KİMYA PDF PDF
Document Details
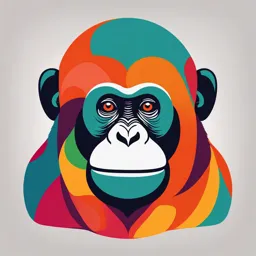
Uploaded by WarmSard8224
Kocaeli University
Tags
Summary
This is a textbook on general chemistry, covering topics such as matter, properties, the scientific method, and chemical calculations. It's an introductory text, including sections on SI units and atomic theory.
Full Transcript
Introduction Reference: General Chemistry Principles and Modern Applications TENTH EDITION, Pearson Canada Toronto Matter-Its Properties and Measurement The scope of Chemistry The Scientific Method Poperties of Matter Classification of Matter Measurement of Matter (SI Units) ...
Introduction Reference: General Chemistry Principles and Modern Applications TENTH EDITION, Pearson Canada Toronto Matter-Its Properties and Measurement The scope of Chemistry The Scientific Method Poperties of Matter Classification of Matter Measurement of Matter (SI Units) The Scope of Chemistry Everything is made up of chemicals, and much of what we do with things involve chemical reactions. The gasoline that fules our automobiles is a mixture of different chemicals. The burning of this mixture provides the energy that propels the automobile. Chemistry is sometimes called the «cental science» because it relates to many areas of human endeavor and curiosity. Chemicals who develop new materials to improve electronic devices such as; solar cells, transistors, fiber optic cables work at the interfaces of chemistry with physics and engineering. The Scientific Method Originated in 17th century with such people as Galileo, Francis Bacon, Isaac Newton. The scientific method is the combination of observations, experimentation and the formulations of laws, hypotheses and theories. Many discoveries (X-Ray, radioactivity, penicilin) have been made by accident. Such chance discoveries are referred to serendipity. Properties of Matter Matter is anything that occupies space, displays a property known as mass and possesses inertia. Composition refers to the parts or components of a sample of matter and their relative proportions. Ordinary water is made up of two simpler substances ; hydrogen and oxygen. A chemist would say that the composition of water is 11.19% hydrogen and 88.81% oxygen by mass. Properties of matter are generally grouped into two broad categories: pyhsical and chemical. A physical property is one that a sample of mater displays without changing its composition. Copper can be hammered into thin sheet or foil. When liquid water freezes into solid water (ice), it certainly looks different in many ways it is different. But , it remains 11.19% hydrogen and 88.81% oxygen by mass. In a chemical change or chemical reaction, one or more samples of matter are converted to new samples with different compositions. The key to identifying chemical change, then, comes in observing a change in composition. The burning of paper involves a chemical change. Paper is a complex material, but its principal components are carbon, hydrogen and oxygen. The chief products of the combustion are two gases, carbon dioxide and water as stream. Classification of Matter Matter is built up from very tiny unıts called atoms. A chemical element is a substance made up of only a single type of atom (118 known elements) Chemical compounds are substances in which atoms of different elements are combined with one another. (millions of different chemical compunds) A molecule is the smallest entitiy having the same proportions of the constituent atoms. Homegeneous mixtures are uniform in compositions and properties throughout a given sample, but the composition and properties may vary from one sample to another. (Seawater, cane sugar in water) Measurement of Matter : SI Units Atoms and Atomic Theory Reference: General Chemistry Principles and Modern Applications TENTH EDITION, Pearson Canada Toronto Law of Conservation of Mass The process of burning is so familiar it is hard to realize what a difficult riddle this posed for early scientists. In 1774, Antoine Lavoisier performed an experiment in which he heated a sealded glass vessel containing a sample of tin and some air. He found that the mass before heating (glass vessel+tin+air) and after heating (glass vessel+tin calx+remaining air) were the same. The total mass of substances present after a chemical reaction is the same as the total mass of substances before the reaction. Stated another way, this law says that matter can neither be created nor destroyed in a chemical reaction. Law of Constant Composition In 1799, Joseph Proust reported one hundred ponds of copper dissolved in sulfuric or nitric acids and precipitated by carbonates of soda, invariably gives 10 pounds of green carbonate. All samples of a compound have the same composition-the same proportions by mass of the constituent elements. Daltons Atomic Theory Describes the basis of atomic theory with three assumptions: 1) Each element is composed of small particles called atoms. Atoms are neither created nor destroyed in chemical reactions. 2) All atoms of a given element are identical but atoms of one element are different from those off all other elements. 3) Compounds are formed when the atoms of more than one element combine in mol numerical ratios. Electrons and Other discoveries in Atomic Physics Electricity snd magnetism were used in the experiments so that led to the current theory of atomic structure Certain objects display properties called electric charge, which can be either positive (+) or negative (-) An object having equal numver of (+) or (-) charged particles carries no net charge and is electrically neutral If the number or (+) charge exceed the number of (-) charge, the object has a net positive charge. If the number of (-) charge exceed the number of (+) charge, the object has a negative charge. (+) and (-) charges attract each other, while two (+) and two (-) charges reperl each other. (a)Electrostatically charged comb. If you comb your hair the static charge develop on the comb and causes bits of paper to be attracted to the comb. (b) Both object on the left carry negative charge repel each other. The objects in the center lack any electrical charge and exert no force on each other. The objects on the right carry opposite charges and attract each other. Atoms and Atomic Theory Reference: General Chemistry Principles and Modern Applications TENTH EDITION, Pearson Canada Toronto Law of Conservation of Mass The process of burning is so familiar it is hard to realize what a difficult riddle this posed for early scientists. In 1774, Antoine Lavoisier performed an experiment in which he heated a sealded glass vessel containing a sample of tin and some air. He found that the mass before heating (glass vessel+tin+air) and after heating (glass vessel+tin calx+remaining air) were the same. The total mass of substances present after a chemical reaction is the same as the total mass of substances before the reaction. Stated another way, this law says that matter can neither be created nor destroyed in a chemical reaction. Law of Constant Composition In 1799, Joseph Proust reported one hundred ponds of copper dissolved in sulfuric or nitric acids and precipitated by carbonates of soda, invariably gives 10 pounds of green carbonate. All samples of a compound have the same composition-the same proportions by mass of the constituent elements. Daltons Atomic Theory Describes the basis of atomic theory with three assumptions: 1) Each element is composed of small particles called atoms. Atoms are neither created nor destroyed in chemical reactions. 2) All atoms of a given element are identical but atoms of one element are different from those off all other elements. 3) Compounds are formed when the atoms of more than one element combine in mol numerical ratios. Electrons and Other discoveries in Atomic Physics Electricity snd magnetism were used in the experiments so that led to the current theory of atomic structure Certain objects display properties called electric charge, which can be either positive (+) or negative (-) An object having equal numver of (+) or (-) charged particles carries no net charge and is electrically neutral If the number or (+) charge exceed the number of (-) charge, the object has a net positive charge. If the number of (-) charge exceed the number of (+) charge, the object has a negative charge. (+) and (-) charges attract each other, while two (+) and two (-) charges reperl each other. (a)Electrostatically charged comb. If you comb your hair the static charge develop on the comb and causes bits of paper to be attracted to the comb. (b) Both object on the left carry negative charge repel each other. The objects in the center lack any electrical charge and exert no force on each other. The objects on the right carry opposite charges and attract each other. Chemical Calculations References : 1. General Chemistry- principles and modern applications (Petrucci, Herring, Madura, Bissonnette) 2. Chemistry 10th Edition (Raymond Chang) Measurement of Matter: SI (Metric) Units Chemistry is a quantitative science, which means that in many cases we can measure a property of a substance and compare it with a standard having a known value of the property. The scientific system of measurement is called the Système Internationale d Unités (International System of Units) and is abbreviated SI. SI Base Quantity Physical quantity Unit Length metre, m Mass kilogram, kg Time second, s Temperature kelvin, K Amount of substance mole, mol Electric current ampere, A Luminous intensity candela, cd Most measurements in chemistry are made in SI units. Sometimes we must convert between SI units, as when converting kilometers to meters. At other times we must convert measurements expressed in non-SI units into SI units, or from SI units into non-SI units. In all of these cases we can use a conversion factor or a series of conversion factors in a scheme called a conversion pathway. To go from one unit to another, we need a conversion factor. We need 3 important pieces of information: Desired amount and units Initial amount and units Conversion factor The general form of a unit conversion calculation is: (desired amount)= (initial amount) x (conversion factor) Mass Mass describes the quantity of matter in an object. In SI the standard of mass is 1 kilogram (kg), which is a fairly large unit for most applications in chemistry. More commonly we use the unit gram (g) (about the mass of three aspirin tablets). Weight is the force of gravity on an object. It is directly proportional to mass, as shown in the following mathematical expressions. An object has a fixed mass (m), which is independent of where or how the mass is measured. Its weight (W), however, may vary because the acceleration due to gravity (g) varies slightly from one point on Earth to another. Density Density is the ratio of mass to volume. Mass and volume are both extensive properties. An extensive property is dependent on the quantity of matter observed. However, if we divide the mass of a substance by its volume, we obtain density, an intensive property. An intensive property is independent of the amount of matter observed. Thus, the density of pure water at 25 °C has a unique value, whether the sample fills a small beaker (small mass/small volume) or a swimming pool (large mass/large volume). Intensive properties are especially useful in chemical studies because they can often be used to identify substances. The SI base units of mass and volume are kilograms and cubic meters, respectively, but chemists generally express mass in grams and volume in cubic centimeters or milliliters. Thus, the most commonly encountered density unit is grams per cubic centimeter (g/cm3) or the identical unit grams per milliliter (g/mL). The Concept of the Mole and the Avogadro Constant The SI quantity that describes an amount of substance by relating it to a number of particles of that substance is called the mole (abbreviated mol). A mole is the amount of a substance that contains the same number of elementary entities as there are atoms in exactly 12 g of pure carbon-12. This definition carefully avoids saying that the entities to be counted are always atoms. As a result, we can apply the concept of a mole to any quantity that we can represent by a symbol or formula—atoms, ions, formula units, or molecules. The “number of elementary entities (atoms, molecules, and so on)” in a mole is the Avogadro constant, NA. Composition of Chemical Compounds A chemical formula conveys considerable quantitative information about a compound and its constituent elements. We have already learned how to determine the molar mass of a compound, and, in this section, we consider some other types of calculations based on the chemical formula. The colorless, volatile liquid halothane has been used as a fire extinguisher and also as an inhalation anesthetic. Both its empirical and molecular formulas are C2HBrCIF3 ,its molecular mass is 197.38 u, and its molar mass is as calculated below: The molecular formula of C2HBrCIF3 tells us that per mole of halothane there are two moles of C atoms, one mole each of H, Br, and Cl atoms, and three moles of F atoms. This factual statement can be turned into conversion factors to answer such questions as, “How many C atoms are present per mole of halothane?” In this case, the factor needed is 2 mol/ mol C2HBrCIF3. That is, Calculating Percent Composition from a Chemical Formula When chemists believe that they have synthesized a new compound, a sample is generally sent to an analytical laboratory where its percent composition is determined. This experimentally determined percent composition is then compared with the percent composition calculated from the formula of the expected compound. In this way, chemists can see if the compound obtained could be the one expected. Equation (3.1) establishes how the mass percent of an element in a compound is calculated. In applying the equation, think in terms of the following steps. 1. Determine the molar mass of the compound. This is the denominator in equation (3.1). 2. Determine the contribution of the given element to the molar mass. This product of the formula subscript and the molar mass of the element appears in the numerator of equation (3.1). 3. Formulate the ratio of the mass of the given element to the mass of the compound as a whole. This is the ratio of the numerator from step 2 to the denominator from step 1. 4. Multiply this ratio by 100% to obtain the mass percent of the element. Establishing Formulas from the Experimentally Determined Percent Composition of Compounds At times, a chemist isolates a chemical compound—say, from an exotic tropical plant—and has no idea what it is. A report from an analytical laboratory on the percent composition of the compound yields data needed to determine its formula. Percent composition establishes the relative proportions of the elements in a compound on a mass basis. A chemical formula requires these proportions to be on a mole basis, that is, in terms of numbers of atoms. Chemical Calculations References : 1.General Chemistry- principles and modern applications (Petrucci, Herring, Madura, Bissonnette) 2. Chemistry 10th Edition (Raymond Chang) Measurement of Matter: SI (Metric) Units Chemistry is a quantitative science, which means that in many cases we can measure a property of a substance and compare it with a standard having a known value of the property. The scientific system of measurement is called the Système Internationale d Unités (International System of Units) and is abbreviated SI. SI Base Quantity Physical quantity Unit Length metre, m Mass kilogram, kg Time second, s Temperature kelvin, K Amount of substance mole, mol Electric current ampere, A Luminous intensity candela, cd Most measurements in chemistry are made in SI units. Sometimes we must convert between SI units, as when converting kilometers to meters. At other times we must convert measurements expressed in non-SI units into SI units, or from SI units into non-SI units. In all of these cases we can use a conversion factor or a series of conversion factors in a scheme called a conversion pathway. To go from one unit to another, we need a conversion factor. We need 3 important pieces of information: Desired amount and units Initial amount and units Conversion factor The general form of a unit conversion calculation is: (desired amount)= (initial amount) x (conversion factor) Mass Mass describes the quantity of matter in an object. In SI the standard of mass is 1 kilogram (kg), which is a fairly large unit for most applications in chemistry. More commonly we use the unit gram (g) (about the mass of three aspirin tablets). Weight is the force of gravity on an object. It is directly proportional to mass, as shown in the following mathematical expressions. An object has a fixed mass (m), which is independent of where or how the mass is measured. Its weight (W), however, may vary because the acceleration due to gravity (g) varies slightly from one point on Earth to another. Density Density is the ratio of mass to volume. Mass and volume are both extensive properties. An extensive property is dependent on the quantity of matter observed. However, if we divide the mass of a substance by its volume, we obtain density, an intensive property. An intensive property is independent of the amount of matter observed. Thus, the density of pure water at 25 °C has a unique value, whether the sample fills a small beaker (small mass/small volume) or a swimming pool (large mass/large volume). Intensive properties are especially useful in chemical studies because they can often be used to identify substances. The SI base units of mass and volume are kilograms and cubic meters, respectively, but chemists generally express mass in grams and volume in cubic centimeters or milliliters. Thus, the most commonly encountered density unit is grams per cubic centimeter (g/cm3) or the identical unit grams per milliliter (g/mL). The Concept of the Mole and the Avogadro Constant The SI quantity that describes an amount of substance by relating it to a number of particles of that substance is called the mole (abbreviated mol). A mole is the amount of a substance that contains the same number of elementary entities as there are atoms in exactly 12 g of pure carbon-12. This definition carefully avoids saying that the entities to be counted are always atoms. As a result, we can apply the concept of a mole to any quantity that we can represent by a symbol or formula—atoms, ions, formula units, or molecules. The “number of elementary entities (atoms, molecules, and so on)” in a mole is the Avogadro constant, NA. Composition of Chemical Compounds A chemical formula conveys considerable quantitative information about a compound and its constituent elements. We have already learned how to determine the molar mass of a compound, and, in this section, we consider some other types of calculations based on the chemical formula. The colorless, volatile liquid halothane has been used as a fire extinguisher and also as an inhalation anesthetic. Both its empirical and molecular formulas are C2HBrCIF3 ,its molecular mass is 197.38 u, and its molar mass is as calculated below: The molecular formula of C2HBrCIF3 tells us that per mole of halothane there are two moles of C atoms, one mole each of H, Br, and Cl atoms, and three moles of F atoms. This factual statement can be turned into conversion factors to answer such questions as, “How many C atoms are present per mole of halothane?” In this case, the factor needed is 2 mol/ mol C2HBrCIF3. That is, Calculating Percent Composition from a Chemical Formula When chemists believe that they have synthesized a new compound, a sample is generally sent to an analytical laboratory where its percent composition is determined. This experimentally determined percent composition is then compared with the percent composition calculated from the formula of the expected compound. In this way, chemists can see if the compound obtained could be the one expected. Equation (3.1) establishes how the mass percent of an element in a compound is calculated. In applying the equation, think in terms of the following steps. 1. Determine the molar mass of the compound. This is the denominator in equation (3.1). 2. Determine the contribution of the given element to the molar mass. This product of the formula subscript and the molar mass of the element appears in the numerator of equation (3.1). 3. Formulate the ratio of the mass of the given element to the mass of the compound as a whole. This is the ratio of the numerator from step 2 to the denominator from step 1. 4. Multiply this ratio by 100% to obtain the mass percent of the element. Establishing Formulas from the Experimentally Determined Percent Composition of Compounds At times, a chemist isolates a chemical compound—say, from an exotic tropical plant—and has no idea what it is. A report from an analytical laboratory on the percent composition of the compound yields data needed to determine its formula. Percent composition establishes the relative proportions of the elements in a compound on a mass basis. A chemical formula requires these proportions to be on a mole basis, that is, in terms of numbers of atoms. Introduction to the Periodic Table References : 1. General Chemistry- principles and modern applications (Petrucci, Herring, Madura, Bissonnette) 2. Chemistry-10th Edition (Raymond Chang) More than half of the elements known today were discovered between 1800 and 1900. During this period, chemists noted that many elements show strong similarities to one another. Recognition of periodic regularities in physical and chemical behavior and the need to organize the large volume of available information about the structure and properties of elemental substances led to the development of the periodic table, a chart in which elements having similar chemical and physical properties are grouped together. Figure shows the modern periodic table in which the elements are arranged by atomic number (shown above the element symbol) in horizontal rows called periods and in vertical columns known as groups or families, according to similarities in their chemical properties. Note that elements 112–116 and 118 have recently been synthesized, although they have not yet been named. In 1869, Dmitri Mendeleev and Lothar Meyer independently proposed the periodic law: When the elements are arranged in order of increasing atomic mass, certain sets of properties recur periodically. Features of the Periodic Table In the periodic table, elements are listed according to increasing atomic number starting at the upper left and arranged in a series of horizontal rows. This arrangement places similar elements in vertical groups or families. For example, sodium and potassium are found together in a group labeled 1 (called the alkali metals). We should expect other members of the group, such as cesium and rubidium, to have properties similar to sodium and potassium. Chlorine is found at the other end of the table in a group labeled 17. Each element is listed in the periodic table by placing its symbol in the middle of a box in the table. The atomic number (Z) of the element is shown above the symbol, and the weighted- average atomic mass of the element is shown below its symbol. Some periodic tables provide other information, such as density and melting point, but the atomic number and atomic mass are generally sufficient for our needs. Elements with atomic masses in parentheses, such as plutonium, Pu (244), are produced synthetically, and the number shown is the mass number of the most stable isotope. It is customary also to divide the elements into two broad categories—metals and nonmetals. In Figure, colored backgrounds are used to distinguish the metals (tan) from the nonmetals (blue and pink). Except for mercury, a liquid, metals are solids at room temperature. They are generally malleable (capable of being flattened into thin sheets), ductile (capable of being drawn into fine wires), good conductors of heat and electricity, and have a lustrous or shiny appearance. The properties of nonmetals are generally opposite those of metals; for example, nonmetals are poor conductors of heat and electricity. Several of the nonmetals, such as nitrogen, oxygen, and chlorine, are gases at room temperature. Some, such as silicon and sulfur, are brittle solids. One—bromine—is a liquid. Two other highlighted categories in Figure are a special group of nonmetals known as the noble gases (pink), and a small group of elements, often called metalloids (green), that have some metallic and some nonmetallic properties. Periodic Variation in Physical Properties Introduction to the Periodic Table References : 1. General Chemistry- principles and modern applications (Petrucci, Herring, Madura, Bissonnette) 2. Chemistry-10th Edition (Raymond Chang) More than half of the elements known today were discovered between 1800 and 1900. During this period, chemists noted that many elements show strong similarities to one another. Recognition of periodic regularities in physical and chemical behavior and the need to organize the large volume of available information about the structure and properties of elemental substances led to the development of the periodic table, a chart in which elements having similar chemical and physical properties are grouped together. Figure shows the modern periodic table in which the elements are arranged by atomic number (shown above the element symbol) in horizontal rows called periods and in vertical columns known as groups or families, according to similarities in their chemical properties. Note that elements 112–116 and 118 have recently been synthesized, although they have not yet been named. In 1869, Dmitri Mendeleev and Lothar Meyer independently proposed the periodic law: When the elements are arranged in order of increasing atomic mass, certain sets of properties recur periodically. Features of the Periodic Table In the periodic table, elements are listed according to increasing atomic number starting at the upper left and arranged in a series of horizontal rows. This arrangement places similar elements in vertical groups or families. For example, sodium and potassium are found together in a group labeled 1 (called the alkali metals). We should expect other members of the group, such as cesium and rubidium, to have properties similar to sodium and potassium. Chlorine is found at the other end of the table in a group labeled 17. Each element is listed in the periodic table by placing its symbol in the middle of a box in the table. The atomic number (Z) of the element is shown above the symbol, and the weighted- average atomic mass of the element is shown below its symbol. Some periodic tables provide other information, such as density and melting point, but the atomic number and atomic mass are generally sufficient for our needs. Elements with atomic masses in parentheses, such as plutonium, Pu (244), are produced synthetically, and the number shown is the mass number of the most stable isotope. It is customary also to divide the elements into two broad categories—metals and nonmetals. In Figure, colored backgrounds are used to distinguish the metals (tan) from the nonmetals (blue and pink). Except for mercury, a liquid, metals are solids at room temperature. They are generally malleable (capable of being flattened into thin sheets), ductile (capable of being drawn into fine wires), good conductors of heat and electricity, and have a lustrous or shiny appearance. The properties of nonmetals are generally opposite those of metals; for example, nonmetals are poor conductors of heat and electricity. Several of the nonmetals, such as nitrogen, oxygen, and chlorine, are gases at room temperature. Some, such as silicon and sulfur, are brittle solids. One—bromine—is a liquid. Two other highlighted categories in Figure are a special group of nonmetals known as the noble gases (pink), and a small group of elements, often called metalloids (green), that have some metallic and some nonmetallic properties. Periodic Variation in Physical Properties Chemical Bondings Reference: General Chemistry Principles and Modern Applications TENTH EDITION, Pearson Canada Toronto Contents Lewis Theory: An Overview Covalent Bonding: An Introduction Polar Covalent Bonds and Electrostatic Potential Maps Writing Lewis Structures Some fundamental ideas associated with Lewis s theory follow: 1. Electrons, especially those of the outermost (valence) electronic shell, playa fundamental role in chemical bonding. 2. In some cases, electrons are transferred from one atom to another. Positive and negative ions are formed and attract each other through electrostatic forces called ionic bonds. 3. In other cases, one or more pairs of electrons are shared between atoms. A bond formed by the sharing of electrons between atoms is called a covalent bond. 4. Electrons are transferred or shared in such a way that each atom acquires an especially stable electron configuration. Usually this is a noble gas configuration, one with eight outer-shell electrons, or an octet. Lewis symbols and Lewis structure A Lewis symbol consists of a chemical symbol to represent the nucleus and core (inner-shell) electrons of an atom, together with dots placed around the symbol to represent the valence (outer-shell) electrons. the Lewis symbol for silicon, which has the electron configuration [Ne]3s23p6, is We will write Lewis symbols in the way Lewis did. We will place single dots on the sides of the symbol, up to a maximum of four. Then we will pair up dots until we reach an octet. Lewis symbols are commonly written for main-group elements but much less often for transition elements. A Lewis structure is a combination of Lewis symbols that represents either the transfer or the sharing of electrons in a chemical bond IONic Bonding electrons are transferred between valence shells of atoms ionic compounds are made of ions NOT MOLECULES ionic compounds are called Salts or Crystals IONic Bonding Electronegativity difference > 2.0 Look up e-neg of the atoms in the bond and subtract NaCl CaCl2 Compounds with polyatomic ions NaNO3 Properties of Ionic Compounds SALTS hard solid @ 22oC Crystals high mp temperatures nonconductors of electricity in solid phase good conductors in liquid phase or dissolved in water (aq) Covalent Bonding molecules Pairs of e- are shared between non-metal atoms electronegativity difference < 2.0 forms polyatomic ions Covalent Bonding The broken circles represent the outermost electron shells of the bonded atoms. The number of dots lying on or within each circle represents the effective number of electrons in each valence shell. The H atom has two dots, as in the electron configuration of He. The Cl atom has eight dots, corresponding to the outershell configuration of Ar. Note that we counted the two electrons between H and Cl twice. These two electrons are shared by the H and Cl atoms. This shared pair of electrons constitutes the covalent bond. As was the case for Cl in HCl, the O atom in the Lewis structure of H2O and Cl2O in is surrounded by eight electrons (when the bond-pair electrons are double counted). In attaining these eight electrons, the O atom conforms to the octet rule a requirement of eight valence- shell electrons for the atoms in a Lewis structure. Note, however, that the H atom is an exception to this rule. The H atom can accommodate only two valence-shell electrons. The sharing of a single pair of electrons between bonded atoms produces a single covalent bond. To underscore the importance of electron pairs in the Lewis theory the term bond pair applies to a pair of electrons in a covalent bond, while lone pair applies to electron pairs that are not involved in bonding. Also, in writing Lewis structures it is customary to replace bond pairs with lines ( ). These features are shown in the following Lewis structures. The bond formed between the N atom of and the ion in structure is a coordinate covalent bond. It is important to note, however, that once the bond has formed, it is impossible to say which of the four bonds is the coordinate covalent bond. Thus, a coordinate covalent bond is indistinguishable from a regular covalent bond. Multiple Covalent Bonds More than one pair of electrons must be shared if an atom is to attain an octet (noble gas electron configuration). CO2 and N2 are two molecules in which atoms share more than one pair of electrons. C atom can share a valence electron with each O atom, thus forming two carbon-to-oxygen single bonds. Lewis structure for the molecule. Our first attempt might again involve a single covalent bond and the incorrect structure shown below: Each N atom appears to have only six outer-shell electrons, not the expected eight. The situation can be corrected by bringing the four unpaired electrons into the region between the N atoms and using them for additional bond pairs. In all, we now show the sharing of three pairs of electrons between the N atoms. Polar Covalent Bonds A covalent bond in which electrons are not shared equally between two atoms is called a polar covalent bond. The unequal sharing of the electrons leads to a partial negative charge on the more nonmetallic element, signified by and a corresponding partial positive charge on the more metallic element, designated by Electronegativity Electronegativity (EN) describes an atom s ability to compete for electrons with other atoms to which it is bonded. As such, electronegativity is related to ionization energy (I) and electron affinity (EA). Writing Lewis Structures All the valence electrons of the atoms in a Lewis structure must appear in the structure. Usually, all the electrons in a Lewis structure are paired. Usually, each atom acquires an outer-shell octet of electrons. Hydrogen, however, is limited to two outer-shell electrons. Sometimes, multiple covalent bonds (double or triple bonds) are needed. Multiple covalent bonds are formed most readily by C, N, O, P, and S atoms. Skeletal structure all the atoms in the structure arranged in the order in which they are bonded to one another. In a skeletal structure with more than two atoms, we generally need to distinguish between central and terminal atoms. A central atom is bonded to two or more atoms, and a terminal atom is bonded to just one other atom. Hydrogen atoms are always terminal atoms. This is because an H atom can accommodate only two electrons in its valence shell, so it can form only one bond to another atom. Central atoms are generally those with the lowest electronegativity. Carbon atoms are always central atoms. Molecules and polyatomic ions generally have compact, symmetrical structures Resonance When we apply the usual rules for Lewis structures for ozone, we come up with these two possibilities. The situation in which two or more plausible Lewis structures contribute to the correct structure is called resonance. Chemical Bondings Reference: General Chemistry Principles and Modern Applications TENTH EDITION, Pearson Canada Toronto Contents Lewis Theory: An Overview Covalent Bonding: An Introduction Polar Covalent Bonds and Electrostatic Potential Maps Writing Lewis Structures Some fundamental ideas associated with Lewis s theory follow: 1. Electrons, especially those of the outermost (valence) electronic shell, playa fundamental role in chemical bonding. 2. In some cases, electrons are transferred from one atom to another. Positive and negative ions are formed and attract each other through electrostatic forces called ionic bonds. 3. In other cases, one or more pairs of electrons are shared between atoms. A bond formed by the sharing of electrons between atoms is called a covalent bond. 4. Electrons are transferred or shared in such a way that each atom acquires an especially stable electron configuration. Usually this is a noble gas configuration, one with eight outer-shell electrons, or an octet. Lewis symbols and Lewis structure A Lewis symbol consists of a chemical symbol to represent the nucleus and core (inner-shell) electrons of an atom, together with dots placed around the symbol to represent the valence (outer-shell) electrons. the Lewis symbol for silicon, which has the electron configuration [Ne]3s23p6, is We will write Lewis symbols in the way Lewis did. We will place single dots on the sides of the symbol, up to a maximum of four. Then we will pair up dots until we reach an octet. Lewis symbols are commonly written for main-group elements but much less often for transition elements. A Lewis structure is a combination of Lewis symbols that represents either the transfer or the sharing of electrons in a chemical bond IONic Bonding electrons are transferred between valence shells of atoms ionic compounds are made of ions NOT MOLECULES ionic compounds are called Salts or Crystals IONic Bonding Electronegativity difference > 2.0 Look up e-neg of the atoms in the bond and subtract NaCl CaCl2 Compounds with polyatomic ions NaNO3 Properties of Ionic Compounds SALTS hard solid @ 22oC Crystals high mp temperatures nonconductors of electricity in solid phase good conductors in liquid phase or dissolved in water (aq) Covalent Bonding molecules Pairs of e- are shared between non-metal atoms electronegativity difference < 2.0 forms polyatomic ions Covalent Bonding The broken circles represent the outermost electron shells of the bonded atoms. The number of dots lying on or within each circle represents the effective number of electrons in each valence shell. The H atom has two dots, as in the electron configuration of He. The Cl atom has eight dots, corresponding to the outershell configuration of Ar. Note that we counted the two electrons between H and Cl twice. These two electrons are shared by the H and Cl atoms. This shared pair of electrons constitutes the covalent bond. As was the case for Cl in HCl, the O atom in the Lewis structure of H2O and Cl2O in is surrounded by eight electrons (when the bond-pair electrons are double counted). In attaining these eight electrons, the O atom conforms to the octet rule a requirement of eight valence- shell electrons for the atoms in a Lewis structure. Note, however, that the H atom is an exception to this rule. The H atom can accommodate only two valence-shell electrons. The sharing of a single pair of electrons between bonded atoms produces a single covalent bond. To underscore the importance of electron pairs in the Lewis theory the term bond pair applies to a pair of electrons in a covalent bond, while lone pair applies to electron pairs that are not involved in bonding. Also, in writing Lewis structures it is customary to replace bond pairs with lines ( ). These features are shown in the following Lewis structures. The bond formed between the N atom of and the ion in structure is a coordinate covalent bond. It is important to note, however, that once the bond has formed, it is impossible to say which of the four bonds is the coordinate covalent bond. Thus, a coordinate covalent bond is indistinguishable from a regular covalent bond. Multiple Covalent Bonds More than one pair of electrons must be shared if an atom is to attain an octet (noble gas electron configuration). CO2 and N2 are two molecules in which atoms share more than one pair of electrons. C atom can share a valence electron with each O atom, thus forming two carbon-to-oxygen single bonds. Lewis structure for the molecule. Our first attempt might again involve a single covalent bond and the incorrect structure shown below: Each N atom appears to have only six outer-shell electrons, not the expected eight. The situation can be corrected by bringing the four unpaired electrons into the region between the N atoms and using them for additional bond pairs. In all, we now show the sharing of three pairs of electrons between the N atoms. Polar Covalent Bonds A covalent bond in which electrons are not shared equally between two atoms is called a polar covalent bond. The unequal sharing of the electrons leads to a partial negative charge on the more nonmetallic element, signified by and a corresponding partial positive charge on the more metallic element, designated by Electronegativity Electronegativity (EN) describes an atom s ability to compete for electrons with other atoms to which it is bonded. As such, electronegativity is related to ionization energy (I) and electron affinity (EA). Writing Lewis Structures All the valence electrons of the atoms in a Lewis structure must appear in the structure. Usually, all the electrons in a Lewis structure are paired. Usually, each atom acquires an outer-shell octet of electrons. Hydrogen, however, is limited to two outer-shell electrons. Sometimes, multiple covalent bonds (double or triple bonds) are needed. Multiple covalent bonds are formed most readily by C, N, O, P, and S atoms. Skeletal structure all the atoms in the structure arranged in the order in which they are bonded to one another. In a skeletal structure with more than two atoms, we generally need to distinguish between central and terminal atoms. A central atom is bonded to two or more atoms, and a terminal atom is bonded to just one other atom. Hydrogen atoms are always terminal atoms. This is because an H atom can accommodate only two electrons in its valence shell, so it can form only one bond to another atom. Central atoms are generally those with the lowest electronegativity. Carbon atoms are always central atoms. Molecules and polyatomic ions generally have compact, symmetrical structures Resonance When we apply the usual rules for Lewis structures for ozone, we come up with these two possibilities. The situation in which two or more plausible Lewis structures contribute to the correct structure is called resonance. Chemical Bondings Reference: General Chemistry Principles and Modern Applications TENTH EDITION, Pearson Canada Toronto Contents Lewis Theory: An Overview Covalent Bonding: An Introduction Polar Covalent Bonds and Electrostatic Potential Maps Writing Lewis Structures Some fundamental ideas associated with Lewis s theory follow: 1. Electrons, especially those of the outermost (valence) electronic shell, playa fundamental role in chemical bonding. 2. In some cases, electrons are transferred from one atom to another. Positive and negative ions are formed and attract each other through electrostatic forces called ionic bonds. 3. In other cases, one or more pairs of electrons are shared between atoms. A bond formed by the sharing of electrons between atoms is called a covalent bond. 4. Electrons are transferred or shared in such a way that each atom acquires an especially stable electron configuration. Usually this is a noble gas configuration, one with eight outer-shell electrons, or an octet. Lewis symbols and Lewis structure A Lewis symbol consists of a chemical symbol to represent the nucleus and core (inner-shell) electrons of an atom, together with dots placed around the symbol to represent the valence (outer-shell) electrons. the Lewis symbol for silicon, which has the electron configuration [Ne]3s23p6, is We will write Lewis symbols in the way Lewis did. We will place single dots on the sides of the symbol, up to a maximum of four. Then we will pair up dots until we reach an octet. Lewis symbols are commonly written for main-group elements but much less often for transition elements. A Lewis structure is a combination of Lewis symbols that represents either the transfer or the sharing of electrons in a chemical bond IONic Bonding electrons are transferred between valence shells of atoms ionic compounds are made of ions NOT MOLECULES ionic compounds are called Salts or Crystals IONic Bonding Electronegativity difference > 2.0 Look up e-neg of the atoms in the bond and subtract NaCl CaCl2 Compounds with polyatomic ions NaNO3 Properties of Ionic Compounds SALTS hard solid @ 22oC Crystals high mp temperatures nonconductors of electricity in solid phase good conductors in liquid phase or dissolved in water (aq) Covalent Bonding molecules Pairs of e- are shared between non-metal atoms electronegativity difference < 2.0 forms polyatomic ions Covalent Bonding The broken circles represent the outermost electron shells of the bonded atoms. The number of dots lying on or within each circle represents the effective number of electrons in each valence shell. The H atom has two dots, as in the electron configuration of He. The Cl atom has eight dots, corresponding to the outershell configuration of Ar. Note that we counted the two electrons between H and Cl twice. These two electrons are shared by the H and Cl atoms. This shared pair of electrons constitutes the covalent bond. As was the case for Cl in HCl, the O atom in the Lewis structure of H2O and Cl2O in is surrounded by eight electrons (when the bond-pair electrons are double counted). In attaining these eight electrons, the O atom conforms to the octet rule a requirement of eight valence- shell electrons for the atoms in a Lewis structure. Note, however, that the H atom is an exception to this rule. The H atom can accommodate only two valence-shell electrons. The sharing of a single pair of electrons between bonded atoms produces a single covalent bond. To underscore the importance of electron pairs in the Lewis theory the term bond pair applies to a pair of electrons in a covalent bond, while lone pair applies to electron pairs that are not involved in bonding. Also, in writing Lewis structures it is customary to replace bond pairs with lines ( ). These features are shown in the following Lewis structures. The bond formed between the N atom of and the ion in structure is a coordinate covalent bond. It is important to note, however, that once the bond has formed, it is impossible to say which of the four bonds is the coordinate covalent bond. Thus, a coordinate covalent bond is indistinguishable from a regular covalent bond. Multiple Covalent Bonds More than one pair of electrons must be shared if an atom is to attain an octet (noble gas electron configuration). CO2 and N2 are two molecules in which atoms share more than one pair of electrons. C atom can share a valence electron with each O atom, thus forming two carbon-to-oxygen single bonds. Lewis structure for the molecule. Our first attempt might again involve a single covalent bond and the incorrect structure shown below: Each N atom appears to have only six outer-shell electrons, not the expected eight. The situation can be corrected by bringing the four unpaired electrons into the region between the N atoms and using them for additional bond pairs. In all, we now show the sharing of three pairs of electrons between the N atoms. Polar Covalent Bonds A covalent bond in which electrons are not shared equally between two atoms is called a polar covalent bond. The unequal sharing of the electrons leads to a partial negative charge on the more nonmetallic element, signified by and a corresponding partial positive charge on the more metallic element, designated by Electronegativity Electronegativity (EN) describes an atom s ability to compete for electrons with other atoms to which it is bonded. As such, electronegativity is related to ionization energy (I) and electron affinity (EA). Writing Lewis Structures All the valence electrons of the atoms in a Lewis structure must appear in the structure. Usually, all the electrons in a Lewis structure are paired. Usually, each atom acquires an outer-shell octet of electrons. Hydrogen, however, is limited to two outer-shell electrons. Sometimes, multiple covalent bonds (double or triple bonds) are needed. Multiple covalent bonds are formed most readily by C, N, O, P, and S atoms. Skeletal structure all the atoms in the structure arranged in the order in which they are bonded to one another. In a skeletal structure with more than two atoms, we generally need to distinguish between central and terminal atoms. A central atom is bonded to two or more atoms, and a terminal atom is bonded to just one other atom. Hydrogen atoms are always terminal atoms. This is because an H atom can accommodate only two electrons in its valence shell, so it can form only one bond to another atom. Central atoms are generally those with the lowest electronegativity. Carbon atoms are always central atoms. Molecules and polyatomic ions generally have compact, symmetrical structures Resonance When we apply the usual rules for Lewis structures for ozone, we come up with these two possibilities. The situation in which two or more plausible Lewis structures contribute to the correct structure is called resonance. Acids and Bases References : 1. General Chemistry- principles and modern applications (Petrucci, Herring, Madura, Bissonnette) 2. Chemistry-10th Edition (Raymond Chang ) Acids, Bases, and Conjugate Acid–Base Pairs One of the most useful theories of acids and bases, particularly for describing the reactions of acids and bases in aqueous solutions, is the Brønsted–Lowry theory. In 1923, J. N. Brønsted and T. M. Lowry in Great Britain independently proposed that an acid is a proton donor and a base is a proton acceptor. Let’s use the Brønsted– Lowry theory to describe the ionization of CH3COOH in aqueous solution. In reaction, CH3COOH acts as an acid. It gives up a proton, H+ , which is taken up by H2O. Thus, H2O acts as a base. In the reverse reaction, the hydronium ion, H3O+ acts as an acid and CH3COO- acts as a base. When CH3COOH loses a proton, it is converted into CH3COO-. Notice that the formulas of these two species differ by a single proton, H+. Species that differ by a single proton (H+ ) constitute a conjugate acid–base pair. Within this pair, the species with the added H+ is the acid, and the species without the H+ is the base. Thus, for reaction (16.1), we can identify two conjugate acid–base pairs. It will be helpful to summarize some key aspects of the Brønsted–Lowry theory. 1. An acid contains at least one ionizable H atom, and a base contains an atom with a lone pair of electrons onto which a proton can bind. For this reason, an acid may be represented in the Brønsted–Lowry theory by the general formula HA, H2A, H3A, etc., depending on the number of ionizable H atoms, and a base is represented by :B. There are substances that contain both an ionizable H atom and an atom with a lone pair of electrons. Such substances may behave as either an acid or a base, depending on the situation, and are said to be amphiprotic. 2. For a conjugate acid–base pair, the molecular formulas for the acid and base differ by a single proton (H+). Therefore, to identify the species in a solution that constitute a conjugate acid–base pair, we need only identify those species that have molecular formulas that differ by one H+ ion. Once such a pair has been identified, the species with the added H is the acid, and the species without the H+ is the base. For example, H2O and OH- are a conjugate acid–base pair because their formulas differ by one H. In this pair, H2O is the acid and OH is the base. Similarly, because the formulas of NH+4 and NH3 differ by one H+ , these two species constitute a conjugate acid– base pair, with NH+4 as the acid and NH3 as the base. 3. When added to water, acids protonate water molecules to form hydronium (H3O+ ) ions and bases deprotonate water molecules to form hydroxide (OH- ) ions. The ability of the Brønsted–Lowry theory to account for the presence these ions in solution arises from its recognition of the role played by the solvent and makes it a more general and useful theory than the Arrhenius theory. Self-Ionization of Water and the pH Scale H2O molecule can act as either an acid or a base it is amphiprotic. It should come as no surprise that amongst themselves water molecules can produce H3O+ and OH- ions via the following self-ionization reaction or autoionization reaction: The Ion Product of Water In the study of acid-base reactions, the hydrogen ion concentration is key; its value indicates the acidity or basicity of the solution. Because only a very small fraction of water molecules are ionized, the concentration of water remains virtually unchanged. Therefore, the equilibrium constant for the autoionization of water, is The self-ionization of water is an important reaction, from a conceptual point of view, because it reveals an important relationship between [H3O ] and [OH- ] that applies to all aqueous solutions. From a practical standpoint, the reaction is not of much concern to us except when dealing with extremely dilute solutions. In fact, the self-ionization of water is partially suppressed by the addition of acid or base to water. This statement is easily justified by applying Le Châtelier’s principle to reaction (16.3). When an acid is added to water, H2O molecules are protonated and [H3O+ ] increases. The increase in [H3O+ ] causes net change to the left in reaction (16.3), and, thus, the self-ionization of water is partially suppressed. Similarly, the addition of a base to water increases [OH- ], causes net change to the left, and partially suppresses the self-ionization of water. pH and pOH Because the concentrations of H and OH ions in aqueous solutions are frequently very small numbers and therefore inconvenient to work with, Soren Sorensen† in 1909 proposed a more practical measure called pH. The pH of a solution is defined as the negative logarithm of the hydrogen ion concentration (in mol/L). Acidic, Basic, and Neutral Solutions In pure water, the concentrations of H3O+ and OH- are equal. However, when an acid or a base is added to water, the H3O+ and OH- ions are no longer present in equal amounts. By comparing the values of [H3O+ ] and [OH-], we can classify a solution as acidic, basic, or neutral (Table 16.1). The classification can also be made, at , by focusing on either [H3O+] or the pH. The relationships between [H3O ], [OH ], pH, and pOH, for acidic, basic, and neutral solutions, are illustrated in Figure 16-4. The pH values of a number of materials—some acidic and others basic—are depicted in Figure 16-5. Ionization of Acids and Bases in Water Figure 16-6 illustrates two ways of showing that ionization has occurred in a solution of acid. One is by the color of an acid–base indicator; the other, the response of a pH meter. The pink color of the solution in Figure 16-7 tells us the pH of the HCl solution is less than 1.2. The pH meter registers a value of 1.20, indicating that [H3O ]=10 -1.20 M=0.063 M in the HCl solution. The yellow color of the solution in Figure 16-7 indicates that the pH of 0.1 M CH3COOH (acetic acid) is 2.8 or greater. The pH meter registers 2.80, and thus [H3O ]=10 -2.80 M=0.0016 M. Notice that the ionization of HCl generates a higher [H3O+] than does the ionization of CH3COOH, even though the initial molarity of CH3COOH (0.1 M) is greater than that of HCl (0.06 to 0.07 M). From this, we conclude that the ionization of HCl occurs to a greater extent than does the ionization of CH3COOH, an indication that HCl is a much stronger acid than CH3COOH. This conclusion is reflected in the placements of HCl and CH3COOH in Table 16.2 which ranks a number of acids and bases in order of increasing acid or base strengths. This ordering is established by experiment. The strength of an acid or a base is quantified by the value of the equilibrium constant for the reaction describing its ionization in water. As discussed earlier, a monoprotic Brønsted–Lowry acid may be represented by the general formula HA. Therefore, the ionization of an acid may be represented generally by the following equation. The value of Ka or Kb gives an indication of the strength of an acid or a base. The following points are worth remembering 1. A strong acid or base has a large ionization constant: Ka or Kb is much greater than 1. Therefore, we expect that the corresponding ionization reaction goes almost to completion. In most situations, we can safely assume that a strong acid or strong base is completely ionized in solution. Fortunately, there are relatively few common strong acids and strong bases Notice that the listing in Table 16.3 does not include Ka or Kb values; these values are not needed. The main point is that the ionization constants are large enough to ensure that the acids and bases in Table 16.3 are almost completely ionized in aqueous solution. Memorizing the list in Table 16.3 can be extremely helpful. For example, if the situation we are dealing with involves a strong acid or base, we can safely assume the strong acid or base will react to completion. On the other hand, if the situation we are considering involves an acid and a base that are not listed in Table 16.3, we can safely assume that the acid and base are weak and react to a limited extent only. The strong acids listed in Table 16.3 are molecular compounds whereas the strong bases are soluble ionic compounds called hydroxides. Molecular compounds ionize in water: Neutral HA molecules produce H3O+ and A- ions by reacting with water (equation 16.9). On the other hand, soluble ionic hydroxides dissociate in water: Positive and negative ions (for example, Na and OH ), which are already present in the solid structure, enter the solution as free ions. 2. A weak acid or base has a small ionization constant: Ka or Kb is much less than 1. For a weak acid or base, the corresponding ionization reaction occurs to a limited extent, with a significant fraction of the acid or base not ionized. To determine the equilibrium composition of a solution of a weak acid or weak base, we need to solve an equilibrium problem, typically by using an ICE table and the value of the appropriate ionization constant, Ka or K b. Ionization constants of some weak acids and weak bases are provided in Table 16.4. Strong Acids and Strong Bases Polyprotic Acids All the acids listed in Table 16.4 are weak monoprotic acids, meaning that their molecules have only one ionizable H atom, even though several of these acids contain more than one H atom. But some acids have more than one ionizable H atom per molecule. These are polyprotic acids. The Relationship Between the Ionization Constants of Acids and Their Conjugate Bases Acids and Bases References : 1. General Chemistry- principles and modern applications (Petrucci, Herring, Madura, Bissonnette) 2. Chemistry-10th Edition (Raymond Chang ) Acids, Bases, and Conjugate Acid–Base Pairs One of the most useful theories of acids and bases, particularly for describing the reactions of acids and bases in aqueous solutions, is the Brønsted–Lowry theory. In 1923, J. N. Brønsted and T. M. Lowry in Great Britain independently proposed that an acid is a proton donor and a base is a proton acceptor. Let’s use the Brønsted– Lowry theory to describe the ionization of CH3COOH in aqueous solution. In reaction, CH3COOH acts as an acid. It gives up a proton, H+ , which is taken up by H2O. Thus, H2O acts as a base. In the reverse reaction, the hydronium ion, H3O+ acts as an acid and CH3COO- acts as a base. When CH3COOH loses a proton, it is converted into CH3COO-. Notice that the formulas of these two species differ by a single proton, H+. Species that differ by a single proton (H+ ) constitute a conjugate acid–base pair. Within this pair, the species with the added H+ is the acid, and the species without the H+ is the base. Thus, for reaction (16.1), we can identify two conjugate acid–base pairs. It will be helpful to summarize some key aspects of the Brønsted–Lowry theory. 1. An acid contains at least one ionizable H atom, and a base contains an atom with a lone pair of electrons onto which a proton can bind. For this reason, an acid may be represented in the Brønsted–Lowry theory by the general formula HA, H2A, H3A, etc., depending on the number of ionizable H atoms, and a base is represented by :B. There are substances that contain both an ionizable H atom and an atom with a lone pair of electrons. Such substances may behave as either an acid or a base, depending on the situation, and are said to be amphiprotic. 2. For a conjugate acid–base pair, the molecular formulas for the acid and base differ by a single proton (H+). Therefore, to identify the species in a solution that constitute a conjugate acid–base pair, we need only identify those species that have molecular formulas that differ by one H+ ion. Once such a pair has been identified, the species with the added H is the acid, and the species without the H+ is the base. For example, H2O and OH- are a conjugate acid–base pair because their formulas differ by one H. In this pair, H2O is the acid and OH is the base. Similarly, because the formulas of NH+4 and NH3 differ by one H+ , these two species constitute a conjugate acid– base pair, with NH+4 as the acid and NH3 as the base. 3. When added to water, acids protonate water molecules to form hydronium (H3O+ ) ions and bases deprotonate water molecules to form hydroxide (OH- ) ions. The ability of the Brønsted–Lowry theory to account for the presence these ions in solution arises from its recognition of the role played by the solvent and makes it a more general and useful theory than the Arrhenius theory. Self-Ionization of Water and the pH Scale H2O molecule can act as either an acid or a base it is amphiprotic. It should come as no surprise that amongst themselves water molecules can produce H3O+ and OH- ions via the following self-ionization reaction or autoionization reaction: The Ion Product of Water In the study of acid-base reactions, the hydrogen ion concentration is key; its value indicates the acidity or basicity of the solution. Because only a very small fraction of water molecules are ionized, the concentration of water remains virtually unchanged. Therefore, the equilibrium constant for the autoionization of water, is The self-ionization of water is an important reaction, from a conceptual point of view, because it reveals an important relationship between [H3O ] and [OH- ] that applies to all aqueous solutions. From a practical standpoint, the reaction is not of much concern to us except when dealing with extremely dilute solutions. In fact, the self-ionization of water is partially suppressed by the addition of acid or base to water. This statement is easily justified by applying Le Châtelier’s principle to reaction (16.3). When an acid is added to water, H2O molecules are protonated and [H3O+ ] increases. The increase in [H3O+ ] causes net change to the left in reaction (16.3), and, thus, the self-ionization of water is partially suppressed. Similarly, the addition of a base to water increases [OH- ], causes net change to the left, and partially suppresses the self-ionization of water. pH and pOH Because the concentrations of H and OH ions in aqueous solutions are frequently very small numbers and therefore inconvenient to work with, Soren Sorensen† in 1909 proposed a more practical measure called pH. The pH of a solution is defined as the negative logarithm of the hydrogen ion concentration (in mol/L). Acidic, Basic, and Neutral Solutions In pure water, the concentrations of H3O+ and OH- are equal. However, when an acid or a base is added to water, the H3O+ and OH- ions are no longer present in equal amounts. By comparing the values of [H3O+ ] and [OH-], we can classify a solution as acidic, basic, or neutral (Table 16.1). The classification can also be made, at , by focusing on either [H3O+] or the pH. The relationships between [H3O ], [OH ], pH, and pOH, for acidic, basic, and neutral solutions, are illustrated in Figure 16-4. The pH values of a number of materials—some acidic and others basic—are depicted in Figure 16-5. Ionization of Acids and Bases in Water Figure 16-6 illustrates two ways of showing that ionization has occurred in a solution of acid. One is by the color of an acid–base indicator; the other, the response of a pH meter. The pink color of the solution in Figure 16-7 tells us the pH of the HCl solution is less than 1.2. The pH meter registers a value of 1.20, indicating that [H3O ]=10 -1.20 M=0.063 M in the HCl solution. The yellow color of the solution in Figure 16-7 indicates that the pH of 0.1 M CH3COOH (acetic acid) is 2.8 or greater. The pH meter registers 2.80, and thus [H3O ]=10 -2.80 M=0.0016 M. Notice that the ionization of HCl generates a higher [H3O+] than does the ionization of CH3COOH, even though the initial molarity of CH3COOH (0.1 M) is greater than that of HCl (0.06 to 0.07 M). From this, we conclude that the ionization of HCl occurs to a greater extent than does the ionization of CH3COOH, an indication that HCl is a much stronger acid than CH3COOH. This conclusion is reflected in the placements of HCl and CH3COOH in Table 16.2 which ranks a number of acids and bases in order of increasing acid or base strengths. This ordering is established by experiment. The strength of an acid or a base is quantified by the value of the equilibrium constant for the reaction describing its ionization in water. As discussed earlier, a monoprotic Brønsted–Lowry acid may be represented by the general formula HA. Therefore, the ionization of an acid may be represented generally by the following equation. The value of Ka or Kb gives an indication of the strength of an acid or a base. The following points are worth remembering 1. A strong acid or base has a large ionization constant: Ka or Kb is much greater than 1. Therefore, we expect that the corresponding ionization reaction goes almost to completion. In most situations, we can safely assume that a strong acid or strong base is completely ionized in solution. Fortunately, there are relatively few common strong acids and strong bases Notice that the listing in Table 16.3 does not include Ka or Kb values; these values are not needed. The main point is that the ionization constants are large enough to ensure that the acids and bases in Table 16.3 are almost completely ionized in aqueous solution. Memorizing the list in Table 16.3 can be extremely helpful. For example, if the situation we are dealing with involves a strong acid or base, we can safely assume the strong acid or base will react to completion. On the other hand, if the situation we are considering involves an acid and a base that are not listed in Table 16.3, we can safely assume that the acid and base are weak and react to a limited extent only. The strong acids listed in Table 16.3 are molecular compounds whereas the strong bases are soluble ionic compounds called hydroxides. Molecular compounds ionize in water: Neutral HA molecules produce H3O+ and A- ions by reacting with water (equation 16.9). On the other hand, soluble ionic hydroxides dissociate in water: Positive and negative ions (for example, Na and OH ), which are already present in the solid structure, enter the solution as free ions. 2. A weak acid or base has a small ionization constant: Ka or Kb is much less than 1. For a weak acid or base, the corresponding ionization reaction occurs to a limited extent, with a significant fraction of the acid or base not ionized. To determine the equilibrium composition of a solution of a weak acid or weak base, we need to solve an equilibrium problem, typically by using an ICE table and the value of the appropriate ionization constant, Ka or K b. Ionization constants of some weak acids and weak bases are provided in Table 16.4. Strong Acids and Strong Bases Polyprotic Acids All the acids listed in Table 16.4 are weak monoprotic acids, meaning that their molecules have only one ionizable H atom, even though several of these acids contain more than one H atom. But some acids have more than one ionizable H atom per molecule. These are polyprotic acids. The Relationship Between the Ionization Constants of Acids and Their Conjugate Bases Radioactivity Reference: General Chemistry Principles and Modern Applications TENTH EDITION, Pearson Canada Toronto Content Radioactivity Naturally Occurring Radioactive Isotopes Nuclear Reactions and Artificially Induced Radioactivity Rate of Radioactive Decay Nuclear Stability Nuclear Fission Nuclear Fusion Background Traditional Chemistry Reactions occur due to interactions between valence electrons (surrounding nucleus) Late 1800s – Early 1900s New Developments Discovery that Uranium emits radiation (Henry Becquerel) Amount of radiation emitted is proportional to amount of element present (Marie Curie) “Radioactive” substances = radiation-emitting (Curie) Radioactivity = a inherent property of certain ATOMS, as opposed to a chemical property of compounds (Curie) Birth of nuclear chemistry The Discovery of Radioactivity (1895 – 1898): Rountgen found that invisible rays were emitted when electrons hit the surface of a fluorosent screen (discovered x-rays) Becquerel accidently discovered that phosphorescent uranium rock produced spots on photographic plates The Discovery of Radioactivity (1895 – 1898): Marie and Pierre Curie isolated the components (uranium atoms) emitting the rays. Radioactivity – process by which atoms give off rays or particles. Radiation – the penetrating rays and particles emitted by a radioactive source. The Discovery of Radioactivity (1895 – 1898): Marie Curie, continued identified 2 new elements, Polonium and Radium on the basis of their radioactivity. These findings contradicted Dalton’s theory of indivisible atoms. Chemical vs. Nuclear Reactions Chemical Reactions Nuclear Reactions Bonds are broken Nuclei emit particles and/or rays Atoms are rearranged Atoms change into atoms of different element Involve valence electrons Involve protons, neutrons, and/or electrons Small energy changes Large energy changes Reaction rate can be changed. Reaction rate cannot be changed Isotopes – atoms of the same element with different numbers of neutrons. Radioisotopes – isotopes of atoms with unstable nuclei (too many or too few neutrons). Radioactive decay – when unstable nuclei lose energy by emitting radiation to become more stable Nuclear Stability How can a stable nucleus exist? – Net positive charge in nucleus surrounded by negative charges (electrons) – Electrostatic force Opposite charges attract Like charges repel – Why doesn’t the nucleus break apart? Nuclear Force (1934) – Force between protons and neutrons than binds nucleus together within atom – Very strong (must be!) Which Elements/Isotopes Are Radioactive? Based on the nuclear force and nuclear stability More protons in nucleus more neutrons needed to bind nucleus together Critical Factor = Neutral-to-Proton Ratio Neutron-to-Proton ratios of stable nuclei increase with increasing atomic number Unstable neutron-to-proton ratio = RADIOACTIVE Nuclei with atomic numbers ≥ 84 = ALWAYS Radioactive Very large nuclei! Neutron-to-proton ratio always unstable Spontaneous Nuclear Reactions Radiation and Radioactive Decay Unstable (radioactive) nuclei emit radiation (energy) in order to become more stable Radioactive Decay – occurs when a nucleus spontaneously decomposes in this way 3 Common Types of Nuclear Reactions Alpha Radiation Beta Radiation Gamma Radiation Alpha radiation Composition – Alpha particles, same as helium nuclei 4 Symbol – Helium nuclei, 2He, α Charge – 2+ Mass (amu) – 4 Approximate energy – 5 MeV Penetrating power – low (0.05 mm body tissue) Shielding – paper, clothing Alpha Radiation: Nuclear Equation Emission of 2 protons and 2 neutrons (as an alpha particle, 42 He ) from radioactive atom’s nucleus Atom’s atomic mass decreases by 4 units Atom’s atomic number decreases by 2 units (a different element!) Beta radiation Composition – Beta particles, same as an electron Symbol – e-, 0-1β Charge – 1- Mass (amu) – 1/1837 (practically 0) Approximate energy – 0.05 – 1 MeV Penetrating power – moderate (4 mm body tissue) Shielding – metal foil Beta negative Radiation: Nuclear Equation Conversion of a neutron into a proton and an electron, followed by the emission of the electron (beta particle) from the nucleus Atom’s atomic mass does NOT change Atom’s atomic number increases by 1 units (a different element!) Gamma radiation Composition – High-energy electromagnetic radiation Symbol – ooγ Charge – 0 Mass (amu) – 0 Approximate energy – 1 MeV Penetrating power – high (penetrates body easily) Shielding – lead, concrete Review Type of Particle Change in Change in Radioactive Emitted Mass # Atomic # Decay 4 Alpha α 2He -4 -2 Beta β -10 e 0 +1 Gamma γ 0 0 Relative Penetrating Power of Radiation Types Alpha radiation has low energy and little penetrating power compared to beta radiation Gamma has the most energy and penetrating power Half-Life Half time is the time required for half of a radioisotope’s nuclei to decay into its products. For any radioisotope, # of ½ lives % Remaining 0 100% 1 50% 2 25% 3 12.5% 4 6.25% 5 3.125% 6 1.5625% Different Elements Different Half-Lives Data for most stable isotope of element Half-Life initial mass mt = m0 x (0.5)n mass remaining of half-lives Stimulated Nuclear Reactions Nuclear Fission Fission-splitting of a nucleus - Very heavy nucleus is split into two approximately equal fragments. - Chain reaction releases several neutrons which split more nuclei - If controlled, energy is released slowly (like in nuclear reactors) Reaction control depends on reducing the speed of the neutrons (increases the reaction rate) and absorbing extra neutrons (decreases the reaction rate). Nuclear Fusion - Fusion: Combining of two nuclei - Two light nuclei combine to form a single heavier nucleus - Does not occur under standard conditions (positive nuclei repel each other) - Advantages compared to fission – No radioactive waste, inexpensive - Disadvantages - requires large amount of energy to start, difficult to control - Examples – energy output of stars, hydrogen bomb, future nuclear power plants Radioactivity Reference: General Chemistry Principles and Modern Applications TENTH EDITION, Pearson Canada Toronto Content Radioactivity Naturally Occurring Radioactive Isotopes Nuclear Reactions and Artificially Induced Radioactivity Rate of Radioactive Decay Nuclear Stability Nuclear Fission Nuclear Fusion Background Traditional Chemistry Reactions occur due to interactions between valence electrons (surrounding nucleus) Late 1800s – Early 1900s New Developments Discovery that Uranium emits radiation (Henry Becquerel) Amount of radiation emitted is proportional to amount of element present (Marie Curie) “Radioactive” substances = radiation-emitting (Curie) Radioactivity = a inherent property of certain ATOMS, as opposed to a chemical property of compounds (Curie) Birth of nuclear chemistry The Discovery of Radioactivity (1895 – 1898): Rountgen found that invisible rays were emitted when electrons hit the surface of a fluorosent screen (discovered x-rays) Becquerel accidently discovered that phosphorescent uranium rock produced spots on photographic plates The Discovery of Radioactivity (1895 – 1898): Marie and Pierre Curie isolated the components (uranium atoms) emitting the rays. Radioactivity – process by which atoms give off rays or particles. Radiation – the penetrating rays and particles emitted by a radioactive source. The Discovery of Radioactivity (1895 – 1898): Marie Curie, continued identified 2 new elements, Polonium and Radium on the basis of their radioactivity. These findings contradicted Dalton’s theory of indivisible atoms. Chemical vs. Nuclear Reactions Chemical Reactions Nuclear Reactions Bonds are broken Nuclei emit particles and/or rays Atoms are rearranged Atoms change into atoms of different element Involve valence electrons Involve protons, neutrons, and/or electrons Small energy changes Large energy changes Reaction rate can be changed. Reaction rate cannot be changed Isotopes – atoms of the same element with different numbers of neutrons. Radioisotopes – isotopes of atoms with unstable nuclei (too many or too few neutrons). Radioactive decay – when unstable nuclei lose energy by emitting radiation to become more stable Nuclear Stability How can a stable nucleus exist? – Net positive charge in nucleus surrounded by negative charges (electrons) – Electrostatic force Opposite charges attract Like charges repel – Why doesn’t the nucleus break apart? Nuclear Force (1934) – Force between protons and neutrons than binds nucleus together within atom – Very strong (must be!) Which Elements/Isotopes Are Radioactive? Based on the nuclear force and nuclear stability More protons in nucleus more neutrons needed to bind nucleus together Critical Factor = Neutral-to-Proton Ratio Neutron-to-Proton ratios of stable nuclei increase with increasing atomic number Unstable neutron-to-proton ratio = RADIOACTIVE Nuclei with atomic numbers ≥ 84 = ALWAYS Radioactive Very large nuclei! Neutron-to-proton ratio always unstable Spontaneous Nuclear Reactions Radiation and Radioactive Decay Unstable (radioactive) nuclei emit radiation (energy) in order to become more stable Radioactive Decay – occurs when a nucleus spontaneously decomposes in this way 3 Common Types of Nuclear Reactions Alpha Radiation Beta Radiation Gamma Radiation Alpha radiation Composition – Alpha particles, same as helium nuclei 4 Symbol – Helium nuclei, 2He, α Charge – 2+ Mass (amu) – 4 Approximate energy – 5 MeV Penetrating power – low (0.05 mm body tissue) Shielding – paper, clothing Alpha Radiation: Nuclear Equation Emission of 2 protons and 2 neutrons (as an alpha particle, 42 He ) from radioactive atom’s nucleus Atom’s atomic mass decreases by 4 units Atom’s atomic number decreases by 2 units (a different element!) Beta radiation Composition – Beta particles, same as an electron Symbol – e-, 0-1β Charge – 1- Mass (amu) – 1/1837 (practically 0) Approximate energy – 0.05 – 1 MeV Penetrating power – moderate (4 mm body tissue) Shielding – metal foil Beta negative Radiation: Nuclear Equation Conversion of a neutron into a proton and an electron, followed by the emission of the electron (beta particle) from the nucleus Atom’s atomic mass does NOT change Atom’s atomic number increases by 1 units (a different element!) Gamma radiation Composition – High-energy electromagnetic radiation Symbol – ooγ Charge – 0 Mass (amu) – 0 Approximate energy – 1 MeV Penetrating power – high (penetrates body easily) Shielding – lead, concrete Review Type of Particle Change in Change in Radioactive Emitted Mass # Atomic # Decay 4 Alpha α 2He -4 -2 Beta β -10 e 0 +1 Gamma γ 0 0 Relative Penetrating Power of Radiation Types Alpha radiation has low energy and little penetrating power compared to beta radiation Gamma has the most energy and penetrating power Half-Life Half time is the time required for half of a radioisotope’s nuclei to decay into its products. For any radioisotope, # of ½ lives % Remaining 0 100% 1 50% 2 25% 3 12.5% 4 6.25% 5 3.125% 6 1.5625% Different Elements Different Half-Lives Data for most stable isotope of element Half-Life initial mass mt = m0 x (0.5)n mass remaining of half-lives Stimulated Nuclear Reactions Nuclear Fission Fission-splitting of a nucleus - Very heavy nucleus is split into two approximately equal fragments. - Chain reaction releases several neutrons which split more nuclei - If controlled, energy is released slowly (like in nuclear reactors) Reaction control depends on reducing the speed of the neutrons (increases the reaction rate) and absorbing extra neutrons (decreases the reaction rate). Nuclear Fusion - Fusion: Combining of two nuclei - Two light nuclei combine to form a single heavier nucleus - Does not occur under standard conditions (positive nuclei repel each other) - Advantages compared to fission – No radioactive waste, inexpensive - Disadvantages - requires large amount of energy to start, difficult to control - Examples – energy output of stars, hydrogen bomb, future nuclear power plants