function and venous return (VR) is reduced. 3. Respiratory pump - mechanical factor A very important factor that increases venous return during exercise Inspiration: ○ Lowers thoracic pressure (your ribs in your thoracic cavity expands) ○ Elevates abdominal pressure (diaphragm contract and moves down to the abdomen and applies pressure to it - elevates abdominal pressure) ○ The compartment pressure gradient during inspiration helps to pump venous blood from the abdomen into the thorax to increase Venous return (VR) ○ This effect is present at rest but is greatly enhanced during dynamic exercise, as respiratory rate and depth of breathing are both increased in proportion to exercise intensity. How does afterload (pressure in aorta needed to eject blood) affect SV? Aortic or MAP is a barrier to the ejection of blood from the left ventricle-sufficient pressure must be generated in the ventricle to overcome the MAP and cause ejection. When you manipulate afterload, you are causing a shift in the graph ○ If you decrease afterload, you are enhancing the amount of blood ejected (stroke volume). AN INVERSE relationship. ○ Changes in afterload shift the SV vs EDV (preload) curve up or down. Degree of curve shift scales with degree of afterload change ○ *Note* During dynamic exercise (cycling), large declines in peripheral resistance due to arterial dilation in skeletal muscle percent large increases in MAP and afterloading from occurring. This makes it possible for the heart to pump the cardiac output necessary to support the O2 demands of the body. Overcome the increase in MAP. How does inotropy/contractility affect SV? Cardiac myocytes contain numerous calcium regulatory proteins (affect the levels of Ca 2+), and numerous regulatory sites on contractile proteins that control the force generated during contraction (these are all cellular sites of inotropy control) If you release Ca you increase action Many of these proteins are sites that are regulated by signalling mechanisms under the control of B adrenergic receptor system (within ventricular myocytes) ○ Via this mechanism, SNS activation and/or circulating epinephrine and norepinephrine (catecholamine hormones) cause increases in contractility ○ This results in the shifting of the curve (during exercise) it scales with degree of SNS stimulation. ○ MAP doesn't change too much during exercise Summary of the effects of muscle pump, respiratory pump and venoconstriction of EDV LV filling is enhanced during progressive exercise by the muscle pump, respiratory pump and venoconstriction. Despite a progressive reduction in the time spent in diastole, we ehenace fill!! As HR increases, cardiac cycle duration increases. Ejection fraction ○ Healthy is greater than or equal to 50% at rest Recall: SV regulation ○ Inotropy/contractility - during progressive exercise SNS activity increases (blood Epi and NE response). The strength of contraction increases. ○ This is important because afterload (MAP) increases during progressive exercise. The pressure the LV must generate to eject blood increases so it is a good thing the inotropy increases ○ Ejection fraction (EF) actually increases despite increased afterload. What drives the increase in VO2? Examining the Fick equation VO2 = CO x (a-v)O2 5 L/min is normal resting cardiac output 20-25 L/min is a normal maximal cardiac output There is redistribution of cardiac output from rest to heavy exercise because we need blood to the more active muscles Kidneys, brain, skeletal muscles and sp-something region: 4 major users of O 2 and require most blood flow at rest Same absolute values during exercise but the rest of the 25% goes to active muscle Blood flow to active skeletal muscle (percentage of cardiac output) is increased in proportion to exercise intensity and attempts to match the demand for oxygen and substrates (glucose and FFAs) and to match removal of waste products (carbon dioxide, H, lactate, heat, etc) to waste production → If you can enhance your maximal cardiac output you will enhance your VO 2 max. Cardiac output influence VO the most Blood pressure response to progressie exercise Normal resting BPs: Solid read is absolute values and shading is stresses that is relative SBP = 120 mmHg DBP = 80 mmHg MAP = 90mmHg Relatively speaking: ○ SBP increases the most: 120 to 200mmHg ○ MAP may increase ~20-30% during maximal dynamic exercise ○ DBP will typically increase slightly (5-10 mmHg) during a progressive exercise test on a bike. However, it could remain the same or it could decrease slightly. Progressive tests performed on a treadmill typically cause a drop in DBP. Doesn’t change much because of the substantial drop in total peripheral resistance. Pulmonary system Ventilation and gas transport in the blood VO2 = HR x SV x (CaO2 - CvO2) Atrial O2 content is determined by ventilation, respiration,, hemoglobin (Hb) concentration and Hb saturation. Venous O2 content is dependent on metabolic rate of the tissues and blood flow to the tissue Respiratory Musculature that the diaphragm is responsible for inspiration, so it will contract and it will pull down. And cause the thoracic cavity to expand and that will participate in inspiration At rest, expiration is passive; diaphragm will relax. We also have accessory muscles that we can rely on to facilitate the expansion or return to the normal size of the thoracic cavity (depth of breathing responsibility from depth and frequency of breathing.) Mechanics of ventilation - changes in volume and pressure drive ventilation Pulmonary - structure and function We have a conducting zone and the respiratory zone Conducting zone (Trachea → bronchi → bronchioles → terminal bronchioles) ○ Trachea and Bronchi are rigid tubes cartilage - protects structures and helps maintain their shape ○ Bronchioles are soft, dynamic tubes These are similar to arterioles Smooth muscle; dilated during expiration because of SNS stimulation - decreases resistance to air flow ○ 4 primary functions - conveys air to alveoli, warms air, humidifies air and filters air. Adds moisture for the alveoli to stay well Respiratory zone (Respiratory bronchioles → alveolar ducts → alveolar sacs) ○ Respiratory bronchioles have sporadic alveoli ○ Alveoli - site of gas exchange Uptake of O2 from the air in the lungs by the pulmonary capillary blood We have an alveolar-capillary unit Capillary has a fixed length. Start of a capillary is on the arterial side (low in oxygen; from the right side of the heart) and end is on venous side (rich in oxygen) There's an exchange is gas occurs as a RBC travels through it PO2 or partial pressure of oxygen is low in the pulmonary capillary is around 40 mmHg PAO2 or partial pressure of Alveolar oxygen is around 104mmHg When a unit of blood arrives at the beginning of the capillary, it has the partial pressure of 40 mmHg and the partial pressure of the alveolus is 104mmHg. Large pressure gradient that causes diffusion When you travel down the length of capillary the PO 2 in blood increases until eventually reaching equilibrium between the alveolus and capillary Exchange happens early on down the length of the capillary If you want to get 25 L/min of blood in the pulmonary circulation (so heavy exercise), the velocity of blood flow must increase As Cardiac output goes up: The length of the tubes is constant, if we want to get more volume we need to increase velocity of blood The equilibration point moves further down the pulmonary capillary due to a decrease in RBC transit time because there is less time for diffusion to occur More alveolar-capillary units are required to ensure full equilibration between alveolar air and blood PO2 There are condition when equilibration is not 100% (during heavy exercise) Changes in PO2 throughout the pulmonary and systemic circulations PTO2 = Tissue partial pressure ○ Oxygen delivery to the tissue (blood flow) ○ Oxygen utilization (mitochondrial O2 consumption in the tissue) Systemic capillary is the exact opposite to pulmonary capillary in parietal pressure As blood flows through systemic capillaries, PO 2 in the blood equilibrates (by diffusion) with the tissue PO2 (PTO2; normally ~40 mmHg, but can be decreased in activate muscles during exercise) Components of Blood Plasma: Liquid portion of the blood (i.e. no cells). Contain ion, protein, glucose, fatty acids, etc. ○ 2-3% of the oxygen carried in the blood is dissolved in the plasma. ~97-98% of the oxygen in the blood is carried bound to hemoglobin in RBC Cellular components: ○ White blood cells (WBC): is important for immunity and prevention of infection ○ Platelets: are important for blood clotting ○ Red blood cells (RBC) or Erythrocytes Plasma ~58%: Water, proteins, nutrients, hormones, etc… Buffy coat 100 million Hb molecules in 1 red blood cell (RBC) and there are 20-30 trillion RBCs in your body! Normal oxygen saturation (SO2) is 96-98% in arterial blood What is the oxygen carrying capacity of the blood? O2-carrying capacity of the blood: The maximum volume of O2 that a volume of blood can carry at FULL SATURATION (i.e. SO2 = 100%) Since saturation is 100%, carrying capacity depends on [Hb] = ○ 15g Hb/100mL blood males ○ 12.5 g Hb/100mL blood females Each hemoglobin molecule can bind a maximum of 4 O2 molecules At full saturation of Hb with O2. this amounts to 1.34 mL O2/g Hb (constant value) So, maximal O2-carrying capacity of the blood = 20 mL O2/100mL blood If [Hb] changes, the O2-carrying capacity of the blood will change What is the oxygen content of the blood? O2- content of the blood: The volume of O2 carried in each volume of blood under given conditions CaO2 depends on carrying capacity ([Hb]); PO 2 that the blood is exposed to and thus, SO2 O2-content = carrying capacity x saturation Arterial oxygen content (CaO2), assume that the partial pressure of oxygen in arterial blood (PaO2) is 100 mmHg, which results in a SO2 of approx 97% Therefore: CaO2 = 20 mL O2/100 mL blood x 0.97 = 19.5 mL O2/100 mL blood Venous oxygen content (CvO2), assume that the partial pressure of oxygen in venous blood (PvO2) is 40 mmHg, which results in a SO2 of approx 72% Therefore: CvO2 = 20 mL O2/100 mL blood x 0.72 = 14.5 mL O2/100 mL blood The O2-Hemoglobin dissociation curve Sigmoidal response Changes in aterial and venous PO2 and (CaO2 - CvO2) during progressive exercisesv