Autoimmune Encephalitis and Related Nervous System Disorders PDF
Document Details
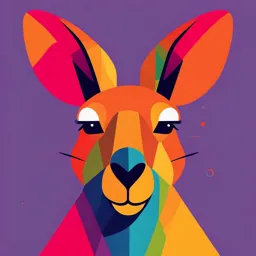
Uploaded by ClearerFantasy7179
2022
Josep Dalmau, Francesc Graus
Tags
Summary
This chapter from a book on autoimmune encephalitis and related nervous system disorders discusses the pathogenesis and disease mechanisms. It examines antibody pathogenicity, neuropathological findings, and the interaction of antibodies with neuronal cell surfaces. The chapter also highlights the differences between antibody-mediated and T cell-mediated autoimmune encephalitis and includes a table summarizing antibody associations with tumours and their potential trigger of herpes simplex encephalitis.
Full Transcript
Chapter 3 - Pathogenesis and Disease Mechanisms in Neuronal Antibody-Mediated Encephalitis from Section 1 - Overview Published online by Cambridge University Press: 27 January 2022 Josep Dalmau and Francesc Graus 1. 3.1 General Concepts on Antibody Pathogenicity and Disease Mechanisms 42 2. 3.2...
Chapter 3 - Pathogenesis and Disease Mechanisms in Neuronal Antibody-Mediated Encephalitis from Section 1 - Overview Published online by Cambridge University Press: 27 January 2022 Josep Dalmau and Francesc Graus 1. 3.1 General Concepts on Antibody Pathogenicity and Disease Mechanisms 42 2. 3.2 Neuropathological Findings 44 3. 3.3 Antibody Properties, Class, and Subclass of Immunoglobulins 44 4. 3.4 Triggers and Genetic Susceptibility of Autoimmune Encephalitis 48 5. 3.5 Functional Interaction of Antibodies with Neuronal Cell Surface and Synaptic Proteins 59 3.1 General Concepts on Antibody Pathogenicity and Disease Mechanisms The concept that some diseases of the central nervous system (CNS) can be mediated by neuronal antibodies has evolved over the years largely influenced by the study of two groups of disorders, the paraneoplastic syndromes of the CNS, which contributed to the 1 discovery of pathogenic antibodies against neuronal cell surface targets, and the myasthenic syndromes, which helped to understand how these antibodies can alter 2–5 synaptic function (see the historical overview in Chapter 1). Paraneoplastic syndromes of the CNS are remote effects of cancer that are frequently associated with immune responses against intracellular proteins expressed by neurons 6 and the associated cancer (onconeural antigens; see Chapter 16). It has been postulated that the ectopic expression of these neuronal proteins by the tumour triggers an immune response that in some patients is enhanced and misdirected against the 7 nervous system. In addition to onconeural antibodies (or classical paraneoplastic antibodies), these patients develop cytotoxic T cell responses against the nervous system 8 that are considered the responsible mediators of the neurological syndrome. In contrast to the antibodies that occur in many paraneoplastic and other neurologic diseases in which the antigens are intracellular, the antibodies associated to myasthenic syndromes (myasthenia gravis or Lambert–Eaton syndrome (LEMS)) can access their cognate cell surface antigens (acetylcholine receptor (AChR) and the voltage-gated calcium channels (VGCC)) and alter their structure and function. In these disorders, the antibodies have direct pathogenic effects by several different mechanisms, such as crosslinking and internalization of the receptors, functional blocking of the target, or 2, 3, 4, 5 complement-mediated alterations in the neuromuscular junction. Currently, there are 19 disorders of the CNS in which the associated antibodies are directed against neuronal cell surface proteins, including excitatory or inhibitory neuronal receptors, and proteins involved in somatodendritic signal integration, clustering and modulation of receptors, synaptic vesicle reuptake, or synaptogenesis 9 (Table 3.1). For most of these disorders the accessibility of the cell surface antigens to circulating antibodies and the reversibility of patients’ symptoms after removing the antibodies or antibody-producing cells place them pathophysiologically closer to the antibody-mediated myasthenic syndromes than to the CNS syndromes in which the antibodies target intracellular proteins, but the main alterations are T cell mediated. Despite these pathophysiological differences, the encephalitis with antibodies against 10–12 cell surface proteins can also occur as paraneoplastic manifestations of cancer, but even in these cases the frequent response to immunological and oncological therapies separate them from the absent or more limited response to treatment of the T cell- 13–15 mediated paraneoplastic syndromes (see definitions and nomenclature in Chapter 1). Table 3.1 Neuronal surface antibodies, triggers, and genetic susceptibility Frequency of Can be tumour triggered by association, and herpes main types of simplex HLA Antibody IgG subclass tumours encephalitis associations Antibodies against neuronal surface receptors Varies with age and Unclear; a suggested sex ; 56% of women associations IgG1 with or 19–21 NMDAR 16 18–45 years old have Yes with without IgG3 17, 22 ovarian teratoma B*07:02, 18 23 DRB1*16:02 b ~60% (SCLC, AMPAR IgG1 11 No N/A thymoma, breast) Limited information: b GluK2R IgG1 1 of 7 patients had No N/A Hodgkin lymphoma b 24 25, 24 GABAaR IgG1 27% (thymoma) Yes N/A b 10, 12 GABAbR IgG1 ~50% (SCLC) No N/A 3 of 26 (11%); 2 Hodgkin lymphoma, 26 mGluR1 IgG1 1 T cell lymphoma, No N/A and prostate 26 adenocarcinoma Limited information: 1 of 2 cases 27 rhabdomyosarcoma, mGluR2 IgG1 and the other small- No N/A cell neuroendocrine 27 cancer IgG1 with or 6/11 (Hodgkin mGluR5 28 28 No N/A without IgG3 lymphoma) 29 30 D2R unknown 0% Yes N/A Frequency of Can be tumour triggered by association, and herpes main types of simplex HLA Antibody IgG subclass tumours encephalitis associations Antibodies against neuronal surface receptors ~20% (thymoma, B 31 GlyR IgG1, IgG3 cell lymphoma, Unknown N/A 31 breast cancer) Antibodies against other neuronal cell surface proteins DRB1*07:01; IgG4 with or 33, DQA1*02:01 without IgG1 80% if b associated with P/Q-type VGCC IgG1 cerebellar Unknown N/A 51 syndrome; about 50% if associated 52 with LEMS b >80% SCLC, breast Amphiphysin IgG1 53–55 No N/A cancer a The association with teratoma is sex and age dependent. While young adult females frequently have an ovarian teratoma, the presence of a tumour is uncommon in children or young adult males. b Unpublished, based on the experience of the authors. AMPAR: α-amino-3-hydroxy-5-methyl-4-isoxazolepropionic acid; CASPR2: contactin- associated protein-like 2; D2R: dopamine 2 receptor; DNER: delta/notch-like epidermal growth factor-related receptor; DPPX: dipeptidyl-peptidase-like protein 6; GABAaR: gamma-aminobutyric acid A receptor; GABAbR: gamma-aminobutyric acid B receptor; GlyR: glycine receptor; GluK2R: glutamate ionotropic kainate 2 receptor; IgLON5: Ig-like domain-containing protein 5; LGI1: leucine-rich, glioma inactivated 1; mGluR: metabotropic glutamate receptor; NMDAR: N-methyl-D-aspartate receptor; SEZ6L2: seizure-related 6 homolog like 2; VGCC: voltage-gated calcium channels. In addition to the immunologic similarities with the myasthenic syndromes and frequent response to immunotherapy, a direct pathogenic role of the antibodies associated with autoimmune encephalitis was suggested by the clinical, pathological, and immunological features described below and summarized in Box 3.1. Box 3.1 General Clinical and Immunological Findings Supporting Antibody Pathogenicity in Autoimmune Encephalitis Similarity between the clinical phenotypes of autoimmune encephalitis and those obtained when the same antigens are altered genetically or pharmacologically (e.g., anti- 17 NMDAR encephalitis and effects of non-competitive antagonists of NMDAR such as 56–58 59 ketamine or phencyclidine; or anti-GlyR encephalitis and hereditary hyperekplexia 60 or strychnine poisoning ). Reversibility of patients’ symptoms with treatments aimed to removing the antibodies or antibody-producing cells. Neuropathological findings (e.g., infiltrates of B or plasma cells predominate over cytotoxic T cells; presence of IgG; absence or limited number of neuronophagic cells or 61, 62 clusters; absent or mild neuronal loss ) which are remarkably different from those observed in paraneoplastic and other autoimmune encephalitis mediated by cytotoxic T cells (e.g., extensive infiltrates of T cells and frequent neuronophagic cells or clusters; 63 frequent neuronal loss and neurodegeneration ). Accessibility of antibodies to antigenic epitopes exposed on the neuronal cell surface. Evidence of structural and functional effects of patients’ antibodies in cultured 64–67 68–73 neurons or animal models of passive antibody transfer or active 74, 75 immunization. Reversibility of antibody effects in animal models using modulators of the target antigen 76 77 (e.g., ephrin B2 receptor activation or allosteric modulation of the NMDAR ). 3.2 Neuropathological Findings Pathological studies on autoimmune encephalitis are scarce, mainly due to the partial or complete recovery of most patients; however, when available the findings are remarkably different from those reported in patients with cytotoxic T cell-mediated mechanisms, including not only the paraneoplastic syndromes with onconeural antibodies but also the 63 78 non-cancer-related encephalitis with GAD65 or AK5 antibodies. In these disorders biopsy and autopsy studies usually show frequent and extensive infiltrates of T cells, often in close apposition to neurons or forming neuronophagic clusters of T cells that lead to irreversible neuronal loss accompanied by astrogliosis (see Chapter 6, Figure 6.3). In contrast, brain biopsy studies of patients with anti-NMDA receptor (NMDAR) encephalitis are often normal or show mild inflammatory changes, while autopsy studies show predominant B cells or plasmablasts/plasma cells, with limited infiltrates of T cells that are almost never in close apposition to neurons or forming neuronophagic clusters 61, 62 (see Figure 16.2). Biopsy and autopsy reports from other types of autoimmune encephalitis are uncommon, but in general all show paucity of T cell infiltrates, even though in some cases a few T cells are shown in apposition to neurons (see Chapter 2, Case Vignette 2.1 and Figure 2.4). 3.3 Antibody Properties, Class, and Subclass of Immunoglobulins 3.3.1 Immunoglobulin Class For all autoimmune encephalitis, the immunoglobulin class of the antibodies is IgG (Box 3.2). The clinical significance and implications of IgA and IgM antibodies against neuronal surface antigens are unclear. As far as IgA or IgM antibodies against NMDAR are concerned, investigations have focused on patients with neurodegenerative diseases 79–83 84, 85 86 (Parkinson, Alzheimer’s, among others), stroke, cancer, and primary psychiatric disorders (see Chapter 19). Although most of these studies make use of anti- NMDAR encephalitis as the model to support their findings and conclusions, none has provided evidence that the presence of IgA or IgM antibodies defines a specific syndrome or associates with a different outcome when compared with seronegative cases with the same diseases. Therefore, it is unclear whether immunotherapy would ameliorate the symptoms of these patients or change the clinical course of the primary disease (e.g., stroke, Parkinson). An important limitation of most of these studies is that they only examine patients’ serum with a single test (e.g., cell-based assays (CBAs)), without further validation of the results. Importantly, studies claiming the presence of NMDAR antibodies in disorders such as schizophrenia, Parkinson disease, other psychiatric and neurodegenerative diseases, or stroke show a percentage of IgA or IgM 81–83, 87, 88 seropositivity (~10–12%) that is frequently similar to that of healthy subjects. These studies rarely use CSF of patients, and when used it is almost always antibody- negative. Box 3.2 Class and Subclass Immunoglobulins Involved in Autoimmune Encephalitis All antibodies against neuronal cell surface proteins and receptors involved in autoimmune encephalitis are of the IgG class (see Table 3.1). The main IgG subclasses in autoimmune encephalitis are IgG1 (sometimes accompanied by IgG3 or IgG2) and IgG4 (see Table 3.1). 89 16 Anti-NMDAR encephalitis is mediated by IgG antibodies, predominantly IgG1. The significance of IgA or IgM antibodies against NMDAR in neurodegenerative 79, 80 81, 90 81, 82, 88 81, 84, 85 diseases, Parkinson, schizophrenia, stroke, and other 91, 92 neurologic or psychiatric diseases is unclear. IgA or IgM antibodies do not confer any distinct symptoms or syndromes upon these diseases. The same IgA or IgM 91, 93 antibodies are found in similar proportions of healthy subjects. It is unknown whether immunotherapy may improve the symptom severity or outcome of these non- immune neurologic or psychiatric diseases. IgG1 antibodies have two identical arms that display similar epitope specificity, and can 64 94 lead to crosslinking and internalization of the target antigens (e.g., NMDAR, AMPAR, 25 66 95 GABAaR ); functional blocking of the receptors (e.g., GlyR, GABAbR ), or activation of 96 complement and cell damage (e.g., aquaporin 4 antibodies ). IgG4 antibodies have the ability to undergo half-antibody exchange, implying that only one arm of the antibody recognizes the antigen. These antibodies disrupt the interaction 97 of the corresponding target antigen (e.g., LGI1, CASPR2, MuSK) with other proteins. Some studies have found NMDAR IgG antibodies in about ~1–4% of patients with all types 81, 87, 98 of diseases, as well as in healthy controls. Only a few series have shown a frequency of IgG NMDAR antibodies in more than 5% of patients with psychiatric 99, 100 diseases (see Table 19.1). In general, these studies have the same limitations as those mentioned above: only serum tested; lack of distinctive clinical features between seropositive and seronegative cases, lack of validation techniques, and when CSF is tested it is always negative. In addition, there is a lack of consistency of results among different laboratories. Whereas some studies show that the prevalence of antibodies between patients and healthy controls is significantly different, leading the authors to 101–103 develop a set of theories, other studies find no significant differences, leading to 87, 98, 104 completely different interpretations. This discrepancy on the prevalence of IgG NMDAR antibodies among different laboratories is likely explained by the type of 98, 102, 104, 105 techniques used for antibody testing and the extent of the testing. In our experience, serum and CSF IgG antibodies against the GluN1 (or NR1) subunit of the NMDAR occur only in patients who have, or have had, anti-NMDAR encephalitis and 19, 89 in cases of autoimmune encephalitis post-herpes simplex encephalitis (HSE). By contrast, we have not found a specific disease-association or clear clinical implications for 89 IgA or IgM NMDAR antibodies. This experience is based on the systematic use of several techniques for antibody determination (brain immunohistochemistry, cell-based assay, often along with cultured neurons) in paired serum and CSF, which probably explain the high specificity and consistency of the results. A separate set of problems derive from studies that determine antibodies (of any immunoglobulin class) against peptides or linear epitopes (e.g., denatured protein sequence) of the NMDAR. These types of antibodies have been reported in a myriad of diseases (Rasmussen’s encephalitis, viral encephalitis, prion diseases, and stroke, among 106–113 others); the antibodies are detected by ELISA, immunoblot, or other techniques that do not require conformational epitopes, and their clinical significance is unknown. As occur with IgA/IgM antibodies, the detection of antibodies against peptides or linear epitopes of NMDAR does not appear to be associated with any distinct group of symptoms or different outcome. Antibodies against an epitope (five amino acid consensus sequence D/E W D/E Y S/G) expressed by the GluN2A and GluN2B subunits of the NMDAR have been reported in 114 patients with systemic lupus erythematosus and neurolupus. Although the description of these antibodies preceded by six years the discovery of anti-NMDAR encephalitis, their use as surrogate biomarkers of specific neuropsychiatric symptoms in patients with 115–117 lupus has not progressed to routine clinical practice (see Chapters 18 and 19). Therefore, a clear distinction between IgG antibodies against the GluN1 (or NR1) subunit of the NMDAR (characteristic of anti-NMDAR encephalitis) and all other ‘NMDAR antibodies’ is critical for a proper diagnosis, treatment, and prognosis of patients with anti-NMDAR encephalitis and for a better understanding of the mechanisms involved in this disease (Table 3.2). Table 3.2 NMDAR antibodies in human disease Type of NMDAR antibodies Techniques Disease CSF/serum Comments Brain tissue Highly specific for immunostaining; anti-NMDAR IgG against CSF 100% encephalitis (only 100% of conformational cell-based identified in this patients with epitopes of the disease when all assays (CBA), anti-NMDAR Serum 88% amino terminal confirmatory live cultured encephalitis region of GluN1 techniques are neurons 89 used) Described in ~9–19% of patients with many different CBA, rarely diseases No specific Only (psychiatric, IgA or IgM demonstrated disease or present in neurodegenerative, against GluN1 with other syndrome serum stroke) and ~9–11% techniques of healthy 81, 82, 93 subjects. Unclear clinical Type of NMDAR antibodies Techniques Disease CSF/serum Comments significance Described in many disorders: Rasmussen’s encephalitis, ELISA or epilepsia partialis immunoblot continua, viral Any encephalitis, immunoglobulin Not No specific Usually only neurodegenerative class against disease or shown in diseases, linear epitopes demonstrated syndrome serum paraneoplastic of GluN2B with live opsoclonus, neurons encephalitis, Creutzfeldt–Jakob, 108, among others. 113 Unclear clinical 118 significance Suggested as a ELISA or biomarker of immunoblot neurolupus or lupus psychosis. IgG against Systemic lupus Inconsistent Not erythematosus Serum or GluN2A or findings; reported a demonstrated (no syndrome CSF in ~12–14% of sera GluN2B specific) with live of patients with neurons lupus neuropsychiatric 115, 116 events. a Five amino acid consensus sequence (D/E W D/E Y S/G) present in the GluN2a and GluN2B subunits of the NMDAR 3.3.2 IgG Subclass In addition to the immunoglobulin class (IgG, IgA, IgM), it is important to consider the IgG subclass (e.g., IgG1, IgG3, or IgG4) (Table 3.1). Most of the antibodies associated with autoimmune encephalitis are IgG1 (with or without IgG3 or IgG2) and less frequently IgG4, and each type of autoimmune encephalitis characteristically occurs with one or several IgG subclasses. The disease mechanisms of IgG1 (or IgG3) antibodies are substantially different from IgG4 antibodies. The two arms of IgG1 and IgG3 antibodies have the same epitope specificity, whereas IgG4 has the ability to undergo half-antibody 97 exchange, implying that each arm of the antibody recognizes a different antigen. These structural and functional properties of the IgG subclasses are critical to understanding their pathogenic effects. IgG1 or IgG3 antibodies induce crosslinking and internalization 64, 68 of the antigen, as seen for instance in the encephalitis associated with NMDAR, 65, 69 25, 119 AMPA receptor (AMPAR), GABAa receptor (GABAaR), or GluK2Receptor (GluK2R) antibodies (see Chapter 1, Figure 1.2). In addition, IgG1 and IgG3 antibodies can potentially activate complement. In contrast, IgG4 autoantibodies cannot induce crosslinking and internalization of the antigen; instead, they cause inhibition of protein–protein interactions, as shown later in this chapter for the encephalitis 70 39, 120 associated with antibodies against LGI1 and CASPR2. These general properties of IgG4 antibodies apply similarly to the autoimmune diseases of the central or peripheral 121–123 nervous system (e.g., myasthenia with MuSK antibodies; chronic inflammatory 124 demyelinating polyneuropathy with neurofascin antibodies ), as well as to other 125 autoimmune conditions outside the nervous system (e.g., pemphigus ) (Figure 3.1). IgG4 antibodies are not able to activate complement. Moreover, all antigens in the currently known group of IgG4-associated autoimmune diseases are N-linked 97 glycoproteins. This, along with the observed association with HLA DQ5 and DR14 in 126, 127 some IgG4-associated autoimmune diseases (e.g., MuSK myasthenia gravis, 128 pemphigus ), suggests a common underlying aetiology for all of the IgG4-associated 97 autoimmune diseases. It is interesting that among all antibody-mediated encephalitis known to date, the three in which a strong HLA association has been demonstrated are 35–37 those associated with IgG4 (sometimes along with IgG1) antibodies, including LGI1, 37 47, 129 CASPR2, and IgLON5 antibodies. Thus, one can speculate that an altered tolerance to post-translationally modified proteins in combination with certain HLA haplotypes increases susceptibility to developing IgG4-mediated autoimmunity against 97 glycosylated proteins. Of note, GAD autoimmunity associates with IgG1 antibodies and 130, 131 has been linked to HLA susceptibility; however, GAD65 is an intracellular protein and the disease is unlikely mediated by the antibodies. Figure 3.1 IgG4 antibody-mediated mechanisms in pemphigus and MuSK antibody-associated myasthenia gravis. (A) IgG4 antibodies against desmoglein 1 disturb the normal interaction with desmocollin and disrupt cell–cell adhesion, resulting in pemphigus. (B) IgG4 MuSK antibodies disrupt the Agrin–LRP4–MuSK signalling cascade, therefore interfering with AChR clustering. MuSK consists of three Ig-like domains and a Frizzled-like domain. The main immunogenic region of MuSK is located in the first Ig-like domain which is essential for the interaction with LRP4. Titres of antibodies against this domain correlate significantly with disease severity. MuSK antibodies also interfere with the interaction of MuSK with ColQ, although the exact contribution of this to the disease is unknown. AChE, acetylcholinesterase; AChR, acetylcholine receptor; ColQ, collagen-like molecule associated with AChE; LRP4, lipoprotein-related protein 4; MuSK, muscle-specific kinase. (reproduced from reference 97 with permission) 3.3.3 Complement-Mediated Mechanisms The potential role of complement-mediated neuronal injury in all types of autoimmune encephalitis associated with antibodies against neuronal cell surface proteins is unclear. To assess whether complement-mediated mechanisms are pathogenically involved it is critical to investigate tissue from patients rather than developing conclusions from in- 132 vitro studies, which can be misleading. For example, any model consisting in cultured neurons exposed to IgG1 antibodies against a cell surface protein in the presence of complement in the media may suggest that complement-mediated neuronal injury has a pathogenic role. However, biopsy or autopsy material from patients may show that complement-mediated mechanisms are not pathogenically involved. An example that illustrates this problem is anti-NMDAR encephalitis; an in-vitro study using patients’ antibodies suggested that complement-mediated mechanisms played a role in the 132 disease, but investigations using patients’ tissue and clinical and radiological 17, 63, 133 outcomes indicate otherwise. In contrast, disorders in which complement activation plays an important role (such in aquaporin 4-mediated neuromyelitis optica) are associated with frequent MRI alterations consistent with oedema and tissue damage, 96, 134 and limited reversibility of the neurological deficits. It has been suggested that the IgG1 antibodies of patients with anti-LGI1 encephalitis (which in most cases are accompanied by IgG4 antibodies) are able to activate complement leading to pathologic alterations. However, pathological evidence for this comes from a single study that included the biopsy of only one patient with LGI1 63 antibodies. The same study included 3 additional patients with antibodies attributed to voltage-gated potassium channels (VGKC) (all 3 negative for LGI1 antibodies and antigenic target unknown), 3 patients with NMDAR antibodies, and 10 patients with antibodies against intracellular proteins (4 Hu or ANNA1, 3 Ma2, and 3 GAD). Interestingly, only the 4 patients considered VGKC antibody-positive (1 anti-LGI1, 3 antigen unknown) showed immunoglobulin staining on the surface of neurons, and C9neo staining (suggesting activation of the complement cascade) in the cytoplasm and surface of neurons in the hippocampal CA4 region, dentate gyrus, and cortex. In one case in which the antigen was unknown, the presence of complement was associated with 63 evidence of neuronal death. Overall, the significance of these findings is unclear, given that only one case had genuine antibodies against a cell surface antigen (LGI1). Complement deposition was also found in the hippocampus (obtained from surgical resection) of a patient with CASPR2 antibody-associated encephalitis. In addition, there were moderate infiltrates of CD3 and CD8 T cells (not in apposition to neurons), small numbers of B cells and plasma cells, and prominent blood–brain barrier leakage 135 accompanied by deposition of IgG in the membranes of neurons. In patients with anti-NMDAR encephalitis, studies examining side by side the presence of C9neo staining in brain (from biopsy or autopsy) and in nervous tissue contained in the ovarian teratoma of the same patients showed absence of complement activation in neurons of the CNS, but extensive deposits of complement on the surface of neurons 133 present in the tumour (Figure 3.2). These findings, along with the absence of contrast enhancement in MRI studies (a feature common to all types of encephalitis with antibodies against neuronal surface antigens) suggest that the absent or uncommon detection of complement activation in the brain of patients with autoimmune encephalitis is likely due to the integrity of the blood–brain barrier and small amount of components of the complement system in the CNS. Nevertheless, there is evidence that resident cells in the CNS, such as astrocytes, oligodendrocytes, and microglia, are able to synthesize components of the complement system, most notably in response to injury, and that complement factors are known to play important non-immune functions, such 136 as neuronal stem cell differentiation, migration, and modulation of synapse formation. Future studies should investigate the role of complement-mediated injury in autoimmune encephalitis and clinical settings in which the blood–brain barrier is disrupted or there are additional mechanisms of brain injury (e.g., autoimmune encephalitis post-herpes simplex virus or Japanese B encephalitis) to determine if it 19, 137 contributes to the worse clinical outcomes of these patients (discussed later). Figure 3.2 Comparative analysis of complement immunostaining in brain and teratoma of patients with anti-NMDAR encephalitis. (A) and (B) correspond to paraffin-embedded sections of brain of two patients with anti- NMDAR encephalitis, and (C) from a patient without NMDAR antibodies (control). (D–F) are sections of teratomas of patients with anti-NMDAR encephalitis. Brain and teratoma sections were immunostained for C9neo. The patients’ tumours show intense complement immunoreactivity on the surface of neurons and neuronal processes. In contrast, patients and control brains lack complement immunoreactivity (the nuclear staining is not specific). The inset in D shows neurons contained in the tumour stained with MAP2, a specific dendritic marker. Scale = 20 µm. (reproduced from reference 133 with permission) 3.4 Triggers and Genetic Susceptibility of Autoimmune Encephalitis The main triggers or factors that influence the development of antibodies to neural surface antigens include: systemic tumours, viral encephalitis (mainly herpes simplex and Japanese B encephalitis), and HLA susceptibility. These triggers are summarized in Table 3.1 and discussed in the following sections. It is important to keep in mind that even for the best-known diseases in which several triggers have been well defined (e.g., anti- NMDAR encephalitis triggered by teratomas or HSE), many patients do not have any of these triggers. Thus, the cause of the disease in many patients with anti-NMDAR and other antibody-mediated encephalitis is unknown. A theory that is well supported by current data, and is applicable to several types of autoimmune encephalitis, mainly those that can be initiated by tumours or viral infections, is shown in Figure 3.3. Figure 3.3 Proposed mechanisms of disease and functional interactions of autoantibodies with neuronal cell surface proteins. The figure shows a multistep process that results in antibody-mediated neuronal dysfunction; some of the steps have been demonstrated, and others are based on proposed hypotheses. Two known triggers of autoimmune encephalitis are represented: HSE and systemic tumours (A, B). The genetic susceptibility of some autoimmune encephalitis and unknown immunological triggers are not depicted. It is postulated that antigens released by viral-induced neuronal destruction or apoptotic tumour cells are loaded into antigen-presenting cells (dendritic cells) and transported to regional lymph 1 nodes. In the lymph nodes, naive B cells exposed to the processed antigens, with cooperation of CD4+ T cells, become antigen-experienced and differentiate into antibody- producing plasma cells. After entering the brain, memory B cells undergo restimulation, antigen-driven affinity maturation, clonal expansion, and differentiation into antibody- 138 producing plasma cells (C). The contribution of systemically produced antibodies to the pool of antibodies present in the CNS is unclear and may depend on systemic antibody titres and integrity of the blood–brain barrier. Based on experimental models with cultured neurons, the presence of antibodies in the brain may lead to neuronal dysfunction by different mechanisms, including functional blocking of the target antigen (GABAbR antibodies; D), receptor crosslinking and internalization (NMDAR antibodies; E), and disruption of protein–protein interactions leading to downstream effects (LGI1 leading to a decrease of Kv1.1 and AMPAR; F), all discussed in further detail in this chapter. These mechanisms depend on the type of antigen and subclass of IgG; whereas IgG1 antibodies frequently crosslink and internalize the target antigen, IgG4 antibodies alter protein–protein interactions (Table 3.1). APC: antigen-presenting cell. (reproduced from reference 9 with permission) 3.4.1 Tumours 3.4.1.1 Autoimmune Responses Triggered by Tumour Expression of Neural Proteins Different from the paraneoplastic syndromes with antibodies against onconeural (intracellular) proteins that almost always associate with the presence of a systemic cancer, the autoimmune encephalitis with antibodies against neuronal surface proteins can occur with or without a tumour association. Therefore, in this regard the autoimmune encephalitis are similar to the antibody-mediated diseases of the neuromuscular junction that can occur with or without an underlying tumour, such as myasthenia gravis, where the frequency of thymoma is around 10%, and LEMS, in which 139 the frequency of small-cell lung cancer (SCLC) is above 50%. Some autoimmune 33, encephalitis rarely associate with tumours, for example anti-LGI1 or DPPX encephalitis, 34 whereas others show a robust and specific cancer association, such as anti-GABAb 10, 12 receptor (GABAbR) encephalitis and SCLC, or anti-CASPR2 associated Morvan 38, 40 syndrome and thymoma. Overall, the frequency and type of tumour association vary according to the type of neuronal antibody (Table 3.1) and, within a specific disorder it can also vary according to age and sex. For example, in patients with anti-NMDAR encephalitis the presence of a tumour (usually teratoma) is much more frequent in young adult women than in younger girls or males of any age (46% in all female patients vs 6% in girls younger than 17, 18 12 years, or 6% in males of any age). Teratomas of the ovary are by far the most common tumours in this disease; however, in patients older than 44 years the frequency of tumours is lower and they are usually carcinomas (ovary, breast, lung, thymus) instead 140 of teratomas. In anti-GABAaR encephalitis, which can also affect children, tumours, 24 usually thymoma, occur only in adults (27% vs 0% children). For many types of encephalitis with antibodies against neuronal surface antigens, the cancer cells express the same neuronal antigen recognized by patients’ antibodies (Table 3.1). In these disorders (e.g., AMPAR, GABAbR, NMDAR) it has been postulated that the expression of neuronal proteins by the tumour breaks immune tolerance for these proteins, leading to the development of the immune response, similar to the mechanisms proposed for paraneoplastic syndromes mediated by cytotoxic T cell 141 reponses. For the latter group of diseases there is evidence that tumour genetic 142 alterations (somatic or gene amplification of CDR2 or CDR2L antigen; HER2 143 overexpression ) may contribute to triggering the paraneoplastic immune response. In paraneoplastic syndromes with antibodies against intracellular proteins (e.g., CDR2 or CDR2L, Hu proteins, and others) the antigens released by apoptotic tumour cells are taken up and processed by antigen-presenting cells at the regional lymph nodes, 8 144, initiating the anti-tumour response. These immune responses are often subclinical, 145 and only a small proportion of patients develop an enhanced immune response that cross-reacts with the nervous system, resulting in the paraneoplastic syndrome. For example, between 16% and 26% of patients with SCLC develop anti-Hu immune responses, but only a very small proportion of these patients develop anti-Hu 144, 146, 147 paraneoplastic encephalomyelitis. Thus, in these patients an initial (and potentially beneficial) anti-tumour immune response subsequently becomes an 63, aggressive T cell cytotoxic immune attack against self-proteins expressed in neurons. 148 It is likely that similar tumour-related triggering mechanisms operate in some of the encephalitis mediated by antibodies against cell surface proteins (e.g., GABAbR and 10 94 SCLC, or AMPAR and lung or other cancers ). However, it is unknown if the neural proteins expressed by these tumours are mutated, as occurs in some of the classical paraneoplastic syndromes. All current evidence suggests that the mechanisms involved in triggering the immune response against cell surface proteins predominantly prime B cell activation and synthesis of pathogenic antibodies. These antibodies are able to alter the function, density, or structure of the neuronal antigens, causing potentially reversible 9 neurological deficits. In the case of ovarian teratomas, the tumour itself contains mature or immature neural tissue that expresses NMDAR and probably contributes to initiating the immune 61, 149 response (Figure 3.4A). Compared with teratomas of patients without anti-NMDAR encephalitis, those with encephalitis have more frequent neuroglial components and extensive inflammatory infiltrates with over-representation of B cells, plasma cells, and dendritic cells forming tertiary lymphoid structures (Figure 3.4C,D; see also Figure 16, 150 8.10). There is evidence that tumour-infiltrating B cells are able to synthesize 151 NMDAR antibodies in vitro. Figure 3.4 Neural tissue and inflammatory infiltrates in teratomas from patients with anti- NMDAR encephalitis. (A) and (B) show the presence of neural tissue in teratomas of two patients with anti- NMDAR encephalitis. The brown immunostaining (MAP2) demonstrates neurons and an extensive network of neural processes that express NMDARs (not shown). (C) and (D) correspond to an ovarian teratoma of a patient with anti-NMDAR encephalitis immunolabelled for the presence of CD3+ cells (C) and CD20+ cells (D). (E) and (F) correspond to an ovarian teratoma of a patient without anti-NMDAR encephalitis immunolabelled for CD3+ cells (E) and CD20+ cells (F). While CD3 T cells are present in both tumours, only the tumour of the patient with anti-NMDAR encephalitis is enriched by infiltrates of CD20 (B) cells. All scale bars = 20 µm. (C–F reproduced from reference 16 with permission) 3.4.1.2 Autoimmune Responses Triggered by Thymomas Thymomas are the only tumours able to generate mature T cells from immature 152 precursors. Because many of these tumours are enriched with autoantigen-specific T cells, a disturbance of circulating T cell subset composition by export of intratumoural cytotoxic and helper T cells likely contributes to systemic and neurologic autoimmunity, including paraneoplastic syndromes of the CNS, myasthenia gravis, and autoimmune 152–155 encephalitis (Table 3.1). Moreover, this predisposition of thymomas to associate with autoimmunity suggests a loss of self-tolerance produced by alterations in the mechanisms of positive and negative T cell selection that normally occur in the thymus, 156–158 resulting in autoreactive T cells that also affect B cell function. A molecular link between thymoma and autoimmunity was demonstrated in a patient whose tumour lacked expression of the autoimmune regulator gene AIRE, and who developed clinical manifestations similar to the autoimmune polyglandular syndrome type 1 (APS1), a 158 monogenic syndrome that results from alterations of the AIRE gene. This gene regulates the expression of tissue-specific self-antigens by medullary thymic epithelial cells, and its disruption in the tumour of the patient predisposes to autoimmunity. Upregulation of AIRE-related genes was found in a transcriptomic analysis of ovarian 159 cancers from patients with anti-Yo associated paraneoplastic cerebellar degeneration. The same study showed over-representation of CD8+ Treg cells in the paraneoplastic tumours compared with those of patients without anti-Yo cerebellar degeneration, although the overall degree of immune cell infiltration was similar in both groups of tumours. The mechanisms of how upregulation of AIRE-related genes could be involved in the anti-Yo immune response or the presence of tumour-infiltrating lymphocytes were 159 not investigated. There are no similar studies of AIRE in thymomas from patients with paraneoplastic syndromes or autoimmune encephalitis, but these patients usually develop multiple concurrent autoantibodies and overlapping syndromes supporting important alterations 155, 160, 161 in the mechanisms of immune self-tolerance. A typical clinical scenario in thymoma-related autoimmune encephalitis is illustrated by patients with a history of thymoma, often invasive or metastatic, and AChR antibodies with or without symptoms of myasthenia gravis, who after several years of follow-up develop clinical manifestations of autoimmune encephalitis. These patients often have concurrent GABAaR, LGI1, CASPR2, or AMPAR antibodies in addition to AChR or CRMP5 antibodies (see Clinical 155 Vignette 9.2 in Chapter 9). 3.4.1.3 Autoimmune Responses Triggered by Hodgkin Lymphoma A third type of tumour-related immunological activation against neural antigens is provided by Hodgkin lymphoma. In patients with Hodgkin lymphoma and CNS disorders associated with neuronal surface antigens, such as DNER (or Tr), mGluR1, or mGluR5, the 49, 71, 162 tumour usually does not express the corresponding antigens. Additionally, a link between systemic autoimmune disorders and some subtypes of Hodgkin lymphoma has 163 been reported. Thus, general dysfunction of the immunological system in some patients with Hodgkin lymphoma may predispose to neurological autoimmunity, although the exact mechanisms are unclear. In some types of autoimmune encephalitis, 164 such as anti-mGluR5 encephalitis (or Ophelia syndrome ), prompt treatment of the lymphoma often leads to a fast and remarkable neurological recovery, supporting a role 28 of the tumour in the immune response. 3.4.1.4 Brain Autoimmunity and Immune Checkpoint Inhibitors Normal activation of T cells occurs through the interaction of the T cell receptor with peptides presented by major histocompatibility complex (MHC) molecules expressed on the surface of dendritic and other antigen-presenting cells. This T cell activation is strongly regulated by several co-stimulatory and inhibitory mechanisms. Inhibition is accomplished by the binding of CTLA-4 (cytotoxic T-lymphocyte-associated protein 4) to 165 CD80 and CD86 molecules expressed by antigen-presenting cells. Another checkpoint inhibitor expressed in T cells is the programmed cell death 1 (or PD-1) molecule that binds to the PD1-ligand 1 (or PDL1) expressed by antigen-presenting cells and tumour 166 cells. Therefore, inhibition of these immune checkpoint molecules (CTLA-4, PD1, and PDL-1) by specific monoclonal antibodies (see Chapter 16) results in persistent and generalized activation of the immune system with clinically effective anti-tumour 167 responses. The enhanced activation of the immune system by ICIs sometimes results in immune- 168, 169 related complications that affect the nervous system (Box 3.3). Moreover, the growing usage of immune checkpoint inhibitors (ICIs) in the treatment of tumours frequently involved in paraneoplastic syndromes and autoimmune encephalitis (e.g., lung cancer, breast cancer, neuroendocrine tumours) has raised concern for a potential 170 increase in the frequency of anti-neuronal immune responses (Figure 3.5). This concern is supported by a mouse model that showed a link between ICIs and immune- mediated paraneoplastic neurological syndromes. In this model, mice expressing a neo- self-antigen (hemagglutinin) in Purkinje neurons and an implanted breast tumour received antigen-specific CD4 and CD8 T cells with or without a CTLA-4 inhibitor. Animals that did not receive the CTLA-4 inhibitor showed a partial anti-tumour response without neurological dysfunction, but mice that received the inhibitor developed prominent + neurological deficits caused by CD8 cytotoxic T cells specifically directed against the neo- 171 antigen expressed in the Purkinje cells. Box 3.3 Mechanisms of Brain Autoimmunity and Immune Checkpoint Inhibitors 1. 1. The ICIs contribute to triggering an immune response against neural proteins expressed by the tumour (classical mechanism proposed for paraneoplastic 168 syndromes ). 2. 2. The ICIs enhance a pre-existing (but subclinical) immune response against 168 onconeural proteins (e.g., patients harbouring onconeural antibodies before 172, 173 starting on ICIs ). 3. 3. Mechanisms #1 and #2 can coexist and are involved in most ICIs-triggered paraneoplastic neurologic syndromes, the majority with antibodies to intracellular 172, 174 antigens (e.g., Hu, Ma2). 4. 4. Mechanisms #1 and #2 are supported by an animal model of expression of a neo- 171 antigen by an implanted tumour and Purkinje cells of the cerebellum. 5. 5. The most frequent immune-related adverse effects of ICIs are not associated with autoantibodies (e.g., myositis, neuropathies) or affect the peripheral nervous system 170 (e.g., myasthenia gravis, Guillain–Barré syndrome). 6. 6. Autoimmune encephalitis mediated by antibodies against neural surface antigens 170 are among the less frequent ICI-induced neurological complications. Figure 3.5 Proposed mechanisms of autoimmune-related adverse effects of immune checkpoint inhibitors. The figure shows a multistep process resulting in several types of paraneoplastic or autoimmune disorders affecting the central and peripheral nervous system. It is postulated that intracellular or cell surface neuronal proteins expressed by the tumour 1 (inset 1) are released by apoptotic cells (inset 2). These proteins are processed by dendritic cells and presented at regional lymph nodes to the immunological system (inset 3). For reasons that are unclear, when the target antigens are neuronal cell surface proteins, the mechanisms of neurological disease are predominantly mediated by B cells/ 95 antibodies. Inset 3a summarizes the complex interaction of activated B lymphocytes and helper T cells in the lymph node leading to B cell proliferation and generation of antibody-secreting memory B cells and plasma cells. Preceding this B–T cell interaction, B cells have been activated by neuronal antigens released from tumour cells, and helper T cells have been activated by peptides processed by antigen-presenting cells (dendritic cells) in the context of MHC class II. There is evidence that antibody-secreting cells reach the CNS, where they undergo restimulation, antigen-driven affinity maturation, clonal expansion, and differentiation into plasma cells (not shown here; see Figure 3.3), with further production of antibodies. In the brain, these antibodies bind to the cell surface targets (e.g., GABAbR, AMPAR, or others), causing structural and functional alterations that, depending on the target, result in different forms of paraneoplastic encephalitis (inset 5). If the antigen is expressed in the peripheral nervous system, where there is no blood–brain barrier, the antibodies directly reach the target (e.g., AChR, VGCC), resulting in the neurological disorder (inset 6 represents antibodies against VGCC in LEMS). When the neuronal antigen released by apoptotic tumour cells is intracellular (onconeuronal antigen), the mechanisms of neurological disease are mediated by cytotoxic T cells. Inset 3b shows the activation of cytotoxic T cells by dendritic cells in the context of MHC class I. If these T cells reach the brain they cause irreversible neuronal loss (inset 4); these cytotoxic T cells may be accompanied by antibodies against the antigen, but these do not appear to play a major pathogenic role. The same cytotoxic T response has anti-tumour effects (inset 7), which likely explains the observation that tumours of patients with PNS 1 often show a more indolent course compared with those of patients without PNS. Whether antibodies generated by cell surface antigens (inset 3a) have clinically significant anti-tumour effects is unknown (not shown in the graph). Immune checkpoint inhibitors acting on CTLA-4 (insets 3a and 3b) or on PD-1 and PD-L1 molecules (inset 7) enhance anti-tumour immune responses that when directed against antigens expressed in the central or peripheral nervous system may lead to paraneoplastic syndromes or autoimmune encephalitis. (reproduced from reference 170 with permission) In the field of autoimmunity the experience gained from the neurological adverse effects of ICIs supports the longstanding theory (formulated many years before ICIs became clinically available) that a tumour-induced onconeural immune response could lead in 7, 175 some patients to important effects on the tumour and nervous system. An effective anti-tumour immune response provides an explanation for the observation that the tumours of patients with paraneoplastic syndromes appear to have a better outcome 144, 146, 176 than those of patients without paraneoplastic syndromes; however, the same immune response misdirected against the nervous system causes severe and often 177, 178 irreversible deficits that in some cases may lead to the death of the patient. Considering that for some types of cancers there are subsets of patients who have 144, subclinical immune responses against onconeural antigens (e.g., SCLC and anti-Hu; 146, 147 145 ovarian cancer and anti-Yo ), it has been suggested that the use of ICIs in these 170, 174 patients may favour the development of paraneoplastic neurologic syndromes. Future studies should address this question; however, the current experience indicates that the introduction of ICIs in clinical practice has been associated with an increasing number of patients with certain types of paraneoplastic encephalitis, such as anti-Ma2 172 encephalitis. Although this disorder typically associates with germ-cell tumours of the 179 testis, for which current therapies do not include ICIs, the increase of cases has been 172 among patients with non-small-cell lung cancer treated with ICIs. The most common immune-related neurologic diseases induced by ICIs in which neural antibodies have been identified are myasthenia gravis, Guillain–Barré syndrome, and the previously noted paraneoplastic encephalitis with antibodies against intracellular 170 antigens. Only a minority of encephalitis triggered by ICIs have been found associated 180, 181 182 with antibodies against surface antigens (NMDAR, CASPR2 ). In most other types of inflammatory or autoimmune encephalitis induced by ICIs, no neural antibodies 183–185 were identified (although many studies were not comprehensive). The clinical aspects, including risk factors and diagnostic and treatment approaches to autoimmune and paraneoplastic encephalitis triggered by ICIs are discussed in Chapter 16. 3.4.2 Herpes Simplex and Other Viral Encephalitis as Triggers of Autoimmune Encephalitis Bacterial and viral infections can lead to neural autoimmunity through mechanisms of molecular mimicry between the infectious agent and molecules expressed by the nervous system. Examples include antibodies against nerve glycolipids triggered by Campylobacter jejuni or Zika virus and the development of Guillain–Barré 186–188 syndrome, and autoimmune responses triggered by group A streptococcal 189 infections leading to Sydenham chorea. Prodromal infections are very common in patients with acute disseminated encephalomyelitis (ADEM), although a link between a specific infection or molecule expressed by the infectious agent and a brain target 190, 191 antigen has not been identified. A study that investigated serum and CSF samples of patients who had herpes simplex encephalitis (HSE) found that 4 (9%) of 44 patients harboured IgG NMDAR antibodies, 20 and 9 (20%) additional patients had IgA or IgM antibodies. The authors did not find clinical differences between patients with and without antibodies, although the clinical 20 information was retrospectively assessed. Nevertheless, these immunological findings led the authors to suggest that autoantibodies could be involved in some of the 192 neurological complications that occur after HSE. Subsequent single-case reports and small series of patients who developed relapsing neurological symptoms post-HSE, but were no longer PCR positive for herpes simplex virus-1 (HSV-1), showed that all harboured antibodies against one or more neural proteins or receptors such as NMDAR, 30, 193–197 and less frequently GABAa receptor, dopamine 2 receptor, or AMPAR. To determine the frequency of autoimmune encephalitis post-HSE and whether the viral 19 infection could trigger brain autoimmunity, Armangue et al. prospectively studied 54 patients with HSE. All patients were followed clinically and immunologically for at least one year since onset of viral encephalitis, and 14 (27%) developed autoimmune encephalitis after the viral infection resolved. At onset of the viral infection these patients did not have neural antibodies, but all were antibody-positive by the time they developed neurological symptoms due to autoimmune encephalitis. In most cases, the antibodies became detectable around three weeks after onset of the viral infection. In 9 (64%) of the 14 antibody-positive patients, the antibodies were against the NMDAR; for the other 5 cases the identity of the antigens was unknown. The authors found that all patients younger than four years developed choreoathetosis along with other symptoms, such as change of behaviour, seizures, or decreased level of consciousness. In contrast, patients older than four years predominantly developed change of behaviour and psychiatric symptoms. At one-year follow-up, children younger than four years were more likely to have a worse outcome and chronic epileptic seizures that those who were older (see 19 Chapter 8). In our experience, brain tissue immunohistochemistry with serum and CSF of patients with autoimmune encephalitis post-HSE show that many of them develop multiple 19 autoantibodies, although the targets are not all known. It has been suggested that antigens released by neurons undergoing viral destruction activate neuronal autoimmunity. This activation most likely occurs in the deep cervical lymph nodes which 198 receive soluble and cell-transported antigens from the CNS. The role of deep cervical lymph nodes as a site of processing and presentation of CNS-derived antigens to the immunological system is well known for T cell-mediated immune responses, but it is less 199 clear for antibody-mediated disorders. Naive B cells exposed to the processed antigens, in conjunction with CD4+ T cells, become antigen-conditioned B cells and differentiate into plasma cells. After entering the brain, memory B cells undergo restimulation, antigen-driven affinity maturation, clonal expansion, and differentiation into antibody-producing plasma cells. The contribution of systemically produced antibodies to the pool of antibodies present in the brain is unclear and may depend on 9 systemic antibody titres and the integrity of the blood–brain barrier (Figure 3.3). In our experience, studies of paired serum and CSF samples of patients who develop autoimmune encephalitis post-HSE show that NMDAR antibodies are first detected in the CSF. Moreover, close follow-up of CSF antibody synthesis and specificity suggests that there is first an extensive antibody synthesis against multiple antigens followed by a subsequent fine-tuning and enhancement of antibody titres against a restricted number of antigens, such as the NMDAR (Figure 3.6). Evidence that HSE can trigger NMDAR antibodies has been provided by a model of intranasal administration of HSV-1 to mice that resulted in the synthesis of NMDAR antibodies; these antibodies reduced the levels 200 of NMDAR in the hippocampus. Figure 3.6 Development of NMDAR antibodies post-HSE. (A–C) The process of NMDAR antibody development in a three-month-old boy with autoimmune encephalitis after HSE. (A) CSF obtained at onset of HSE does not immunoreact with the brain; there is no evidence of antibodies in the CBA with HEK293 cells expressing NMDAR. (B) diffuse brain immunostaining by CSF obtained on day 21; there is mild reactivity with NMDAR in the CBA. (C) a clearly defined pattern of NMDAR immunostaining with CSF obtained on day 25; there is intense reactivity with NMDAR in the CBA. (D) Reactivity of the CSF from a patient with classic (not HSE-related) anti- NMDAR encephalitis; there is intense reactivity with NMDAR in the CBA. Similar patterns of antibody development and immunostaining were seen for other patients who 19 developed NMDAR antibodies after HSE. Scale bar for frames showing hippocampus, 200 µm; scale bar for brain sections, 2 mm; Scale bar in the CBA, 10 µm. (reproduced from reference 19 with permission) The observation that many patients with autoimmune encephalitis post-HSE develop antibodies against several antigens supports the theory of antigen release by neurons undergoing destruction, rather than a mechanism of molecular mimicry between a protein sequence of the virus and a single neural protein or receptor (e.g., NMDAR). In the latter case, one would expect antibodies against one neural protein. However, a study found that patients who developed classical anti-NMDAR encephalitis (without previous history of HSE), were more likely to have antibodies against HSV-1 than age-matched controls with other neuroinflammatory disorders, suggesting a mechanism of molecular 201 mimicry. Alternatively, HSV-1 infection could potentially alter NMDAR expression, even 201 outside the CNS, in a way that would make the receptor more immunogenic. Regardless of the mechanism involved, the initial symptoms of most patients with autoimmune encephalitis post-HSE occur within the first three months after the viral encephalitis, resulting in a biphasic curve of symptoms, the first caused by the virus and 19 the second by the autoimmune response (Figure 3.7). In rare instances, this biphasic curve is not observed because patients develop autoimmune-related symptoms in 197 continuity with the infection-related symptoms. These patients can be particularly difficult to diagnose, and the autoimmune symptoms might be mistaken for residual 19 deficits of the viral encephalitis. Figure 3.7 Biphasic clinical course in patients who develop autoimmune encephalitis after herpes simplex encephalitis. The graph describes the most common clinical scenario and time course of symptoms in patients who develop autoimmune encephalitis (AE) after herpes simplex encephalitis (HSE). At presentation of HSE (day 0) patients do not harbour serum or CSF neuronal antibodies. Within the first three months (median ~25 days) after the presentation of HSE, and after the viral infection has resolved, some patients develop a second wave of neurological symptoms that correspond to AE. In a prospective study of 51 patients with 19 HSE, 14 (27%) developed AE. In 64% of these AE patients neuronal antibodies could be detected in CSF (and less frequently serum) on day 21 when acyclovir was discontinued and preceding the development of AE. In many of these patients the antibodies were directed against NMDAR, but some also had concurrent antibodies against other known or unknown neuronal antigens. At one-year follow-up, ~50% of patients with AE post-HSE still had detectable neuronal antibodies. In general, the outcome of AE post-HSE was not 19 as good as the outcome of classical anti-NMDAR encephalitis unrelated to HSE. AE has 202–205 also been reported after Japanese B encephalitis, showing a biphasic clinical course resembling the one depicted here. A few case reports have suggested that CNS infections by other types of herpesvirus such 197 as Epstein–Barr virus (EBV) meningo-encephalitis, or varicella zoster virus (VZV) 206 myelitis may also initiate anti-neural immune responses, including NMDAR 207 encephalitis. Besides CNS infections by herpesvirus, a case of tick-borne encephalitis 208 and another case of human immunodeficiency virus (HIV) encephalitis have been reported as preceding anti-NMDAR encephalitis. A separate scenario is the identification of a positive viral PCR (e.g., EBV or human herpesvirus 6 (HHV-6)) concurrent with the autoimmune encephalitis. Although these cases may be described as ‘parainfectious’ autoimmune encephalitis, the patients do not show evidence of infection or immunodepression favouring an infection, and the clinical features are otherwise typical of autoimmune encephalitis (e.g., anti-NMDAR or 197 GABAbR). It has been postulated that CSF viral positivity may be detected in some patients because of shedding of latent neurotropic viral DNA caused by the autoimmune 209 encephalitis. Alternatively, viral reactivation can potentially occur. Moreover, CSF PCR positivity for EBV, HHV-6, or HSV-1, but without evidence of CNS viral infection, has been described in immunosuppressed patients and those with stroke, neurodegenerative, or 210, 211 neuroinflammatory diseases. In this setting, EBV DNA is one of the most frequently detected; the fact that EBV is carried by white blood cells may explain why in some studies this was found only among patients with traumatic or well-established 211 inflammatory CNS diseases (e.g., bacterial infection, meningitis). Besides HSE, Japanese B encephalitis is the other viral encephalitis that unambiguously 137, 202–205 associates with autoimmune encephalitis post-viral encephalitis. Japanese B encephalitis is caused by a mosquito-borne flavivirus, and is one of the most common 212 causes of viral encephalitis in Asia, with potentially devastating residual deficits. In most patients the disease is monophasic and presents with fever, headache, seizures, decreased level of consciousness, and brain MRI abnormalities bilaterally involving the thalamus or basal ganglia. Although the majority of patients only have a monophasic (viral) disease, a small number of cases develop ‘a second phase’ that manifests with 213 mutism, dystonia, orofacial dyskinesia, and behavioural changes. The similarity of these symptoms with those of autoimmune encephalitis post-HSE led us to speculate 193 that antibody-mediated mechanisms were likely involved. In subsequent studies, Ma 137, 202 et al. demonstrated that the second phase of the disease was characterized by the presence of IgG NMDAR antibodies in CSF (less frequently in serum), although some patients did not have these antibodies (the presence of other antibodies was not investigated). In a study of 65 patients with Japanese B encephalitis (63 of them with follow-up), 5 (7.9%) patients developed symptoms of autoimmune encephalitis during the convalescence phase of the viral encephalitis. Three of the patients were NMDAR antibody-positive and two were NMDAR antibody-negative (other neural antibodies were not investigated). The four younger patients (age range 24 months to 11 years) developed choreoathetosis with irritability, agitation, or sleep dysfunction, whereas the older child (13 years) developed psychosis, aggressive behaviour, confusion, and a sleep 202 disorder. Thus, compared with the autoimmune encephalitis post-HSE, the number of reported cases of post-Japanese encephalitis is smaller, but the timing of the development of the autoimmune complication and the type of neurological symptoms are remarkably similar in both disorders. Among non-viral infections preceding (or potentially linked to) anti-NMDAR encephalitis, the following infectious agents have been reported: Angiostrongylus cantonensis 214 215 causing eosinophilic meningitis; leptospira interrogans; treponema pallidum 216 217 218 (syphilis), mycoplasma pneumonia, and tuberculous meningitis. In the latter case, the diagnosis of anti-NMDAR encephalitis was based on an ELISA (not CBA or brain immunostaining), and therefore the diagnosis should be considered unclear. Overall, except for HSE and Japanese B encephalitis, which definitively can trigger autoimmune encephalitis (often with NMDAR antibodies), the potential role of other infectious agents as triggers of autoimmune encephalitis is unclear due to the small number of cases identified. 3.4.3 HLA The human leukocyte antigen (HLA) system, which corresponds to the MHC in humans, plays a critical role in the antigen presentation of intracellular and extracellular peptides and the regulation of innate and adaptive immune responses. Moreover, the ability of 219 220 HLA to influence thymic selection and peripheral anergy of T and B cells underscores its role in the pathogenesis of immune-mediated disorders. The strong association of some diseases with specific HLA alleles can have diagnostic value, sometimes with additional prognostic and treatment implications. These concepts are well illustrated with several rheumatological diseases, such as spondyloarthritis, which is linked to MHC class I (HLA-B27), or coeliac disease, linked to MHC class II (HLA- 221 DQ2/DQ8). In the case of spondyloarthritis, HLA-B27 status is part of the Assessment for Spondyloarthritis International Society classification criteria for axial and peripheral 222 spondyloarthritis. In addition to being an important part of the diagnostic work-up of ankylosing spondylitis, HLA-B27 positivity has prognostic value: it associates with earlier disease onset, higher disease activity, or symmetric sacroiliitis, among other features (reviewed in reference 221). TNF-alpha inhibitors have greater therapeutic effect in HLA- 223 B27-positive patients. 224 Coeliac disease has a strong association with HLA-DQ2 and DQ8. In this disease, zygosity is a strong determinant of gluten peptide presentation and disease risk; for 225, 226 example, homozygous patients have a more severe disease course. While a positive HLA test merely indicates genetic susceptibility, the negative predictive value is 227 so strong (~99%) that a negative test virtually excludes coeliac disease. In narcolepsy-cataplexy type 1 (NT1), the association with HLA class II DQB1*06:02 is very robust, so that almost all patients carry this allele, but it is not included as part of the diagnostic criteria (reviewed in reference 228). This and several other class II risk/ protective allele associations provide strong support to the autoimmune hypothesis of sporadic NT1. A similar autoimmune background for risk/protective HLA alleles has been identified in patients with NT1 post-H1N1 (mainly H1N1 vaccinated). A study on NT1 post- H1N1 (84% H1N1 vaccinated with Pandemrix) identified C*02:02 as potentially protective 229 against sleep paralysis and hypnagogic hallucinations. In autoimmune encephalitis, the potential association with specific HLA alleles has been 22, 23 35–37 37, 41 46, examined in patients with anti-NMDAR, LGI1, CASPR2, and anti-IgLON5 47, 129 encephalitis. Robust HLA associations occur in anti-LGI1, CASPR2, and IgLON5 disorders, but not in anti-NMDAR (Table 3.1). In contrast to spondyloarthritis, coeliac disease, and NT1, no clinical implications for diagnosis, disease severity, treatment, or prognosis have been identified in patients with anti-NMDAR, LGI1, or CASPR2 encephalitis carrying the indicated HLA alleles. Attempts to find a genetic background to explain the frequent adverse effects of steroid and common drug rashes (mainly due to anti-seizure medications) in patients with anti-LGI1 encephalitis did not clearly identify distinct HLA 37 associations. In a study that included 25 patients with anti-LGI1 encephalitis without tumour, and 4 with tumour, the group with tumour was less likely to show the robust HLA associations 35 (DRB4 and DR7) observed in the non-tumour group. This suggested two separate immunopathogenic routes leading to the same disease, as described previously in 230 LEMS. However, another study that included 59 patients without tumour and 9 with 37 tumour did not find differences in HLA association (see also Chapter 7). The only CNS disorder with antibodies against neuronal surface antigens in which the 129 presence of a specific HLA allele had clinical implications is IgLON5. In a study that included 20 patients with HLA DRB1*10:01 and 15 patients with HLA DRB1*non-10:01, those that were HLA DRB1*10:01-positive were more likely to have sleep or bulbar symptoms, parasomnia, sleep breathing disorder, or dysautonomia, and less likely to 129 have cognitive impairment (see Chapter 15). In patients with CASPR2 antibodies, the association with DRB1*11:01 only occurs in cases with predominant limbic encephalitis; other phenotypes such as peripheral nervous system hyperexcitability (or 41 neuromyotonia) and Morvan syndrome do not associate with this haplotype. 3.5 Functional Interaction of Antibodies with Neuronal Cell Surface and Synaptic Proteins 3.5.1 General Concepts: In-Vitro and In-Vivo Models of Functional Assessment of Antibodies After the initial descriptions of anti-NMDAR encephalitis and preliminary clinical and pathological findings suggesting that the antibodies could be pathogenic, the first steps to experimentally confirm their pathogenicity consisted in examining whether patients’ antibodies caused alterations in cultured neurons. For this, the hippocampi of rat embryos were dissected and the dissociated neurons were cultured and exposed to 64, 65 patients’ antibodies. These primary cultures of rat hippocampal neurons are able to establish functional synapses and have proven to be extraordinarily helpful in determining the functional and structural synaptic alterations mediated by antibodies of 231 patients with most autoimmune encephalitis. Experiments are usually performed when the cultures are ~2–3 weeks old, and consist in adding for a few hours or days patients’ CSF or IgG isolated from serum. The same experimental model can be used to 138, 232 assess monoclonal antibodies derived from B cells or plasma cells from patients. Studies may include: determination of antibody binding to extracellular epitopes of neuronal receptors or cell surface proteins; quantification of the levels of the corresponding receptors or protein antigens at synaptic and extrasynaptic sites (to assess whether antibodies cause internalization of the antigen); visualization of the internalized antigen–antibody complexes, and electrophysiological assessment of the effects of antibodies on synaptic function and excitability using whole-cell patch-clamp 64, 69, 233–236 studies. By contrast, in-vitro models based on human embryonic kidney 293 (HEK293) cells transfected with a specific receptor or protein of interest have limitations. Transfected cells are useful for the electrophysiological assessment of antibody effects on specific receptors, but the artificial expression of receptors or proteins outside the synapse or without their normal interacting partners prevents a full assessment of antibody pathogenicity (e.g., disruption of normally interacting synaptic proteins not present in HEK cells, or internalization of receptors). An example of this limitation is exemplified by studies examining the effects of GlyR antibodies on HEK293 cells expressing alpha 1 homodimeric glycine receptors that initially suggested antibody- 31 mediated internalization of receptors as the main pathogenic mechanism. However, investigations using cultured neurons exposed to antibodies or Fab fragments (a fragment containing a single arm of the antibody) demonstrated a fast and substantial reduction of glycinergic currents, suggesting that this was the main pathogenic 66 mechanism. In our experience, the demonstration of antigen-specific antibody effects in cultured neurons predicts similar alterations in animal models of passive transfer of antibodies, usually accompanied by impairment of memory and behaviour. Fulfilment of these criteria in an animal model confirms that the antibodies have pathogenic effects and that the disease is antibody-mediated (Box 3.4). * Box 3.4 Defining Criteria for Antibody Pathogenicity in Autoimmune Encephalitis 1. 1. Demonstration that injection or infusion of patients’ antibodies (but not control ** IgG) cause neurological symptoms in animals. 2. 2. Demonstration that the infused antibodies specifically react with the target neural antigen. 3. 3. Demonstration that the infused antibodies alter the structure or function of the target antigen leading to synaptic or neural dysfunction. 4. 4. Demonstration that the induced neurological symptoms correlate with the structural/functional effects of the antibodies (e.g., reversibility of symptoms after the antibody infusion is discontinued). 237 *Adapted from Witebsky’s postulates as modified by Rose and Bona. **Alternatively, systemic injection of antibodies to pregnant rodents during the period in which there is placental transfer of patients’ antibodies and the blood–brain barrier is immature (i.e., permeable to IgG) can also demonstrate behavioural alterations in the pups, along with antibody-specific effects on the target neuronal antigen, and potential 238 neurodevelopment alterations. The most frequent animal model approach used to assess whether antibodies from patients with autoimmune encephalitis cause synaptic alterations and symptoms consists in the infusion of the antibodies into the cerebroventricular system of mice. In this passive transfer model, unilateral or bilateral ventricular catheters are placed in the lateral ventricles of the animal and then connected to subcutaneous osmotic pumps. The pumps are filled with CSF, serum IgG, or human-derived monoclonal antibodies that for 68, 138 approximately 14 days are continuously infused into the ventricular system. Animals can be tested for memory impairment, behavioural changes, or evidence of seizures during the infusion period, and they can also be assessed for reversibility of any 68, 69 alterations once the infusion has stopped. Subsets of animals can be studied at different time points to determine the presence of antibodies in brain and to identify antibody-mediated molecular alterations (e.g., internalization of the target protein or receptor accompanied or not by effects on proteins that normally interact with the target), antibody-mediated functional alterations (e.g., neuronal excitability, hippocampal 76, 239 plasticity), or whether experimental manipulations (e.g., novel therapeutics) can 77 modify the antibody effects. Another animal model approach to study the effects of autoantibodies uses active 74, 75 immunization with cell surface or synaptic proteins. These models have the advantage of combining the presence of autoantibodies generated by the animals along with an inflammatory component, which does not occur in the passive transfer model. Both models, however, have important caveats that need to be taken in consideration (Box 3.5). Box 3.5 General Considerations for Animal Models of Antibody-Mediated Encephalitis Administration of patients’ CSF or serum IgG antibodies via chronic infusion or single injection into the cerebroventricular system of rodents provides a good model to assess whether antibodies affect memory and behaviour. Spinal intrathecal antibody infusion is useful to assess antibody effects on spinal cord function. Caveats: to assess effects on memory and behaviour, cerebroventricular infusion of antibodies is better than systemic administration, which requires opening the blood–brain barrier with, for example, lipopolysaccharide (LPS), which by itself alters 240, 241 behaviour and enhances susceptibility to seizures. Human-derived monoclonal antibodies provide a highly specific approach to assess the effects of antibodies against a single epitope, but have potential limitations in animal models. Caveat: for diseases in which epitopes are distributed in different protein domains of the 70, 242 39 antigen (e.g., LGI1, CASPR2 ) monoclonal antibodies may provide limited or partial 243 information about the overall antibody-mediated effects. Monoclonal antibodies should match the IgG subclass of the disease-associated antibodies. Despite the advantages offered by the model of cerebroventricular transfer of antibodies, it has also limitations: 1. There is a predominant regional distribution of antibodies around the ventricular system (e.g., hippocampus). This distribution probably favours the assessment of hippocampal-dependent memory and behavioural alterations, but has limitations in assessing more global behavioural alterations. 2. The passive transfer approach does not associate with the T cell responses and 68 inflammatory alterations that occur in the disease. In active immunization models, the animals synthesize the autoantibodies which are 74, 75, 244 accompanied by T cell and other brain inflammatory mechanisms. However, several caveats should be considered: Caveats: antibodies raised by active immunization are often directed against epitopes 74 different from those targeted by human antibodies. These animals may develop symptoms caused by the antibodies or accompanying T cell-mediated mechanisms, which do not necessarily model those occurring in patients. A summary of the in-vitro and in-vivo models assessing the effects of patients’ antibodies is shown in Tables 3.3 and 3.4 For each disorder, the structure and main function of the target antigens and the effect of the functional interaction with the antibodies are described below in more detail. Table 3.3 Target antigens, epitope regions, and antibody effects in vitro (cultured neurons) Structural Antibody effects a Antigen properties Epitope targets on neurons Antibodies against cell surface receptors Alter surface dynamics and crosslink and internalize 64, 67, 234, Ionotropic receptor. NMDAR. Heterotetramer, Disrupt the composed of 2 interaction of GluN1 subunits 235, 247 NMDAR with ephrin NMDAR 76 (obligatory GluN1 B2 and dopamine subunits), and 2 239 receptors. GluN2 (A, B, C or D), 245, 246 Decreased synaptic or GluN3. NMDAR-mediated currents in whole- cell patch-clamp recordings of 64 miniature EPSCs. Induce receptor internalization and a reduction of Ionotropic receptor. synaptic Most are GluA2-containing heterotetramers GluA2; in some AMPAR followed by AMPAR composed on GluA1, patients GluA194 compensatory 2, 3, or 4 ryanodine receptor- 248 249 subunits. dependent incorporation of synaptic non-GluA2 69, 65 AMPAR. Ionotropic receptor. Hetero- or Induce homotetramer, 252 internalization of GluK2R GluK2 resulting from the 252 combination of GluK2Rs. GluK1, 2, 3, 4, or Structural Antibody effects a Antigen properties Epitope targets on neurons 250, 251 5. Ionotropic receptor. Heteropentamer Main targets: α1, Induce resulting from GABAaR and β3. Minor target internalization of combination of 19 119, 25, 255 119, 25 different γ subunit GABAaR. 253, 254 subunits. Do not induce receptor internalization. Metabotropic Preliminary data receptor. suggest the Heterodimer antibodies directly composed of 2 160 GABAbR GABAB1 block the function of subunits (GABA-B1 the rece