Ischemic Heart Disease PDF
Document Details
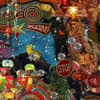
Uploaded by Nany
Collegium Medicum Uniwersytetu Mikołaja Kopernika
Tags
Summary
This document provides a comprehensive introduction to ischemic heart disease (IHD), covering its causes, symptoms, epidemiology, and pathogenesis. It discusses factors like reduced coronary blood flow, atherosclerosis, angina pectoris, and myocardial infarction (MI).
Full Transcript
# Ischemic Heart Disease Ischemic heart disease (IHD) is a broad term encompassing several closely related syndromes caused by an imbalance between cardiac blood supply (perfusion) and myocardial oxygen and nutritional demands. Despite dramatic improvements in therapy in the past quarter century, I...
# Ischemic Heart Disease Ischemic heart disease (IHD) is a broad term encompassing several closely related syndromes caused by an imbalance between cardiac blood supply (perfusion) and myocardial oxygen and nutritional demands. Despite dramatic improvements in therapy in the past quarter century, IHD in its various forms remains the leading cause of mortality in the United States and other higher income nations, accounting for 7.5 million deaths worldwide each year. In more than 90% of cases, IHD is a consequence of reduced coronary blood flow secondary to obstructive atherosclerotic vascular disease (Chapter 8). Thus, unless otherwise specified, IHD is usually synonymous with coronary artery disease (CAD). In most cases, the various syndromes of IHD are consequences of coronary atherosclerosis that has been gradually progressing for decades. In the remaining cases, cardiac ischemia may be the result of increased demand (e.g., with increased heart rate or hypertension); diminished blood volume (e.g., with hypotension or shock); diminished blood oxygenation (e.g., due to pneumonia or CHF); or diminished blood oxygen-carrying capacity (e.g., due to anemia or carbon monoxide poisoning). Cardiac myocytes generate energy almost exclusively through mitochondrial oxidative phosphorylation, and their function and survival are strictly dependent upon the continuous flow of oxygen-ated blood through the coronary arteries. The manifestations of IHD are a direct consequence of the insufficient delivery of oxygen to the heart. The clinical presentation may include one or more of the following cardiac syndromes: - **Angina pectoris (literally, "chest pain").** Ischemia induces pain but is insufficient to cause myocyte death. Angina can be stable (occurring predictably at certain levels of exertion), can be caused by vessel spasm (vasospatic angina, Prinzmetal angina), or can be unstable (occurring with progressively less exertion or even at rest). - **Myocardial infarction (MI).** This occurs when the severity or duration of ischemia is sufficient to cause cardiomyocyte death. - **Chronic IHD with CHF.** This progressive cardiac decompensation, which occurs after acute MI or secondary to the accumulated effect of multiple ischemic insults, eventually precipitates mechanical pump failure. - **Sudden cardiac death (SCD).** This occurs as a consequence of myocardial ischemia that induces a lethal ventricular fibrillation. Typically, there is underlying coronary artery disease. The term acute coronary syndrome is applied to any of the three catastrophic manifestations of IHD: unstable angina, MI, and SCD. ## Epidemiology About 800,000 Americans experience an MI each year, and roughly half of those affected die. As troubling as this toll is, it represents spectacular progress: since peaking in 1963, the mortality related to IHD in the United States has declined by 50%. The improvement is largely attributed to interventions that have diminished cardiac risk factors (behaviors or conditions that promote atherosclerosis; Chapter 8), in particular smoking cessation programs, hypertension and diabetes treatment, and use of cholesterol-lowering agents. To a lesser extent, diagnostic and therapeutic advances have also contributed; these include aspirin prophylaxis, better arrhythmia control, establishment of coronary care units, thrombolysis for MI, angioplasty and endovascular stenting, use of ventricular assist devices, and coronary artery bypass graft surgery. Maintaining this downward trend in mortality will be particularly challenging given the predicted longevity of "baby boomers," as well as the epidemic of obesity that is sweeping the United States and other parts of the world. ## Pathogenesis of Ischemic Heart Disease IHD is a consequence of inadequate coronary perfusion relative to myocardial demand. In the vast majority of cases this is due to either or both of the following: - Preexisting ("fixed") atherosclerotic occlusion of the coronary arteries - Acute plaque change with superimposed thrombosis and/or vasospasm Next we will discuss each of these two factors in greater detail. ### Chronic Vascular Occlusion Fixed obstructions that occlude less than 70% of a coronary vessel lumen are typically asymptomatic, even with exertion. In comparison, lesions that occlude more than 70% of a vessel lumen-resulting in so-called "critical stenosis"-generally cause symptoms in the setting of increased demand; with critical stenosis, certain levels of exertion predictably cause chest pain, and the patient is said to have stable angina. A fixed stenosis that occludes 90% or more of a vascular lumen can lead to inadequate coronary blood flow with symptoms even at rest-one of the forms of unstable angina (discussed later in the chapter). Atherosclerotic narrowing can affect any of the coronary arteries-left anterior descending (LAD), left circumflex (LCX), and right coronary artery (RCA)-singly or in combination. Clinically significant plaques tend to occur within the first several centimeters of the LAD and LCX takeoff from the aorta, and along the entire length of the RCA. Sometimes, secondary branches are also involved (i.e., diagonal branches of the LAD, obtuse marginal branches of the LCX, or posterior descending branch of the RCA). Of note, if an atherosclerotic lesion progressively occludes a coronary artery at a slow rate over years, other coronary vessels may undergo remodeling and provide compensatory blood flow to the area at risk; such collateral perfusion can protect against MI, even if the original vessel becomes completely occluded. Unfortunately, with acute coronary blockage, there is no time for collateral flow to develop, and infarction results. Vasoconstriction directly compromises lumen diameter; moreover, by increasing local mechanical shear forces, vessel spasm can cause plaque disruption. Vasoconstriction in atherosclerotic plaques can be stimulated by the following: - Circulating adrenergic agonists - Locally released platelet contents - Imbalance between endothelial cell-relaxing factors (e.g., nitric oxide) and endothelial cell-contracting factors (e.g., endothelin) due to endothelial dysfunction - Mediators released from perivascular inflammatory cells ### Acute Plaque Change In most patients, unstable angina, infarction, and sudden cardiac death occur because of abrupt plaque change followed by thrombosis-hence the term acute coronary syndrome (Fig. 9.7). * **What is the initiating event?** The initiating event is typically a sudden disruption (rupture or erosion) of a partially occlusive plaque. Rupture, fissuring, ulceration, or erosion of plaques exposes highly thrombogenic constituents or underlying subendothelial basement membrane, leading to rapid thrombosis. * **Why does plaque volume expand?** In addition, hemorrhage into the core of plaques can expand plaque volume, thereby acutely exacerbating the degree of luminal occlusion. * **How can thrombus cause infarction?** Even partial luminal occlusion by a thrombus can compromise blood flow sufficiently to cause an infarction of the innermost zone of the myocardium (subendocardial infarct). Mural thrombi in a coronary artery can also embolize; indeed, small emboli may be found in the distal intramyocardial circulation (along with associated microinfarcts) at autopsy of patients with unstable angina. * **What does complete obstruction cause?** Most seriously, completely obstructive thrombus over a disrupted plaque typically results in MI. Factors that trigger loss of endothelial cells without plaque rupture (plaque erosion) include endothelial injury and apoptosis, likely attributable to some combination of inflammatory and toxic exposures. Acute plaque rupture, on the other hand, involves factors that influence plaque susceptibility to disruption by mechanical stress. These include intrinsic aspects of plaque composition and structure (Chapter 8) and extrinsic factors, such as blood pressure and platelet reactivity: * **What are vulnerable plaques?** Plaques that contain large atheromatous cores or have thin overlying fibrous caps are more likely to rupture and are therefore termed vulnerable. Fissures frequently occur at the junction of the fibrous cap and the adjacent normal (plaque-free) arterial segment, where the mechanical stresses are highest and the fibrous cap is thinnest. Fibrous caps are also continuously remodeling; the overall balance of collagen synthesis and degradation within the plaque determines its mechanical strength and stability. Collagen is produced by smooth muscle cells and degraded by the action of metalloproteases elaborated by macrophages. Consequently, atherosclerotic lesions with a paucity of smooth muscle cells or large numbers of inflammatory cells are vulnerable to rupture. Of interest, statins may have a beneficial effect not only by reducing circulating cholesterol levels but also by multiple poorly understood effects on atherogenesis. These include reversal of endothelial dysfunctions and stabilizing plaques through a reduction in plaque inflammation. * **What is 'culprit lesion'?** In a majority of cases, the "culprit lesion" in patients who experience an MI was not critically stenotic or even symptomatic before its rupture. As noted previously, anginal symptoms typically occur with fixed lesions exhibiting greater than 70% chronic occlusion. Pathologic and clinical studies show that two-thirds of ruptured plaques are less than or equal to 50% stenotic before plaque rupture, and 85% exhibit significant stenotic occlusion of less than or equal to 70%. Thus, it is sobering to note that a large number of asymptomatic adults are at significant risk for a catastrophic coronary event. At present, it is impossible to predict plaque rupture in any given patient. Plaque disruption and ensuing nonocclusive thrombosis are additional common, repetitive, and often clinically silent complications of atheromas. The healing of subclinical disrupted plaques is an important mechanism by which atherosclerotic lesions progressively enlarge (see Fig. 9.7). ## Angina Pectoris Angina pectoris is intermittent chest discomfort or pain caused by transient, reversible myocardial ischemia that is insufficient to cause myocyte necrosis. It is a consequence of the ischemia-induced release of adenosine, bradykinin, and other molecules that stimulate autonomic nerves. Three variants are recognized: - **Typical or stable angina** is predictable episodic chest discomfort associated with exertion or some other cause of increased demand (e.g., tachycardia). The discomfort is described as a crushing or squeezing substernal sensation radiating down the left arm or to the left jaw (referred pain). The discomfort is usually relieved by rest (reducing demand) or by medications such as nitroglycerin, a vasodilator that increases coronary perfusion. - **Prinzmetal or variant angina** occurs at rest and is caused by coronary artery spasm. Although vasospasm typically involves atherosclerotic vessels, a completely healthy vessel can be affected. Prinzmetal angina typically responds promptly to vasodilators such as nitroglycerin and calcium channel blockers. - **Unstable angina** is characterized by increasingly frequent chest pain, precipitated by progressively less exertion or occurring at rest. Unstable angina is associated with plaque disruption and superimposed thrombosis, distal embolization of the thrombus, and/or vasospasm. Recent studies have shown that the majority of cases of unstable angina are associated with evidence of myocyte injury, and such patients are treated aggressively to limit irreversible myocardial damage. ## Myocardial Infarction Myocardial infarction (MI), also commonly referred to as "heart attack," is necrosis of the heart muscle resulting from ischemia. The 2018 joint task force of U.S. and European Cardiology groups defines MI "as the presence of acute myocardial injury detected by abnormal cardiac biomarkers in the setting of evidence of acute myocardial ischemia." The major underlying cause of IHD is atherosclerosis; while MIs can occur at virtually any age, the frequency rises progressively with aging and with increasing risk factors for atherosclerosis (Chapter 8). Nevertheless, approximately 10% of MIs occur before 40 years of age, and 45% occur before 65 years of age. Men are at greater risk than women, but the gap progressively narrows with age. In general, women tend to be protected against MI during their reproductive years. However, menopause-with declining estrogen production-is associated with exacerbation of coronary artery disease, and IHD is the most common cause of death in older adult women. **Pathogenesis.** The vast majority of MIs are caused by acute thrombosis within coronary arteries (see Fig. 9.7). In most instances, disruption or erosion of preexisting atherosclerotic plaque serves as the nidus for thrombus generation and consequent vascular occlusion. In 10% of MIs, however, transmural infarction occurs in the absence of occlusive atherosclerotic vascular disease; such infarcts are mostly ascribed to coronary artery vasospasm or to embolization from mural thrombi (e.g., in the setting of atrial fibrillation) or from valve vegetations. Occasionally, especially with infarcts limited to the innermost (subendocardial) myocardium, thrombi or emboli are absent. In such cases, severe fixed coronary atherosclerosis leads to marginal perfusion of the heart (eFig. 9.1). In this setting, a prolonged period of increased demand (e.g., due to tachycardia or hypertension) can lead to ischemic necrosis of endomyocardium, the portion of the heart that is most distal to the epicardial vessels. Finally, ischemia without detectable atherosclerosis or thromboembolic disease can be caused by disorders of small intramyocardial arterioles, including vasculitis, amyloid deposition, or stasis, as in sickle cell disease. ### Coronary Artery Occlusion In a typical MI, the following sequence of events takes place: 1. An atheromatous plaque is eroded or suddenly disrupted by endothelial injury, intraplaque hemorrhage, or mechanical forces, exposing subendothelial collagen and necrotic plaque contents to the blood. 2. Platelets adhere, aggregate, and are activated, releasing thromboxane A2, adenosine diphosphate (ADP), and serotonin, all of which cause further platelet aggregation and vasospasm (Chapter 3). 3. Activation of coagulation by exposure of tissue factor adds to the growing thrombus. 4. Within minutes, the enlarging thrombus may completely occlude the coronary artery lumen. The evidence for this scenario derives from autopsy studies of patients dying of acute MI, as well as imaging studies demonstrating a high frequency of thrombotic occlusion early after MI. Angiography performed within 4 hours of the onset of MI demonstrates coronary thrombosis (eFig. 9.2) in almost 90% of cases. When angiography is performed 12 to 24 hours after onset of symptoms, however, evidence of thrombosis is seen in only 60% of patients, even without intervention. Thus, at least some occlusions clear spontaneously through lysis of the thrombus or relaxation of spasm. This sequence of events in a typical MI also has therapeutic implications: early thrombolysis and/or angioplasty can be highly successful in limiting the extent of myocardial necrosis. ### Myocardial Response to Ischemia Loss of blood supply has profound functional, biochemical, and morphologic consequences for the myocardium. Within seconds of vascular obstruction, aerobic metabolism ceases, leading to a drop in adenosine triphosphate (ATP) and accumulation of potentially noxious metabolites (e.g., lactic acid) in cardiac myocytes. The functional consequence is a rapid loss of contractility, occurring within minutes of the onset of ischemia. These early changes are reversible, but if ischemia persists for 20 to 40 minutes, it leads to irreversible damage and coagulative necrosis of myocytes. The earliest detectable feature of myocyte necrosis is disruption of the integrity of the sarcolemmal membrane, allowing intracellular macromolecules to leak out of necrotic cells into the cardiac interstitium and the vasculature (Chapter 1). If blood flow is restored before irreversible injury occurs, myocardium can be preserved; this is the goal of early diagnosis and prompt intervention by thrombolysis or angioplasty. However, as discussed later, reperfusion can have deleterious effects. Even when reperfusion is timely, postischemic myocardium can be profoundly dysfunctional for a number of days due to persistent abnormalities in cellular biochemistry that result in a noncontractile state (stunned myocardium) which may be sufficiently severe to produce transient but reversible cardiac failure. Myocardial ischemia also contributes to arrhythmias, probably by causing electrical instability (irritability) of ischemic regions of the heart. Although massive myocardial damage can cause fatal mechanical failure, in 80% to 90% of cases cardiac death in the setting of myocardial ischemia is due to ventricular fibrillation caused by myocardial irritability. Irreversible injury of ischemic myocytes first occurs in the subendocardial zone (Fig. 9.8). As mentioned, this region is especially susceptible to ischemia, as it is the last area to receive blood delivered by the epicardial vessels and is exposed to relatively high intramural pressures, which impede the inflow of blood. With more prolonged ischemia, a wavefront of cell death moves through other regions of the myocardium, driven by progressive tissue edema and myocardial-derived reactive oxygen species and inflammatory mediators. In the absence of intervention, an infarct caused by occlusion of an epicardial vessel can involve the entire wall thickness (transmural infarct). An infarct achieves its full extent in 3 to 6 hours. Clinical intervention within this critical window of time can lessen the size of the infarct. ### Patterns of Infarction The location, size, and morphologic features of an acute myocardial infarct depend on multiple factors: - Size and distribution of the involved vessel (Fig. 9.9) - Rate of development and duration of the occlusion - Metabolic demands of the myocardium (affected, for example, by blood pressure and heart rate) - Extent of collateral supply Acute occlusion of the proximal left anterior descending (LAD) artery is the cause of 40% to 50% of all MIs and typically results in infarction of the anterior wall of the left ventricle, the anterior two-thirds of the ventricular septum, and most of the heart apex; acute proximal obstructions are often fatal while more distal occlusion of the same vessel may affect only the apex. Similarly, acute occlusion of the proximal left circumflex (LCX) artery (seen in 15% to 20% of MIs) causes necrosis of the lateral left ventricle, and proximal right coronary artery (RCA) occlusion (30% to 40% of MIs) affects much of the right ventricle. The posterior third of the septum and the posterior left ventricle are perfused by the posterior descending artery. The posterior descending artery can arise from either the RCA (in 90% of individuals) or the LCX. By convention, the coronary artery-either RCA or LCX-that gives rise to the posterior descending artery and thereby perfuses portions of the inferior/posterior left ventricle and the posterior third of the septum is considered the dominant vessel. Thus, in a right dominant heart, occlusion of the RCA leads to posterior septal and posterior wall ischemic injury. In comparison, in a left dominant heart, where the posterior descending artery arises from the circumflex artery, occlusion of the LCX generally affects the left lateral wall as well as the posterior third of the septum, and the inferior and posterior wall of the left ventricle. Occlusions can also occur within secondary branches, such as the diagonal branches of the LAD artery or marginal branches of the LCX artery. Atherosclerosis is primarily a disease of epicardial vessels; significant atherosclerosis or thrombosis of penetrating intramyocardial branches of coronary arteries is rare-although these can be affected by vasculitis, vasospasm, or embolization. Even though the three major coronary arteries are end arteries, they are interconnected by numerous anastomoses (collateral circulation). Gradual narrowing of one artery allows blood to flow from high to low-pressure areas through the collateral channels. In this manner, gradual collateral dilation can provide adequate perfusion to areas of the myocardium despite occlusion of an epicardial vessel. Based on the size of the involved vessel and the degree of collateral circulation, myocardial infarcts may take one of the following patterns: - **Transmural infarctions** involve the full thickness of the ventricle and are caused by epicardial vessel occlusion resulting from atherosclerosis and acute plaque change with occlusive thrombosis. - **Subendocardial infarctions** are MIs limited to the inner third of the myocardium. They occur as a result of plaque disruption followed by a coronary thrombus that is lysed spontaneously or therapeutically before the necrosis becomes transmural. As mentioned earlier, the subendocardial region is most vulnerable to hypoperfusion and hypoxia. Thus, in the setting of severe coronary artery disease, transient decreases in oxygen delivery (as from hypotension, anemia, or pneumonia) or increases in oxygen demand (as with tachycardia or hypertension) can cause subendocardial ischemic injury. Microscopic infarcts occur in the setting of small-vessel occlusions and may not show any diagnostic ECG changes. These can occur in the setting of vasculitis, embolization of valve vegetations or mural thrombi, or vessel spasm due to elevated catecholamines, as may occur in extreme emotional stress, with certain tumors (e.g., pheochromocytoma), or as a consequence of cocaine use. ## Morphology Nearly all transmural infarcts (involving 50% or more of the ventricle thickness) affect at least a portion of the left ventricle and/or interventricular septum. Roughly 15% to 30% of Mls that involve the posterior or posteroseptal wall also extend into the right ventricle. Isolated right ventricle infarcts occur in only 1% to 3% of cases of IHD. The gross and microscopic appearance of an MI depends on the age of the injury. Areas of damage progress through a highly characteristic sequence of morphologic changes from coagulative necrosis, to acute and then chronic inflammation, to fibrosis (Table 9.2). Myocardial necrosis proceeds invariably to scar formation without any significant regeneration. Gross and/or microscopic recognition of very recent myocardial infarcts can be challenging, particularly when death occurs within a few hours. ### Myocardial infarcts less than 12 hours old are usually not grossly apparent. However, infarcts more than 3 hours old can be visualized by exposing myocardium to vital stains, such as triphenyl tetrazolium chloride, a substrate for lactate dehydrogenase. Because this enzyme leaks out of damaged cells in the area of ischemic necrosis, the infarcted area discoloration due to stagnant, trapped blood. Thereafter, infarcts become progressively better delineated as soft, yellow-tan areas; by 10 to 14 days, infarcts are rimmed by hyperemic (highly vascularized) granulation tissue. Over the succeeding weeks, the infarcted tissue evolves to a fibrous scar. The microscopic appearance also undergoes a characteristic sequence of changes (see Table 9.2 and Fig. 9 .11). Typical features of coagulative necrosis (Chapter 1) become detectable within 4 to 12 hours of infarction. "Wavy fibers," which reflect the stretching and buckling of noncontractile dead fibers, may be present at the edges of an infarct. Sublethal ischemia may induce intracellular myocyte vacuolization; such myocytes are viable but frequently contract poorly. Necrotic myocardium elicits acute inflammation (typically 1 to 3 days after MI), followed by a wave of macrophages that remove necrotic myocytes and neutrophil fragments (most pronounced 5 to 10 days after MI). The infarcted zone is progressively replaced by granulation tissue by 1 to 2 weeks after MI, which in turn forms the provisional scaffolding upon which dense collagenous scar forms. In most instances, scarring is well advanced by the end of the sixth week, but the extent of repair depends on the size of the original lesion and the ability of the host tissues to heal. Healing requires the migration of inflammatory cells and ingrowth of new vessels from the infarct margins. Thus, an MI heals from its borders toward the center, and a large infarct may not heal as quickly nor as completely as a small one. Moreover, malnutrition, poor vasculature, or exogenous corticosteroids can impede infarct scarring (Chapter 2). Once an MI is completely healed, it is impossible to distinguish its age: whether present for 8 weeks or 10 years, fibrous scars look the same. ### Infarct Modification by Reperfusion The therapeutic goal in acute MI is restoration of tissue perfusion as quickly as possible (hence the adage "time is myocardium"). Such reperfusion is achieved by thrombolysis (dissolution of thrombus by tissue plasminogen activator), angioplasty, or coronary arterial bypass graft. While preservation of a viable (but at-risk) heart can improve short- and long-term outcomes, reperfusion is not an unalloyed blessing because of a phenomenon called reperfusion injury (Chapter 1). The factors that contribute to reperfusion injury include the following: - **Mitochondrial dysfunction.** Ischemia alters mitochondrial membrane permeability, which leads to swelling and rupture of the outer membrane, releasing mitochondrial contents that promote apoptosis. - **Myocyte hypercontracture.** During periods of ischemia, the intracellular levels of calcium increase due to increased influx through the damaged plasma membrane and release from intracellular stores. The increased intracellular calcium leads to cytoskeletal contraction, causing enhanced and uncontrolled myofibril contractions and culminating in cell death. - **Free radicals, including superoxide anion (O2), hydrogen peroxide (H2O2), hypochlorous acid (HOCl), nitric oxide-derived peroxynitrite, and hydroxyl radicals (OH)** are produced within minutes of reperfusion and cause damage to the myocytes by altering membrane proteins and phospholipids. ROS generated by infiltrating leukocytes may also contribute to the damaged vulnerable cells. - **Leukocyte aggregation within the reperfused vessels** may occlude the microvasculature and contribute to the impairment of blood flow, the so-called "no-reflow" phenomenon. This is mediated in part by activation of phospholipase A2, which gives rise to reversible cardiac failure. Myocardial ischemia also contributes to arrhythmias, probably by causing electrical instability (irritability) of ischemic regions of the heart. Although massive myocardial damage can cause fatal mechanical failure, in 80% to 90% of cases cardiac death in the setting of myocardial ischemia is due to ventricular fibrillation caused by myocardial irritability. Irreversible injury of ischemic myocytes first occurs in the subendocardial zone (Fig. 9.8). As mentioned, this region is especially susceptible to ischemia, as it is the last area to receive blood delivered by the epicardial vessels and is exposed to relatively high intramural pressures, which impede the inflow of blood. With more prolonged ischemia, a wavefront of cell death moves through other regions of the myocardium, driven by progressive tissue edema and myocardial-derived reactive oxygen species and inflammatory mediators. In the absence of intervention, an infarct caused by occlusion of an epicardial vessel can involve the entire wall thickness (transmural infarct). An infarct achieves its full extent in 3 to 6 hours. Clinical intervention within this critical window of time can lessen the size of the infarct. ### Patterns of Infarction The location, size, and morphologic features of an acute myocardial infarct depend on multiple factors: - Size and distribution of the involved vessel (Fig. 9.9) - Rate of development and duration of the occlusion - Metabolic demands of the myocardium (affected, for example, by blood pressure and heart rate) - Extent of collateral supply Acute occlusion of the proximal left anterior descending (LAD) artery is the cause of 40% to 50% of all MIs and typically results in infarction of the anterior wall of the left ventricle, the anterior two-thirds of the ventricular septum, and most of the heart apex; acute proximal obstructions are often fatal while more distal occlusion of the same vessel may affect only the apex. Similarly, acute occlusion of the proximal left circumflex (LCX) artery (seen in 15% to 20% of MIs) causes necrosis of the lateral left ventricle, and proximal right coronary artery (RCA) occlusion (30% to 40% of MIs) affects much of the right ventricle. The posterior third of the septum and the posterior left ventricle are perfused by the posterior descending artery. The posterior descending artery can arise from either the RCA (in 90% of individuals) or the LCX. By convention, the coronary artery-either RCA or LCX-that gives rise to the posterior descending artery and thereby perfuses portions of the inferior/posterior left ventricle and the posterior third of the septum is considered the dominant vessel. Thus, in a right dominant heart, occlusion of the RCA leads to posterior septal and posterior wall ischemic injury. In comparison, in a left dominant heart, where the posterior descending artery arises from the circumflex artery, occlusion of the LCX generally affects the left lateral wall as well as the posterior third of the septum, and the inferior and posterior wall of the left ventricle. Occlusions can also occur within secondary branches, such as the diagonal branches of the LAD artery or marginal branches of the LCX artery. Atherosclerosis is primarily a disease of epicardial vessels; significant atherosclerosis or thrombosis of penetrating intramyocardial branches of coronary arteries is rare-although these can be affected by vasculitis, vasospasm, or embolization. Even though the three major coronary arteries are end arteries, they are interconnected by numerous anastomoses (collateral circulation). Gradual narrowing of one artery allows blood to flow from high to low-pressure areas through the collateral channels. In this manner, gradual collateral dilation can provide adequate perfusion to areas of the myocardium despite occlusion of an epicardial vessel. Based on the size of the involved vessel and the degree of collateral circulation, myocardial infarcts may take one of the following patterns: - **Transmural infarctions** involve the full thickness of the ventricle and are caused by epicardial vessel occlusion resulting from atherosclerosis and acute plaque change with occlusive thrombosis. - **Subendocardial infarctions** are MIs limited to the inner third of the myocardium. They occur as a result of plaque disruption followed by a coronary thrombus that is lysed spontaneously or therapeutically before the necrosis becomes transmural. As mentioned earlier, the subendocardial region is most vulnerable to hypoperfusion and hypoxia. Thus, in the setting of severe coronary artery disease, transient decreases in oxygen delivery (as from hypotension, anemia, or pneumonia) or increases in oxygen demand (as with tachycardia or hypertension) can cause subendocardial ischemic injury. Microscopic infarcts occur in the setting of small-vessel occlusions and may not show any diagnostic ECG changes. These can occur in the setting of vasculitis, embolization of valve vegetations or mural thrombi, or vessel spasm due to elevated catecholamines, as may occur in extreme emotional stress, with certain tumors (e.g., pheochromocytoma), or as a consequence of cocaine use. ## Morphology Nearly all transmural infarcts (involving 50% or more of the ventricle thickness) affect at least a portion of the left ventricle and/or interventricular septum. Roughly 15% to 30% of Mls that involve the posterior or posteroseptal wall also extend into the right ventricle. Isolated right ventricle infarcts occur in only 1% to 3% of cases of IHD. The gross and microscopic appearance of an MI depends on the age of the injury. Areas of damage progress through a highly characteristic sequence of morphologic changes from coagulative necrosis, to acute and then chronic inflammation, to fibrosis (Table 9.2). Myocardial necrosis proceeds invariably to scar formation without any significant regeneration. Gross and/or microscopic recognition of very recent myocardial infarcts can be challenging, particularly when death occurs within a few hours. ### Myocardial infarcts less than 12 hours old are usually not grossly apparent. However, infarcts more than 3 hours old can be visualized by exposing myocardium to vital stains, such as triphenyl tetrazolium chloride, a substrate for lactate dehydrogenase. Because this enzyme leaks out of damaged cells in the area of ischemic necrosis, the infarcted area discoloration due to stagnant, trapped blood. Thereafter, infarcts become progressively better delineated as soft, yellow-tan areas; by 10 to 14 days, infarcts are rimmed by hyperemic (highly vascularized) granulation tissue. Over the succeeding weeks, the infarcted tissue evolves to a fibrous scar. The microscopic appearance also undergoes a characteristic sequence of changes (see Table 9.2 and Fig. 9 .11). Typical features of coagulative necrosis (Chapter 1) become detectable within 4 to 12 hours of infarction. "Wavy fibers," which reflect the stretching and buckling of noncontractile dead fibers, may be present at the edges of an infarct. Sublethal ischemia may induce intracellular myocyte vacuolization; such myocytes are viable but frequently contract poorly. Necrotic myocardium elicits acute inflammation (typically 1 to 3 days after MI), followed by a wave of macrophages that remove necrotic myocytes and neutrophil fragments (most pronounced 5 to 10 days after MI). The infarcted zone is progressively replaced by granulation tissue by 1 to 2 weeks after MI, which in turn forms the provisional scaffolding upon which dense collagenous scar forms. In most instances, scarring is well advanced by the end of the sixth week, but the extent of repair depends on the size of the original lesion and the ability of the host tissues to heal. Healing requires the migration of inflammatory cells and ingrowth of new vessels from the infarct margins. Thus, an MI heals from its borders toward the center, and a large infarct may not heal as quickly nor as completely as a small one. Moreover, malnutrition, poor vasculature, or exogenous corticosteroids can impede infarct scarring (Chapter 2). Once an MI is completely healed, it is impossible to distinguish its age: whether present for 8 weeks or 10 years, fibrous scars look the same. ### Infarct Modification by Reperfusion The therapeutic goal in acute MI is restoration of tissue perfusion as quickly as possible (hence the adage "time is myocardium"). Such reperfusion is achieved by thrombolysis (dissolution of thrombus by tissue plasminogen activator), angioplasty, or coronary arterial bypass graft. While preservation of a viable (but at-risk) heart can improve short- and long-term outcomes, reperfusion is not an unalloyed blessing because of a phenomenon called reperfusion injury (Chapter 1). The factors that contribute to reperfusion injury include the following: - **Mitochondrial dysfunction.** Ischemia alters mitochondrial membrane permeability, which leads to swelling and rupture of the outer membrane, releasing mitochondrial contents that promote apoptosis. - **Myocyte hypercontracture.** During periods of ischemia, the intracellular levels of calcium increase due to increased influx through the damaged plasma membrane and release from intracellular stores. The increased intracellular calcium leads to cytoskeletal contraction, causing enhanced and uncontrolled myofibril contractions and culminating in cell death. - **Free radicals, including superoxide anion (O2), hydrogen peroxide (H2O2), hypochlorous acid (HOCl), nitric oxide-derived peroxynitrite, and hydroxyl radicals (OH)** are produced within minutes of reperfusion and cause damage to the myocytes by altering membrane proteins and phospholipids. ROS generated by infiltrating leukocytes may also contribute to the damaged vulnerable cells. - **Leukocyte aggregation within the reperfused vessels** may occlude the microvasculature and contribute to the impairment of blood flow, the so-called "no-reflow" phenomenon. This is mediated in part by activation of phospholipase A2, which gives rise to reversible cardiac failure. Myocardial ischemia also contributes to arrhythmias, probably by causing electrical instability (irritability) of ischemic regions of the heart. Although massive myocardial damage can cause fatal mechanical failure, in 80% to 90% of cases cardiac death in the setting of myocardial ischemia is due to ventricular fibrillation caused by myocardial irritability. Irreversible injury of ischemic myocytes first occurs in the subendocardial zone (Fig. 9.8). As mentioned, this region is especially susceptible to ischemia, as it is the last area to receive blood delivered by the epicardial vessels and is exposed to relatively high intramural pressures, which impede the inflow of blood. With more prolonged ischemia, a wavefront of cell death moves through other regions of the myocardium, driven by progressive tissue edema and myocardial-derived reactive oxygen species and inflammatory mediators. In the absence of intervention, an infarct caused by occlusion of an epicardial vessel can involve the entire wall thickness (transmural infarct). An infarct achieves its full extent in 3 to 6 hours. Clinical intervention within this critical window of time can lessen the size of the infarct. ### Patterns of Infarction The location, size, and morphologic features of an acute myocardial infarct depend on multiple factors: - Size and distribution of the involved vessel (Fig. 9.9) - Rate of development and duration of the occlusion - Metabolic demands of the myocardium (affected, for example, by blood pressure and heart rate) - Extent of collateral supply Acute occlusion of the proximal left anterior descending (LAD) artery is the cause of 40% to 50% of all MIs and typically results in infarction of the anterior wall of the left ventricle, the anterior two-thirds of the ventricular septum, and most of the heart apex; acute proximal obstructions are often fatal while more distal occlusion of the same vessel may affect only the apex. Similarly, acute occlusion of the proximal left circumflex (LCX) artery (seen in 15% to 20% of MIs) causes necrosis of the lateral left ventricle, and proximal right coronary artery (RCA) occlusion (30% to 40% of MIs) affects much of the right ventricle. The posterior third of the septum and the posterior left ventricle are perfused by the posterior descending artery. The posterior descending artery can arise from either the RCA (in 90% of individuals) or the LCX. By convention, the coronary artery-either RCA or LCX-that gives rise to the posterior descending artery and thereby perfuses portions of the inferior/posterior left ventricle and the posterior third of the septum is considered the dominant vessel. Thus, in a right dominant heart, occlusion of the RCA leads to posterior septal and posterior wall ischemic injury. In comparison, in a left dominant heart, where the posterior descending artery arises from the circumflex artery, occlusion of the LCX generally affects the left lateral wall as well as the posterior third of the septum, and the inferior and posterior wall of the left ventricle. Occlusions can also occur within secondary branches, such as the diagonal branches of the LAD artery or marginal branches of the LCX artery. Atherosclerosis is primarily a disease of epicardial vessels; significant atherosclerosis or thrombosis of penetrating intramyocardial branches of coronary arteries is rare-although these can be affected by vasculitis, vasospasm, or embolization. Even though the three major coronary arteries are end arteries, they are interconnected by numerous anastomoses (collateral circulation). Gradual narrowing of one artery allows blood to flow from high to low-pressure areas through the collateral channels. In this manner, gradual collateral dilation can provide adequate perfusion to areas of the myocardium despite occlusion of an epicardial vessel. Based on the size of the