Basic of Electricity Learning Content PDF
Document Details
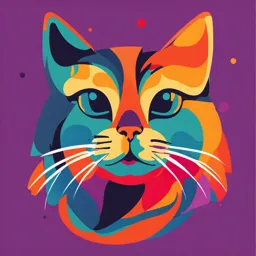
Uploaded by AudibleSine
Tags
Related
- Basic Electrical Engineering Module 1-1 PDF
- A/C Electrical System 2 Reviewer PDF
- EContent 11 2024 Electrical Engineering DC Circuits PDF
- Engineering Module - Semester I (Electronic & Electrical Engineering) PDF
- Electrical Engineering - Topics and Concepts
- Electricity - Electrical Principles and Technologies (Topics 1-3) PDF
Summary
This document provides a basic introduction to electricity focusing on DC and AC circuits, series parallel circuits, and power triangles. It also discusses common electrical safety hazards in the workplace. The content is geared toward electrical technicians and those preparing for electrical work.
Full Transcript
Control page ASSIGNMENT DISCIPLINE JOB / AREA \# DESCRIPTION ------------ -- ----------------------- --------------- --------------------------------------- Electrical Technician Area 2 Plant Electrical Installation Level-1 +-----------+--------...
Control page ASSIGNMENT DISCIPLINE JOB / AREA \# DESCRIPTION ------------ -- ----------------------- --------------- --------------------------------------- Electrical Technician Area 2 Plant Electrical Installation Level-1 +-----------+-----------+-----------+-----------+-----------+-----------+ | DOCUMENT | | REFERENCE | | | | | CONTROLS | | \# | | | | +===========+===========+===========+===========+===========+===========+ | | | JAD-LC-A2 | | | | | | | -YP-EMT-L | | | | | | | M | | | | +-----------+-----------+-----------+-----------+-----------+-----------+ | | | MODULE \# | REVISION | ISSUE | NEXT | | | | | \# | DATE: | REVIEW | | | | | | | DATE: | +-----------+-----------+-----------+-----------+-----------+-----------+ | | | 2024 | 2024.01 | 6 Nov | 01 Oct | | | | | | 2024 | 2027 | +-----------+-----------+-----------+-----------+-----------+-----------+ | | | DEVELOPED | REVIEWED | APPROVED | | | | | BY: | BY | BY: | | | | | | | | | | | | (SUBJECT | (SUBJECT | (AREA | | | | | MATTER | MATTER | OWNERS/LE | | | | | EXPERTS): | EXPERTS): | ADERS): | | +-----------+-----------+-----------+-----------+-----------+-----------+ | | | Marwan | Ali | XXXXXXXXX | | | | | Owaidhah | Fallatah | XXXXXXX | | | | | (12943) | (12610) | | | | | | Electrica | Electrica | | | | | | l | l | | | | | | Trainer | Specialis | | | | | | | t | | | | | | | | | | | | | | Bandar | | | | | | | Al-Mesawi | | | | | | | (13107) | | | | | | | Electrica | | | | | | | l | | | | | | | Specialis | | | | | | | t | | | | | | | | | | | | | | Ahmed | | | | | | | Haresi | | | | | | | (33423) | | | | | | | Electrica | | | | | | | l | | | | | | | Specialis | | | | | | | t | | | +-----------+-----------+-----------+-----------+-----------+-----------+  BASIC OF ELECTRICITY LEARNING CONTENT SN SUBJECT PAGE NUMBER ---- -------------------------------------- ------------- 1 Table of Contents 5 2 Learning Objectives 6 3 Basic Introduction of Electricity 8 4 DC and Magnetism 16 5 Understanding of AC Circuits 20 6 Series Parallel Circuits 42 7 Understanding Of Electrical Formulas 48 8 Understanding of Power Triangle 54 OBJECTIVES SN OBJECTIVE ---- --------------------------------------------------------------- 1 Basic understanding of DC and Magnetism 2 Basic understanding of AC Circuits & Series Parallel Circuits 3 Understanding of Power Triangle COMMON ELECTRICAL HAZARDS It's Your Life -- Protect it! It's not a secret -- electricity can be dangerous and when things go wrong lives can be at stake. According to the Statistics, 289 employees were killed by contact with electric current. This equates to more than one work related death every workday in electrical sites. Between 1992 and 2001 an average of 4,309 employees lost time away from work because of electrical injuries. Even if an injured employee doesn't die as a result of their exposure to electricity, the recuperation period can be long, painful and expensive. The goal is to keep employee health and maintain a quality of life that we all deserve! Current through the body, even at levels as low as 3 milliamperes, can also cause injuries of an indirect or secondary nature in which involuntary muscular reaction from the electric shock can cause bruises, bone fractures and even death resulting from collisions or falls (i.e. fall from a ladder after receiving a small shock). Workplace safety Preventing workplace hazards Good electrical safety habits can help protect you and your co-workers from injury. And you can play a role in recognizing and preventing workplace hazards. Keep in mind that: - - - Stay alert! Many electrical injuries could be prevented if people were alert to hazards. Stay aware by keeping focused on your job and don\'t let emotions like anger and frustration get in the way. BASIC INTRODUCTION OF ELECTRICITY Elements of an atom: All matter is composed of molecules which are made up of a combination of atoms. Atoms have a nucleus with electrons orbiting around it. The nucleus is composed of protons and neutrons. Most atoms have an equal number of electrons and protons. Electrons have a negative charge (-). Protons have a positive charge (+). Neutrons are neutral. The negative charge of the electrons is balanced by the positive charge of the protons. Electrons are bound in their orbit by the attraction of the protons. These are referred to as bound electrons. Free Electrons Electrons in the outer band can become free of their orbit by the application of some external force such as movement through a magnetic field, friction, or chemical action. These are referred to as [free electrons]. A free electron leaves a void which can be filled by an electron forced out of orbit from another atom. As free electrons move from one atom to the next an electron flow is produced. This is the basis of electricity. Conductors An electric current is produced when free electrons move from one atom to the next. Materials that permit many electrons to move freely are called conductors. Copper, gold, silver, and aluminum are examples of materials that are good conductors. Copper is widely used as a conductor because it is one of the best conductors and is relatively inexpensive. Insulators Materials that allow few free electrons are called insulators. Materials such as plastic, rubber, glass, mica, and ceramic are good insulators. An electrical cable is one example of how conductors and insulators are used together. Electrons flow along a copper conductor to provide energy to an electric device such as a radio, lamp, or motor. An insulator around the outside of the copper conductor is provided to keep electrons in the conductor. Semiconductors Semiconductor materials, such as silicon, can be used to manufacture devices that have characteristics of both conductors and insulators. Many semiconductor devices act like a conductor when an external force is applied in one direction and like an insulator when an external force is applied in the opposite direction. This principle is basic to the operation of transistors, diodes, and other solid-state electronic devices. Electric Charges Elements are defined by the number of electrons in orbit around the nucleus of an atom and by the number of protons in the nucleus. A hydrogen atom, for example, has only one electron and one proton. An aluminum atom has 13 electrons and 13 protons. An atom with an equal number of electrons and protons is said to be electrically neutral. Electrons in the outer band of an atom are easily displaced by the application of some external force. Electrons which are forced out of their orbits can result in a lack of electrons where they leave and an excess of electrons where they come to rest. A material with more protons than electrons has a net positive charge and a material with more electrons than protons has a net negative charge. A positive or negative charge is caused by an absence or excess of electrons, because the number of protons in an atom normally remains constant. Attraction and repulsion of electric charges The old saying, \"opposites attract,\" is true when dealing with electric charges. Charged bodies have an invisible electric field around them. When two like-charged bodies are brought together, their electric fields repel one body from the other. When two unlike-charged bodies are brought together, their electric fields attract one body to the other. The electric field around a charged body forms invisible lines of force. These invisible lines of force cause the attraction or repulsion. During the 18th century a French scientist, Charles A. Coulomb, studied fields of force that surround charged bodies. Coulomb discovered that charged bodies attract or repel each other with a force that is directly proportional to the product of the charges and inversely proportional to the square of the distance between them. Today we call this Coulomb\'s Law of Charges. Simply put, the force of attraction or repulsion depends on the strength of the charges and the distance between them. Current Electricity is the flow of electrons in a conductor from one atom to the next atom in the same general direction. This flow of electrons is referred to as current and is designated by the symbol \"I\". Current is measured in amperes, which is often shortened to \"amps\". The letter \"A\" is the symbol for amps. Current that constantly flows in the same direction is called direct current (DC). Current that periodically changes direction is called alternating current (AC). Direction of current flow Conventional current flow theory ignores the flow of electrons and states that current flows from positive to negative. To avoid confusion, we will use the electron flow concept which states that electrons flow from negative to positive. Voltage The force required to make electricity flow through a conductor is called a difference in potential, electromotive force (emf), or voltage. Voltage is designated by the letter \"E\" or the letter \"V.\" The unit of measurement for voltage is volts which is also designated by the letter \"V.\" A voltage can be generated in various ways. A battery uses an electrochemical process. A car\'s alternator and a power plant generator utilize a magnetic induction process. All voltage sources share the characteristic of an excess of electrons at one terminal and a shortage at the other terminal. This results in a difference of potential between the two terminals. For a DC voltage source, the polarity of the terminals does not change, so the resulting current constantly flows in the same direction. The terminals of an AC voltage source periodically change polarity, causing the current flow direction to change with each switch in polarity. Resistance A third factor that plays a role in an electrical circuit is resistance. Resistance is the property of a circuit, component, or material that opposes current flow. All material impedes the flow of electrical current to some extent. The amount of resistance depends upon composition, length, cross-section and temperature of the resistive material. For any specific material at a constant temperature, the resistance of a conductor increases with an increase of length or a decrease of cross-section. Resistance is designated by the symbol \"R.\" The unit of measurement for resistance is ohms, symbolized by the Greek letter omega. While all circuit components have resistance, a resistor is a component manufactured to provide a designated resistance that is often shown in color coded bands around the resistor. Ohms Law A simple electric circuit consists of a voltage source, some type of load, and a conductor to allow electrons to flow between the voltage source and the load. Ohm\'s law defines the relationship between current, voltage, and resistance and shows that current varies directly with voltage and inversely with resistance. Ohm\'s law defines the relationship between current, voltage, and resistance and shows that current varies directly with voltage and inversely with resistance. Ohms low triangle Ohm\'s law can be expressed in three ways. There is an easy way to remember which form of Ohm\'s law to use. By arranging current, voltage and resistance in a triangle, as shown in the accompanying illustration, you can quickly determine the correct formula. Ohms law example The accompanying illustration shows that if any two values are known, the third value can be easily calculated using the appropriate Ohm\'s law formula. BASICS OF ELECTRICITY (DC AND MAGNETISM) Permanent magnets: The principles of magnetism are an integral part of electricity. In fact, magnetism can be used to produce electric current and vice versa. When we think of a permanent magnet, we often envision a horse-shoe or bar magnet or a compass needle, but permanent magnets come in many shapes. However, all magnets have two characteristics. They attract iron and, if free to move (like the compass needle), a magnet will assume a north-south orientation. Magnetic lines of force: Every magnet has two poles, one north pole and one south pole. Invisible magnetic lines of flux leave the north pole and enter the south pole. Although the lines of flux are invisible, the effects of magnetic fields can be made visible. When a sheet of paper is placed on a magnet and iron filings loosely scattered over it, the filings arrange themselves along the invisible lines of flux. The density of these lines of flux is greatest inside the magnet and where the lines of flux enter and leave the magnet. The greater the density of the lines of flux, the stronger the magnetic field. Interaction between magnets: When two magnets brought together, the magnetic field around the magnets causes some form of interaction. When two unlike poles are brought together, the magnets attract each other. When two like poles are brought together, the magnets repel each other. "Like poles repel and unlike poles attract." Electromagnetism: An electromagnetic field is a magnetic field generated by current flow in a conductor. Every electric current generates a magnetic field and a relationship exists between the direction of current flow and the direction of the magnetic field. The left-hand rule for conductors demonstrates this relationship. If a current-carrying conductor is grasped with the left hand with the thumb pointing in the direction of electron flow, the fingers point in the direction of the magnetic lines of flux. Electromagnets: A coil of wire carrying a current, acts like a magnet. Individual loops of wire act as small magnets. The individual fields add together to form one magnet. The strength of the field can be increased by adding more turns to the coil, increasing the amount of current, or winding the coil around a material such as iron that conducts magnetic flux more easily than air. The left-hand rule for coils states that if the fingers of the left hand are wrapped around the coil in the direction of electron flow. The thumb points to the north pole of the electromagnet. An electromagnet is usually wound around a core of soft iron or some other material that easily conducts magnetic lines of force. A large variety of electrical devices such as motors, circuit breakers, contactors, relays and motor starters use electromagnetic principles. UNDERSTANDING OF AC CIRCUITS AC CURRENT Alternating current: The supply of current for electrical devices may come from a direct current (DC) source or an alternating current (AC) source. In a direct current circuit, electrons flow continuously in one direction from the source of power through a conductor to a load and back to the source of power. Voltage polarity for a direct current source remains constant. DC power sources include batteries and DC generators. By contrast, an AC generator makes electrons flow first in one direction then in another. In fact, an AC generator reverses its terminal polarities many times a second, causing current to change direction with each reversal. Alternating voltage and current vary continuously. The graphic representation for AC is a sine wave. A sine wave can represent current or voltage. There are two axes. The vertical axis represents the direction and magnitude of current or voltage. The horizontal axis represents time. When the waveform is above the time axis, current is flowing in one direction. This is referred to as the positive direction. When the waveform is below the time axis, current is flowing in the opposite direction. This is referred to as the negative direction. A sine wave moves through a complete rotation of 360 degrees, which is referred to as one cycle. Alternating current goes through many of these cycles each second. Basic AC generator: A basic generator consists of a magnetic field, an armature, slip rings, and brushes which connect to the load. In a commercial generator, the magnetic field is created by an electromagnet, but, for this simple generator, permanent magnets are used. An armature is any number of conductive wires wound in loops which rotates through the magnetic field. For simplicity, one loop is shown. When a conductor is moved through a magnetic field, a voltage is induced in the conductor. As the armature rotates through the magnetic field, a voltage is generated in the armature, which causes current to flow. Slip rings are attached to the armature and rotate with it. Carbon brushes ride against the slip rings to conduct current from the armature to the load, which in this case is a resistor and an indicator light. If the movement of the AC generator is tracked through a complete rotation of 360 degrees, during the first quarter of a revolution, voltage increases until it reaches a maximum positive value at 90 degrees. Voltage decreases during the next quarter cycle to zero at 180 degrees. During the third quarter cycle, voltage increases in a negative direction and reaches a peak at 270 degrees. During the last quarter cycle, voltage returns to zero. This is one complete revolution. This process continues as long as the generator is in operation. Frequency: The number of cycles per second of voltage induced in the armature is the frequency of the generator. If the armature rotates at a speed of 60 revolutions per second, the generated voltage will be 60 cycles per second. The recognized unit for frequency is hertz, abbreviated \"Hz.\" 1 Hz is equal to 1 cycle per second. Power companies generate and distribute electricity at very low frequencies. The standard power line frequency in the United States, Saudi Arabia and many other countries is 60 Hz. 50 Hz is also a common power line frequency used throughout the world. The following illustration shows 15 cycles in 1/4 second which is equivalent to 60 Hz. Four pole AC Generator: The frequency is the same as the number of rotations per second if the magnetic field is produced by only two poles. An increase in the number of poles causes an increase in the number of cycles completed in a revolution. A two-pole generator completes one cycle per revolution and a four-pole generator completes two cycles per revolution. In other words, an AC generator produces one cycle per revolution for each pair of poles. Amplitude: As previously discussed, voltage and current in an AC circuit rise and fall over time in a pattern referred to as a sine wave. In addition to frequency, which is the rate of variation, an AC sine wave also has amplitude, which is the range of variation. Amplitude can be specified in three ways: peak value, peak-to-peak value, and effective value. The peak value of a sine wave is the maximum value for each half of the sine wave. The peak-to-peak value is the range from the positive peak to the negative peak. This is twice the peak value. The effective value of AC is defined in terms of an equivalent heating effect when compared to DC. Instruments designed to measure AC voltage and current usually display the effective value. The effective value of an AC voltage or current is approximately equal to 0.707 times the peak value. The effective value is also referred to as the RMS value. This name is derived from the root-mean-square mathematical process used to determine the effective value of a waveform. Instantaneous value: The instantaneous value is the value at any one point on the sine wave. The voltage waveform produced as the armature of a basic two-pole AC generator rotates through 360 degrees is called a sine wave because the instantaneous voltage or current is related to the sine trigonometric function. As shown in the accompanying illustration, the instantaneous voltage (e) and current (i) at any point on the sine wave are equal to the peak value times the sin of the angle. The sine values shown in the illustration are obtained from trigonometric tables. Keep in mind that each point has an instantaneous value, but this illustration only shows the sine of the angle at 30-degree intervals. Inductance and inductor: The circuits studied to this point have been resistive. Resistance and voltage are not the only circuit properties that affect current flow, however. Inductance is the property of an electric circuit that opposes any change in electric current. Resistance opposes current flow, inductance opposes changes in current flow. Inductance is designated by the letter \"L\". The unit of measurement for inductance is the henry (h). Current flow produces a magnetic field in a conductor. The amount of current determines the strength of the magnetic field. As current flow increases, field strength increases, and as current flow decreases, field strength decreases. Any change in current causes a corresponding change in the magnetic field surrounding the conductor. Current is constant for a regulated DC source, except when the circuit is turned on and off, or when there is a load change. However, alternating current is constantly changing, and inductance is continually opposing the change. A change in the magnetic field surrounding the conductor induces a voltage in the conductor. This self-induced voltage opposes the change in current. This is known as counter emf. All conductors have inductance, but inductors are coils of wire wound for a specific inductance. For some applications, inductors are wound around a metal core to further concentrate the inductance. The inductance of a coil is determined by the number of turns in the coil, the coil diameter and length, and the core material. An inductor is usually indicated symbolically on an electrical drawing as a curled line. Inductors in series: In the accompanying circuit, an AC source supplies electrical power to four inductors. Total inductance of series inductors is equal to the sum of the inductances. Inductors in parallel: The total inductance of inductors in parallel is calculated using a formula similar to the formula for resistance of parallel resistors. The accompanying illustration shows the calculation for a circuit with three parallel inductors. CAPACITOR Capacitance and Capacitors: Capacitance is a measure of a circuit\'s ability to store an electrical charge. A device manufactured to have a specific amount of capacitance is called a capacitor. A capacitor is made up of a pair of conductive plates separated by a thin layer of insulating material. Another name for the insulating material is dielectric material. When a voltage is applied to the plates, electrons are forced onto one plate. That plate has an excess of electrons while the other plate has a deficiency of electrons. The plate with an excess of electrons is negatively charged. The plate with a deficiency of electrons is positively charged. Direct current cannot flow through the dielectric material because it is an insulator; however, the electric field created when the capacitor is charged is felt through the dielectric. Capacitors are rated for the amount of charge they can hold. The capacitance of a capacitor depends on the area of the plates, the distance between the plates, and type of dielectric material used. The unit of measurement for capacitance is the farad (F). However, because the farad is a large unit, capacitors are often rated in microfarads or picofarads. Capacitance in series: Connecting capacitors in series decreases total capacitance. The formula for series capacitors is similar to the formula for parallel resistors. In the accompanying circuit, an AC source supplies electrical power to three capacitors. Capacitors in Parallel: Adding capacitors in parallel increases circuit capacitance. In the accompanying circuit, an AC source supplies electrical power to three capacitors. Total capacitance is determined by adding the values of the capacitors. Inductive reactance: In a purely resistive AC circuit, resistance is the only opposition to current flow. In an AC circuit with only inductance, capacitance, or both inductance and capacitance, but no resistance, opposition to current flow is called reactance, designated by the symbol \"X.\" Total opposition to current flow in an AC circuit that contains both reactance and resistance is called impedance, designated by the symbol \"Z.\" Just like resistance, reactance and impedance are expressed in ohms. Inductance only affects current flow when the current is changing. Inductance produces a self-induced voltage (counter emf) that opposes changes in current. In an AC circuit, current is changing constantly. Inductance in an AC circuit, therefore, causes a continual opposition. This opposition to current flow is called inductive reactance. Inductive reactance is proportional to both the inductance and the frequency applied. Current and voltage phases: In a purely resistive circuit, current and voltage rise and fall at the same time. They are said to be \"in phase.\" For the circuit in the top illustration, there is no inductance. Therefore, resistance and impedance are the same. in a purely inductive circuit, voltage leads current by 90 degrees. Current and voltage are said to be \"out of phase.\" For the circuit in the middle illustration, impedance and inductive reactance are the same. All circuits have some resistance, however, and in an AC circuit with both resistance and inductive reactance, voltage leads the current by more than 0 degrees and less than 90 degrees. For the circuit in the bottom illustration, resistance and inductive reactance are equal and voltage leads current by 45 degrees. Another way to say this is that current lags the voltage in a circuit with resistance and inductance. The exact amount of lag depends on the relative amounts of resistance and inductive reactance. The more resistive a circuit is, the closer it is to being in phase. The more reactive a circuit is, the more out of phase it is. Capacitive reactance: Capacitors also oppose current flow in an AC circuit. This opposition is called capacitive reactance. Capacitive reactance is inversely proportional to frequency and capacitance. Therefore, the larger the capacitor or the higher the frequency, the smaller the capacitive reactance. Current and voltage phases: For capacitive circuits, the phase relationship between current and voltage are opposite to the phase relationship for an inductive circuit. In a purely capacitive circuit, current leads voltage by 90 degrees. In an AC circuit with both resistance and capacitive reactance, current leads voltage by more than 0 degrees and less than 90 degrees. The exact amount of lead depends on the relative amounts of resistance and capacitive reactance. The more resistive a circuit is, the closer it is to being in phase. The more reactive a circuit is, the more out of phase it is. In the bottom illustration, resistance and capacitive reactance are equal and current leads voltage by 45 degrees.  Inductance only affects current flow when the current is changing. Inductance produces a self-induced voltage (counter emf) that opposes changes in current. In an AC circuit, current is changing constantly. Inductance in an AC circuit, therefore, causes a continual opposition. This opposition to current flow is called inductive reactance. Inductive reactance is proportional to both the inductance and the frequency applied. Calculating impedance: Impedance (Z) is the total opposition to current flow in an AC circuit. Impedance is often represented as a vector. A vector is a quantity that has magnitude and direction. An impedance vector diagram shows reactance and resistance vectors at right angles to each other with the impedance vector plotted at some angle in between the reactance and resistance vectors. Resistance is plotted at 0 degrees, inductive reactance at 90 degrees, and capacitive reactance at -90 degrees. The accompanying illustration shows two circuits with equal values of resistance and reactance. The upper circuit has inductive reactance and resistance and the lower circuit has capacitive reactance and resistance. As shown in the accompanying illustration, the magnitude of the impedance vectors can be determined by taking the square root of the sum of the squares of the reactance and resistance vectors. Although the magnitude of the impedance vector (141.4 ohms) is the same for both circuits, the angles of the impedance vectors are different. For the upper circuit, the impedance vector has an angle of +45 degrees, but for the lower circuit, but angle is -45 degrees. Note the relationship between the impedance vector angle and the phase relationship between voltage and current for each circuit. RLC CIRCUITS Series R-L-C Circuit: Many circuits contain values of resistance, inductance, and capacitance in series. In an inductive AC circuit, current lags voltage by 90 degrees. In a capacitive AC circuit, current leads voltage by 90 degrees. When represented in vector form, inductive reactance and capacitive reactance are plotted 180 degrees apart. As a result, the net reactance is determined by taking the difference between the two reactance\'s. The total impedance of the circuit can be calculated using the formula shown in the accompanying illustration. Once the total impedance is known, the current can be determined using Ohm\'s law. Keep in mind that because both inductive and capacitive reactance are dependent upon frequency, if the frequency of the source voltage changes, the net reactance changes. For example, if frequency increases, inductive reactance increases and capacitive reactance decreases. For the special case where the inductive reactance and capacitive reactance are equal, the circuit impedance is the total resistance. Parallel R-L-C Circuit: Many circuits contain values of resistance, inductance, and capacitance in parallel. One method for determining the total impedance for a parallel circuit is to begin by calculating the total current. In a capacitive AC circuit, current leads voltage by 90 degrees. In an inductive AC circuit, current lags voltage by 90 degrees. When represented in vector form, capacitive current and inductive current and are plotted 180 degrees apart. The net reactive current is determined by taking the difference between the reactive currents. The total current for the circuit can be calculated using the formula shown in the accompanying illustration. Once the total current is known, the total impedance can be calculated using Ohm\'s law. Keep in mind that because both inductive reactance and capacitive reactance are dependent upon frequency, if the frequency of the source voltage changes, the reactance\'s and corresponding currents change. For example, if the frequency increases, the inductive reactance increases, and the current through the inductor decreases, but the capacitive reactance decreases and the current through the capacitor increases. For the special case where the inductive reactance and capacitive reactance are equal, the inductive and capacitive currents cancel and the net current is the resistive current. In this case, the inductor and capacitor form a parallel resonant circuit. Transformers: Transformers are electromagnetic devices that transfer electrical energy from one circuit to another by mutual induction. They are frequently used to step a voltage up to a higher level or down to a lower level. A single-phase transformer has two coils, a primary coil and a secondary coil. Energy is transferred from the primary to the secondary via mutual induction. The accompanying illustration shows an AC source connected to the primary coil. The magnetic field produced by the primary induces a voltage in the secondary coil, which supplies power to a load. Mutual inductance between two coils depends on their flux linkage. Maximum coupling occurs when all the lines of flux from the primary coil cut through the secondary coil. To maximize the amount of coupling, both coils are often wound on the same iron core, which provides a good path for the lines of flux. The following discussions of step-up and step-down transformers apply to transformers with an iron core. Transformer turns ratio: There is a relationship between primary and secondary voltage, current, and impedance and the ratio of transformer primary turns to secondary turns. When the primary has fewer turns than the secondary, voltage is stepped up from primary to secondary. For the circuit on the left, the transformer secondary has twice as many turns as the primary and voltage is stepped up from 120 VAC to 240 VAC. Because the impedance of the load is also higher than the impedance of the primary, current is stepped down from 10 amps to 5 amps. When the primary has more turns than the secondary, voltage is stepped down from primary to secondary. For the circuit on the right, the primary coil has twice as many turns as the secondary coil and voltage is stepped down from 240 VAC to 120 VAC. Because the impedance of the load is lower than the impedance of the primary, the secondary current is stepped up from 5 amps to 10 amps. Transformers are rated for the amount of apparent power they can provide. Because values of apparent power are often large, the transformer rating is frequently given in kVA (kilovolt-amps). The kVA rating determines the current and voltage a transformer can deliver to its load without overheating. Residential transformer applications: The most common power supply system used in residential applications in the United States today is a single-phase, three-wire supply system. In this system, the voltage between either hot wire and neutral is 120 volts and the voltage between the two hot wires is 240 volts. The 120-volt supply is used for general-purpose receptacles and lighting. The 240 volt supply is used for heating, cooling, cooking, and other high demand loads. Three phase power: Up till now, we have been talking only about single-phase AC power. Single-phase power is used where power demands are relatively small, such as for a typical home. However, power companies generate and distribute three-phase power. Three-phase power is used in commercial and industrial applications where of power requirements are higher than those of a typical residence. Three-phase power, as shown in the accompanying illustration, is a continuous series of three overlapping AC cycles. Each wave represents a phase and is offset by 120 electrical degrees from each of the two other phases. Three phase transformers: Transformers used with three-phase power require three interconnected coils in both the primary and the secondary. These transformers can be connected in either a wye or a delta configuration. The type of transformer and the actual voltage depend on the requirements of the power company and the needs of the customer. The accompanying illustration shows the secondary of a wye-connected transformer and the secondary of a delta-connected transformer. These are only examples of possible distribution configurations, the specific voltages and configurations vary widely depending upon the application requirements. BASICSOFUNDERSTANDING OF AC CIRCUITS -POWER AND PF Power in AC Circuit: In resistive circuits, power is dissipated in heat. This is called true power or effective power because it is the rate at which energy is used. True power is equal to the current squared times the resistance. The unit for true power is the watt. Although reactive components do not consume energy, they do increase the amount of energy that must be generated to do the same amount of work. The rate at which this nonworking energy must be generated is called reactive power. The unit for reactive power the var or (VAR), which stands for volt-amperes reactive. The vector sum of true power and reactive power is called apparent power. Apparent power is also equal to the total current multiplied by the applied voltage (P = IE). The unit for apparent power is the volt-ampere (VA). Power factor: Power factor is the ratio of true power to apparent power in an AC circuit. This ratio is also the cosine of the phase angle. In a purely resistive circuit, current and voltage are in phase. This means that there is no angle of displacement between current and voltage. The cosine of a zero-degree angle is one. Therefore, the power factor is one. This means that all energy delivered by the source is consumed by the circuit and dissipated in the form of heat. In a purely reactive circuit, voltage and current are 90 degrees apart. The cosine of a 90-degree angle is zero. Therefore, the power factor is zero. This means that all the energy the circuit receives from the source is returned to the source. For the circuit in the accompanying illustration, the power factor is 0.8. This means the circuit uses 80 percent of the energy supplied by the source and returns 20 percent to the source. Another way of expressing true power is as the apparent power times the power factor. This is also equal to I times E times the cosine of the phase angle. UNDERSTANDING OF SERIES PARALLEL CIRCUITS Series Circuit Resistance: A series circuit is formed when any number of resistors are connected end-to-end so that there is only one path for current to flow. The resistors can be actual resistors or other devices that have resistance. The accompanying illustration shows five resistors connected end-to-end. There is one path of current flow from the negative terminal of the voltage source through all five resistors and returning to the positive terminal. The total resistance in a series circuit can be determined by adding all the resistor values. Although the unit for resistance is the ohm, different metric unit prefixes, such as kilo (k) or mega (M) are often used. Therefore, it is important to convert all resistance values to the same units before adding. Series circuit voltage and current The current in a series circuit can be determined using Ohm\'s law. First, total the resistance and then divide the source voltage by the total resistance. This current flows through each resistor in the circuit. The voltage measured across each resistor can be calculated using Ohm\'s law. The voltage across a resistor is often referred to as a voltage drop. The sum of the voltage drops across each resistor is equal to the source voltage. The accompanying illustration shows two voltmeters, one measuring total voltage and one measuring the voltage across R3. Parallel circuit Resistance: A parallel circuit is formed when two or more resistances are placed in a circuit side-by side so that current can flow through more than one path. The accompanying illustration shows the simplest parallel circuit, two parallel resistors. There are two paths of current flow. One path is from the negative terminal of the battery through R1 returning to the positive terminal. The second path is from the negative terminal of the battery through R2 returning to the positive terminal of the battery. The current through any resistor can be determined by dividing the circuit voltage by the resistance of that resistor. Parallel circuit resistance: The total resistance for a parallel circuit with any number of resistors can be calculated using the formula shown in the accompanying illustration. In the unique example where all resistors have the same resistance, the total resistance is equal to the resistance of one resistor divided by the number of resistors. Parallel circuit current: Current in each of the branches of a parallel circuit can be calculated by dividing the circuit voltage, which is the same for all branches, by the resistance of the branch. The total circuit current can be calculated by adding the current for all branches or by dividing the circuit voltage by the total resistance. SERIES PARALLEL CIRCUIT Series Parallel Circuit: Series-parallel circuits are also known as compound circuits. At least three resistors are required to form a series-parallel circuit. The accompanying illustration shows the two simplest series parallel circuits. The circuit on the left has two parallel resistors in series with another resistor. The circuit on the right has two series resistors in parallel with another resistor. Series-parallel circuits are usually more complex than the circuits shown here, but by using the circuit formulas discussed earlier in this course, you can easily determine circuit characteristics. Series Parallel circuit example: The accompanying illustration shows how total resistance can be determined for two series-parallel circuits in two easy steps for each circuit. More complex circuits require more steps, but each step is relatively simple. In addition, by using Ohm's law, you can also solve for current and voltage throughout each circuit, if the source voltage is known. UNDERSTANDING OF ELECTRICAL FORMULAS Ohm's Law: Ohm's Law/Power Formulas: P = Power = Watts R = Resistance = Ohms I = Current = Amperes E = Force = Volts Ohm's Law Diagram and Formulas: E = I X R Voltage = Current X Resistance. I = E / R Current = Voltage /Resistance R = E/I Resistance = Voltage / Current. Power Diagram and Formulas: I = P / E Current = Power / Voltage. E = P / I Voltage = Power /Current. P = I X E Power = Current X Voltage. Kirchhoff's Laws:  1^st^ Law At any electrical junction the current flowing into the junction is equal to the sum of currents flowing out of the junction. Therefore I1 = I2 + I3 + I4 2^nd^ Law The sum of the voltage drop across any complete path of components connected across the voltage supply equals the voltage provided by the power supply. Therefore V4 = V1 + V2 +V3 Ohm\'s Law and Impedance: E = VOLTAGE (V) I = CURRENT (A) Z = IMPEDANCE (Ω) E = I X Z I = E/Z Z = E/I Ohm's law and the power formula are limited to circuits in which electrical resistance is the only significant opposition to current flow including all DC circuits and AC circuits that do not contain a significant amount of inductance and/or capacitance. AC circuits that include inductance are any circuits that include a coil as the load such as motors, transformers, and solenoids. AC circuits that include capacitance are any circuits that include a capacitor (s). In DC and AC circuits that do not contain a significant amount of inductance and /or capacitance, the opposition to current flow is resistance (R). In circuits that contain inductance (XL) or capacitance (XC), the opposition to the flow of current is reactance (X). In circuits that contain resistance (R) and reactance (X), the combined opposition to the flow of current is impedance (Z). Resistance and Impedance are both measured in Ohms. Ohm's law is used in circuits that contain impedance, however, and Z is substituted for R in the formula. Z represents the total resistive force (resistance and reactance) opposing current flow.  Standard formulas used for power consumption calculations: Standard Electric Motor formulas:  UNDERSTANDING OF POWER TRIANGLE Power Triangle: In AC circuits, current and voltage are normally out of phase and, as a result, not all the power produced by the generator can be used to accomplish work. By the same token, power cannot be calculated in AC circuits in the same manner as in DC circuits. The power triangle, shown in Figure 1, equates AC power to DC power by showing the relationship between generator output (apparent power - S) in volt-amperes (VA), usable power (true power - P) in watts, and wasted or stored power (reactive power - Q) in voltamperes-reactive (VAR). The phase angle (Θ) represents the inefficiency of the AC circuit and corresponds to the total reactive impedance (Z) to the current flow in the circuit. The power triangle represents comparable values that can be used directly to find the efficiency level of generated power to usable power, which is expressed as the power factor. Apparent power, reactive power, and true power can be calculated by using the DC equivalent (RMS value) of the AC voltage and current components along with the power factor. True Power: True power (P) is the power consumed by the resistive loads in an electrical circuit. Given Equation is a mathematical representation of true power. The measurement of true power is in watts. Where: P = true power (watts), I = RMS current (A), E = RMS voltage (V), R = resistance (Ω) and Θ= angle between E and I sine waves Apparent Power: Apparent power (S) is the power delivered to an electrical circuit. Given Equation is a mathematical representation of apparent power. The measurement of apparent power is in volt amperes (VA). Where: S = apparent power (VA), I = RMS current (A), E = RMS voltage (V) and Z = impedance (Ohms) Reactive power: Reactive power (Q) is the power consumed in an AC circuit because of the expansion and collapse of magnetic (inductive) and electrostatic (capacitive) fields. Reactive power is expressed in volt-amperes-reactive (VAR). Given Equation is a mathematical representation for reactive power. Where: Q = reactive power (VAR), I = RMS current (A), X = net reactance (Ω), E = RMS voltage (V) and Θ= angle between the E and I sine waves. Unlike true power, reactive power is not useful power because it is stored in the circuit itself. This power is stored by inductors, because they expand and collapse their magnetic fields in an attempt to keep current constant, and by capacitors, because they charge and discharge in an attempt to keep voltage constant. Circuit inductance and capacitance consume and give back reactive power. Reactive power is a function of a system's amperage. The power delivered to the inductance is stored in the magnetic field when the field is expanding and returned to the source when the field collapses. The power delivered to the capacitance is stored in the electrostatic field when the capacitor is charging and returned to the source when the capacitor discharges. None of the power delivered to the circuit by the source is consumed. It is all returned to the source. The true power, which is the power consumed, is thus zero. We know that alternating current constantly changes; thus, the cycle of expansion and collapse of the magnetic and electrostatic fields constantly occurs. Total Power: The total power delivered by the source is the apparent power. Part of this apparent power, called true power, is dissipated by the circuit resistance in the form of heat. The rest of the apparent power is returned to the source by the circuit inductance and capacitance. Table of content SN SUBJECT PAGE NUMBER ---- -------------------------- ------------- 1 Introduction 61 2 Switch Types 62 3 Process Switches 64 4 Pole and Throw 70 5 Contact \"normal\" state 74 6 Generic Symbology 75 7 Review 79 Objectives SN Objectives ---- ------------------------------------------------------ 1 To understand type, process and contacts of switches Introduction Switches An electrical switch is any device used to interrupt the flow of electrons in a circuit. Switches are essentially binary devices: they are either completely on \"closed\" or completely off \"open\". There are many different types of switches, and we will explore some of these types in this chapter. Though it may seem strange to cover this elementary electrical topic at such a late stage in this book series, I do so because the chapters that follow explore an older realm of digital technology based on mechanical switch contacts rather than solid-state gate circuits, and a thorough understanding of switch types is necessary for the undertaking. Learning the function of switch-based circuits at the same time that you learn about solid-state logic gates makes both topics easier to grasp and sets the stage for an enhanced learning experience in Boolean algebra, the mathematics behind digital logic circuits. The simplest type of switch is one where two electrical conductors are brought in contact with each other by the motion of an actuating mechanism. Other switches are more complex, containing electronic circuits able to turn on or off depending on some physical stimulus (such as light or magnetic field) sensed. In any case, the final output of any switch will be (at least) a pair of wire-connection terminals that either will be connected together by the switch\'s internal contact mechanism (\"closed\"), or not connected together (\"open\"). Switch Types **Hand Switches** Any switch designed to be operated by [a person] is generally called a hand switch, and they are manufactured in several varieties: Toggle switch  Toggle switches are actuated by a lever angled in one of two or more positions. The common light switch used in household wiring is an example of a toggle switch. Most toggle switches will come to rest in any of their lever positions, while others have an internal spring mechanism returning the lever to a certain normal position, allowing for what is called \"momentary\" operation. Pushbutton Switch Pushbutton switches are two-position devices actuated with a button that is pressed and released. Most pushbutton switches have an internal spring mechanism returning the button to its \"out,\" or \"unpressed,\" position, for momentary operation. Some pushbutton switches will latch alternately on or off with every push of the button. Other pushbutton switches will stay in their \"in,\" or \"pressed,\" position until the button is pulled back out. This last type of pushbutton switches usually have a mushroom-shaped button for easy push-pull action. Selector switch  Selector switches are actuated with a rotary knob or lever of some sort to select one of two or more positions. Like the toggle switch, selector switches can either rest in any of their positions or contain spring-return mechanisms for momentary operation. Joystick switch A joystick switch is actuated by a lever free to move in more than one axis of motion. One or more of several switch contact mechanisms are actuated depending on which way the lever is pushed, and sometimes by how far it is pushed. The circle and-dot notation on the switch symbol represents the direction of joystick lever motion required to actuate the contact. Joystick hand switches are commonly used for crane and robot control. **Process Switches** Limit Switch Some switches are specifically designed to be operated by the motion of a machine rather than by the hand of a human operator. These motion-operated switches are commonly called limit switches, because they are often used to limit the motion of a machine by turning off the actuating power to a component if it moves too far. As with hand switches, limit switches come in several varieties: -  These limit switches closely resemble rugged toggle or selector hand switches fitted with a lever pushed by the machine part. Often, the levers are tipped with a small roller bearing, preventing the lever from being worn off by repeated contact with the machine part. - Proximity switches sense the approach of a metallic machine part either by a magnetic or high-frequency electromagnetic field. Simple proximity switches use a permanent magnet to actuate a sealed switch mechanism whenever the machine part gets close (typically 1 inch or less). More complex proximity switches work like a metal detector, energizing a coil of wire with a high-frequency current, and electronically monitoring the magnitude of that current. If a metallic part (not necessarily magnetic) gets close enough to the coil, the current will increase, and trip the monitoring circuit. The symbol shown here for the proximity switch is of the electronic variety, as indicated by the diamond-shaped box surrounding the switch. A non-electronic proximity switch would use the same symbol as the lever-actuated limit switch. Another form of proximity switch is the optical switch, comprised of a light source and photocell. Machine position is detected by either the interruption or reflection of a light beam. Optical switches are also useful in safety applications, where beams of light can be used to detect personnel entry into a dangerous area.  Photoelectric proximity sensor In many industrial processes, it is necessary to monitor various physical quantities with switches. Such switches can be used to sound alarms, indicating that a process variable has exceeded normal parameters, or they can be used to shut down processes or equipment if those variables have reached dangerous or destructive levels. There are many different types of process switches: Speed Switch These switches sense the rotary speed of a shaft either by a centrifugal weight mechanism mounted on the shaft, or by some kind of non-contact detection of shaft motion such as optical or magnetic. Pressure switch  Gas or liquid pressure can be used to actuate a switch mechanism if that pressure is applied to a piston, diaphragm, or bellows, which converts pressure to mechanical force. Temperature switch An inexpensive temperature-sensing mechanism is the \"bimetallic strip:\" a thin strip of two metals, joined back-to-back, each metal having a different rate of thermal expansion. When the strip heats or cools, differing rates of thermal expansion between the two metals causes it to bend. The bending of the strip can then be used to actuate a switch contact mechanism. Other temperature switches use a brass bulb filled with either a liquid or gas, with a tiny tube connecting the bulb to a pressure sensing switch. As the bulb is heated, the gas or liquid expands, generating a pressure increase which then actuates the switch mechanism. Liquid level (Float) Switch  A floating object can be used to actuate a switch mechanism when the liquid level in an tank rises past a certain point. If the liquid is electrically conductive, the liquid itself can be used as a conductor to bridge between two metal probes inserted into the tank at the required depth. The conductivity technique is usually implemented with a special design of relay triggered by a small amount of current through the conductive liquid. In most cases it is impractical and dangerous to switch the full load current of the circuit through a liquid. Level switches can also be designed to detect the level of solid materials such as wood chips, grain, coal, or animal feed in a storage silo, bin, or hopper. A common design for this application is a small paddle wheel, inserted into the bin at the desired height, which is slowly turned by a small electric motor. When the solid material fills the bin to that height, the material prevents the paddle wheel from turning. The torque response of the small motor than trips the switch mechanism. Another design uses a \"tuning fork\" shaped metal prong, inserted into the bin from the outside at the desired height. The fork is vibrated at its resonant frequency by an electronic circuit and magnet/electromagnet coil assembly. When the bin fills to that height, the solid material dampens the vibration of the fork, the change in vibration amplitude and/or frequency detected by the electronic circuit. Liquid flow switch Inserted into a pipe, a flow switch will detect any gas or liquid flow rate in excess of a certain threshold, usually with a small paddle or vane which is pushed by the flow. Other flow switches are constructed as differential pressure switches, measuring the pressure drop across a restriction built into the pipe. Another type of level switch, suitable for liquid or solid material detection, is the nuclear switch. Composed of a radioactive source material and a radiation detector, the two are mounted across the diameter of a storage vessel for either solid or liquid material. Any height of material beyond the level of the source/detector arrangement will attenuate the strength of radiation reaching the detector. This decrease in radiation at the detector can be used to trigger a relay mechanism to provide a switch contact for measurement, alarm point, or even control of the vessel level. Nuclear level Switch (for solid or liquid material)  Both source and detector are outside of the vessel, with no intrusion at all except the radiation flux itself. The radioactive sources used are weak and pose no immediate health threat to operations or maintenance personnel. As usual, there is usually more than one way to implement a switch to monitor a physical process or serve as an operator control. There is usually no single \"perfect\" switch for any application, although some obviously exhibit certain advantages over others. Switches must be intelligently matched to the task for efficient and reliable operation. **Pole and Throw** Circuit symbols of switches, the common terminal in SPDT and DPDT types. https://qph.fs.quoracdn.net/main-qimg-37ed73cc8e0b400a35eff35f78f11b95  Since relays are switches, the terminology applied to switches is also applied to relays. A relay will switch one or more poles, each of whose contacts can be thrown by energizing the coil in one of three ways: - Normally-open (NO) contacts connect the circuit when the relay is activated; the circuit is disconnected when the relay is inactive. It is also called a Form A contact or \"make\" contact. - Normally-closed (NC) contacts disconnect the circuit when the relay is activated; the circuit is connected when the relay is inactive. It is also called a Form B contact or \"break\" contact. Change-over (CO), or double-throw (DT), contacts control two circuits: one normally-open contact and one normally-closed contact with a common terminal. It is also called a Form C contact or \"transfer\" contact (\"break before make\"). If this type of contact utilizes a \"make before break\" functionality, then it is called a Form D contact. The following designations are commonly encountered: - SPST - Single Pole Single Throw. These have two terminals which can be connected or disconnected. Including two for the coil, such a relay has four terminals in total. It is ambiguous whether the pole is normally open or normally closed. The terminology \"SPNO\" and \"SPNC\" is sometimes used to resolve the ambiguity. - SPDT - Single Pole Double Throw. A common terminal connects to either of two others. Including two for the coil, such a relay has five terminals in total. - DPST - Double Pole Single Throw. These have two pairs of terminals. Equivalent to two SPST switches or relays actuated by a single coil. Including two for the coil, such a relay has six terminals in total. The poles may be Form A or Form B (or one of each). - DPDT - Double Pole Double Throw. These have two rows of change-over terminals. Equivalent to two SPDT switches or relays actuated by a single coil. Such a relay has eight terminals, including the coil. The \"S\" or \"D\" may be replaced with a number, indicating multiple switches connected to a single actuator. For example, 4PDT indicates a four-pole double throw switch. Here are a few common switch configurations and their abbreviated designations:  Four-pole, double-throw (4 PDT) Contact \"normal\" state and make/ break sequence. Any kind of switch contact can be designed so that the contacts \"close\" (establish continuity) when actuated, or \"open\" (interrupt continuity) when actuated. For switches that have a spring-return mechanism in them, the direction that the spring returns it to with no applied force is called the normal position. Therefore, contacts that are open in this position are called normally open and contacts that are closed in this position are called normally closed. For process switches, the normal position, or state, is that which the switch is in when there is no process influence on it. An easy way to figure out the normal condition of a process switch is to consider the state of the switch as it sits on a storage shelf, uninstalled. Here are some examples of \"normal\" process switch conditions: - **Speed switch**: Shaft not turning - **Pressure switch**: Zero applied pressure - **Temperature switch**: Ambient (room) temperature - **Level switch**: Empty tank or bin - **Flow switch**: Zero liquid flow It is important to differentiate between a switch\'s \"normal\" condition and its \"normal\" use in an operating process. Consider the example of a liquid flow switch that serves as a low-flow alarm in a cooling water system. The normal, or properly operating, condition of the cooling water system is to have fairly constant coolant flow going through this pipe. If we want the flow switch\'s contact to close in the event of a loss of coolant flow (to complete an electric circuit which activates an alarm siren, for example), we would want to use a flow switch with normally-closed rather than normally-open contacts. When there\'s adequate flow through the pipe, the switch\'s contacts are forced open; when the flow rate drops to an abnormally low level, the contacts return to their normal (closed) state. This is confusing if you think of \"normal\" as being the regular state of the process, so be sure to always think of a switch\'s \"normal\" state as that which its in as it sits on a shelf. The schematic symbology for switches vary according to the switch\'s purpose and actuation. A normally-open switch contact is drawn in such a way as to signify an open connection, ready to close when actuated. Conversely, a normally-closed switch is drawn as a closed connection which will be opened when actuated. Generic Symbology Normally-open Normally-closed  Pushbutton switch There is also a generic symbology for any switch contact, using a pair of vertical lines to represent the contact points in a switch. Normally-open contacts are designated by the lines not touching, while normally-closed contacts are designated with a diagonal line bridging between the two lines. Compare the two: Generic switch contact designation Normally-open Normally-closed The switch on the left will close when actuated and will be open while in the \"normal\" (unactuated) position. The switch on the right will open when actuated and is closed in the \"normal\" (unactuated) position. If switches are designated with these generic symbols, the type of switch usually will be noted in text immediately beside the symbol. Please note that the symbol on the left is not to be confused with that of a capacitor. If a capacitor needs to be represented in a control logic schematic, it will be shown like this:  Capacitor In standard electronic symbology, the figure shown above is reserved for polarity sensitive capacitors. In control logic symbology, this capacitor symbol is used for any type of capacitor, even when the capacitor is not polarity sensitive, so as to clearly distinguish it from a normally-open switch contact. With multiple-position selector switches, another design factor must be considered: that is, the sequence of breaking old connections and making new connections as the switch is moved from position to position, the moving contact touching several stationary contacts in sequence, The selector switch shown above switches a common contact lever to one of five different positions, to contact wires numbered 1 through 5. The most common configuration of a multi-position switch like this is one where the contact with one position is broken before the contact with the next position is made. This configuration is called break-before-make. To give an example, if the switch were set at position number 3 and slowly turned clockwise, the contact lever would move off of the number 3 position, opening that circuit, move to a position between number 3 and number 4 (both circuit paths open), and then touch position number 4, closing that circuit. There are applications where it is unacceptable to completely open the circuit attached to the \"common\" wire at any point in time. For such an application, a make-before-break switch design can be built, in which the movable contact lever actually bridges between two positions of contact (between number 3 and number 4, in the above scenario) as it travels between positions. The compromise here is that the circuit must be able to tolerate switch closures between adjacent position contacts (1 and 2, 2 and 3, 3 and 4, 4 and 5) as the selector knob is turned from position to position. Such a switch is shown here: When movable contact(s) can be brought into one of several positions with stationary contacts, those positions are sometimes called throws. The number of movable contacts is sometimes called poles. Both selector switches shown above with one moving contact and five stationary contacts would be designated as \"single pole, five-throw\" switches. If two identical single-pole, five-throw switches were mechanically ganged together so that they were actuated by the same mechanism, the whole assembly would be called a \"double-pole, five-throw\" switch: REVIEW: - - - - - - - - - Table of content SN SUBJECT PAGE NUMBER ---- ---------------------------------- ------------- 1 Introduction 84 2 Contactor 84 3 Construction 85 4 Operating Principle 87 5 Rating 88 4 Relay 89 5 Basic Design and Operation 95 6 Types of Relay 97 7 Applications 108 8 Relay Application Considerations 109 Objectives SN Objectives ---- ------------------------------------------------------------------ 1 To understand Electrical Contactors and Relays 2 Contactor and Relay operation principle, types and applications. Electromechanical Switches  **\ ** Introduction Contactor ========= In semiconductor testing, contactor can also refer to the specialized socket that connects the device under test. In process industries, contactor is a vessel where two streams interact, for example, air and liquid. AC contactor for pump application. A contactor is an electro-magnetic switching device [used for remotely switching a power or control circuit]. A contactor is activated by a control input which is a lower voltage/current than that which the contactor is switching. Contactors come in many forms with varying capacities and features. Unlike a circuit breaker, a contactor is not intended to interrupt a short circuit current. Contactors range from having a breaking current of several amps and 110 volts to thousands of amps and many kilovolts. The physical size of contactors ranges from a device small enough to pick up with one hand, to large devices approximately a meter on a side. Contactors are used to control electric motors, lighting, heating, capacitor banks, and other electrical loads. What is an electromechanical switch? ----------------------------------------------------------------------------- An electromechanical switch is an electrical switch actuated by a solenoid. Construction ============  Albright SPST DC Contactor, --------------------------- A contactor is composed of three different systems. 1. 2. 3. Contactors used for starting electric motors are commonly fitted with overload protection to prevent damage to their loads. When an overload is detected, the contactor is tripped, removing power downstream from the contactor. High voltage contactors (greater than 1000 volts) often have arc suppression systems fitted (such as a vacuum or an inert gas surrounding the contacts). Magnetic blowouts are sometimes used to increase the amount of current a contactor can successfully break. The magnetic field produced by the blowout coils force the electric arc to lengthen and move away from the contacts. This is especially useful in contactors used in DC power circuits; AC arcs have periods of low current, during which the arc can be extinguished with relative ease, but DC arcs have continuous high current, so blowing them out requires the arc to be stretched further than an AC arc of the same current. The magnetic blowouts in the pictured Albright contactor (which is designed for DC currents) more than double the current it can break, increasing it from 600 amps to 1500 amps. Sometimes an economizer circuit is also installed to reduce the power required to keep a contactor closed. A somewhat greater amount of power is required to initially close a contactor than is required to keep it closed thereafter. Such a circuit can save a substantial amount of power and allow the energized coil to stay cooler. Economizer circuits are nearly always applied on direct-current contactor coils and on large alternating current contactor coils. Contactors are often used to provide central control of large lighting installations, such as an office building or retail building. To reduce power consumption in the contactor coils, latching contactors are used, which have two operating coils. One coil, momentarily energized, closes the power circuit contacts, which are then mechanically held closed; the second coil opens the contacts. A basic contactor will have a coil input (which may be driven by either an AC or DC supply depending on the contactor design). [The coil may be energized at the same voltage as the motor or may be separately controlled with a lower coil voltage better suited to control by programmable controllers and lower-voltage pilot devices]. Certain contactors have series coils connected in the motor circuit; these are used, for example, for automatic acceleration control, where the next stage of resistance is not cut out until the motor current has dropped. Operating Principle Unlike general-purpose relays, [contactors are designed to be directly connected to high-current load devices]. Relays tend to be of lower capacity and are usually designed for both Normally Closed and Normally Open applications. Devices switching more than 15 amperes or in circuits rated more than a few kilowatts are usually called contactors. Apart from optional auxiliary low current contacts, [contactors are almost exclusively fitted with Normally Open contacts]. Unlike relays, contactors are designed with features to control and suppress the arc produced when interrupting heavy motor currents. When current passes through the electromagnet, a magnetic field is produced which attracts ferrous objects, in this case the moving core of the contactor is attracted to the stationary core. Since there is an air gap initially, the electromagnet coil draws more current initially until the cores meet and redact the gap, increasing the inductive impedance of the circuit. The moving contact is propelled by the moving core; the force developed by the electromagnet holds the moving and fixed contacts together. When the contactor coil is de-energized, gravity or a spring returns the electromagnet core to its initial position and opens the contacts. Most motor control contactors at low voltages (600 volts and less) are \"air break\" contactors, since ordinary air surrounds the contacts and extinguishes the arc when interrupting the circuit. Modern medium-voltage motor controllers use vacuum contactors. Motor control contactors can be fitted with short-circuit protection (fuses or circuit breakers), disconnecting means, overload relays and an enclosure to make a combination starter. In large industrial plants many contactors may be assembled in motor control centers. Ratings ------- Contactors are rated by designed [load current] per contact (pole), [maximum fault withstand current], [duty cycle], [voltage], and [coil voltage]. A general purpose motor control contactor may be suitable for heavy starting duty on large motors; so-called \"definite purpose\" contactors are carefully adapted to such applications as air conditioning compressor motor starting. North American and European ratings for contactors follow different philosophies, with North American general purpose machine tool contactors generally emphasizing simplicity of application while definite purpose and European rating philosophy emphasizes design for the intended life cycle of the application. Relay ----- ### Automotive style miniature relay A relay is an electrical switch that opens and closes under the control of another electrical circuit. In the original form, the switch is operated by an electromagnet to open or close one or many sets of contacts. It was invented by Joseph Henry in 1835. Because a relay is able to control an output circuit of higher power than the input circuit, it can be considered to be, in a broad sense, a form of an electrical amplifier. Relay Construction ------------------ An electric current through a conductor will produce a magnetic field at right angles to the direction of electron flow. If that conductor is wrapped into a coil shape, the magnetic field produced will be oriented along the length of the coil. The greater the current, the greater the strength of the magnetic field, all other factors being equal: ![Explained\] Main Differences Between Relay and Contactor - ETechnoG](media/image113.png) Inductors react against changes in current because of the energy stored in this magnetic field. When we construct a transformer from two inductor coils around a common iron core, we use this field to transfer energy from one coil to the other. However, there are simpler and more direct uses for electromagnetic fields than the applications we\'ve seen with inductors and transformers. The magnetic field produced by a coil of current-carrying wire can be used to exert a mechanical force on any magnetic object, just as we can use a permanent magnet to attract magnetic objects, except that this magnet (formed by the coil) can be turned on or off by switching the current on or off through the coil. If we place a magnetic object near such a coil for the purpose of making that object move when we energize the coil with electric current, we have what is called a solenoid. The movable magnetic object is called an armature, and most armatures can be moved with either direct current (DC) or alternating current (AC) energizing the coil. The polarity of the magnetic field is irrelevant for the purpose of attracting an iron armature. Solenoids can be used to electrically open door latches, open or shut valves, move robotic limbs, and even actuate electric switch mechanisms. However, if a solenoid is used to actuate a set of switch contacts, we have a device so useful it deserves its own name: the relay. Relays are extremely useful when we have a need to control a large amount of current and/or voltage with a small electrical signal. The relay coil which produces the magnetic field may only consume fractions of a watt of power, while the contacts closed or opened by that magnetic field may be able to conduct hundreds of times that amount of power to a load. In effect, a relay acts as a binary (on or off) amplifier. Just as with transistors, the relay\'s ability to control one electrical signal with another finds application in the construction of logic functions. This topic will be covered in greater detail in another lesson. For now, the relay\'s \"amplifying\" ability will be explored. In the above schematic, the relay\'s coil is energized by the low-voltage (12 VDC) source, while the single-pole, single-throw (SPST) contact interrupts the high-voltage (480 VAC) circuit. It is quite likely that the current required to energize the relay coil will be hundreds of times less than the current rating of the contact. Typical relay coil currents are well below 1 amp, while typical contact ratings for industrial relays are at least 10 amps. One relay coil/armature assembly may be used to actuate more than one set of contacts. Those contacts may be normally-open, normally-closed, or any combination of the two. As with switches, the \"normal\" state of a relay\'s contacts is that state when the coil is de-energized, just as you would find the relay sitting on a shelf, not connected to any circuit. Relay contacts may be open-air pads of metal alloy, mercury tubes, or even magnetic reeds, just as with other types of switches. The choice of contacts in a relay depends on the same factors which dictate contact choice in other types of switches. Open-air contacts are the best for high-current applications, but their tendency to corrode and spark may cause problems in some industrial environments. Mercury and reed contacts are sparkless and won\'t corrode, but they tend to be limited in current-carrying capacity. Shown here are three small relays (about two inches in height, each), installed on a panel as part of an electrical control system at a municipal water treatment plant: The relay units shown here are called \"octal-base,\" because they plug into matching sockets, the electrical connections secured via eight metal pins on the relay bottom. The screw terminal connections you see in the photograph where wires connect to the relays are actually part of the socket assembly, into which each relay is plugged. This type of construction facilitates easy removal and replacement of the relay(s) in the event of failure. Aside from the ability to allow a relatively small electric signal to switch a relatively large electric signal, relays also offer electrical isolation between coil and contact circuits. This means that the coil circuit and contact circuit(s) are electrically insulated from one another. One circuit may be DC and the other AC (such as in the example circuit shown earlier), and/or they may be at completely different voltage levels, across the connections or from connections to ground. While relays are essentially binary devices, either being completely on or completely off, there are operating conditions where their state may be indeterminate, just as with semiconductor logic gates. In order for a relay to positively \"pull in\" the armature to actuate the contact(s), there must be a certain minimum amount of current through the coil. This minimum amount is called the pull-in current, and it is analogous to the minimum input voltage that a logic gate requires to guarantee a \"high\" state (typically 2 Volts for TTL, 3.5 Volts for CMOS). Once the armature is pulled closer to the coil\'s center, however, it takes less magnetic field flux (less coil current) to hold it there. Therefore, the coil current must drop below a value significantly lower than the pull-in current before the armature \"drops out\" to its spring-loaded position and the contacts resume their normal state. This current level is called the drop-out current, and it is analogous to the maximum input voltage that a logic gate input will allow to guarantee a \"low\" state (typically 0.8 Volts for TTL, 1.5 Volts for CMOS). The hysteresis, or difference between pull-in and drop-out currents, results in operation that is similar to a Schmitt trigger logic gate. Pull-in and drop-out currents (and voltages) vary widely from relay to relay, and are specified by the manufacturer. REVIEW: - - - - Basic Design and Operation --------------------------  Simple electromechanical relay small relay as used in electronics A simple electromagnetic relay, such as the one taken from a car in the first picture, is an adaptation of an electromagnet. It consists of a coil of wire surrounding a soft iron core, an iron yoke, which provides a low reluctance path for magnetic flux, a moveable iron armature, and a set, or sets, of contacts; two in the relay pictured. The armature is hinged to the yoke and mechanically linked to a moving contact or contacts. It is held in place by a spring so that when the relay is de-energized there is an air gap in the magnetic circuit. In this condition, one of the two sets of contacts in the relay pictured is closed, and the other set is open. Other relays may have more or fewer sets of contacts depending on their function. The relay in the picture also has a wire connecting the armature to the yoke. This ensures continuity of the circuit between the moving contacts on the armature, and the circuit track on the Printed Circuit Board (PCB) via the yoke, which is soldered to the PCB. When an electric current is passed through the coil, the resulting magnetic field attracts the armature, and the consequent movement of the movable contact or contacts either makes or breaks a connection with a fixed contact. If the set of contacts was closed when the relay was de-energized, then the movement opens the contacts and breaks the connection, and vice versa if the contacts were open. When the current to the coil is switched off, the armature is returned by a force, approximately half as strong as the magnetic force, to its relaxed position. Usually this force is provided by a spring, but gravity is also used commonly in industrial motor starters. Most relays are manufactured to operate quickly. In a low voltage application, this is to reduce noise. In a high voltage or high current application, this is to reduce arcing. If the coil is energized with DC, a diode is frequently installed across the coil, to dissipate the energy from the collapsing magnetic field at deactivation, which would otherwise generate a voltage spike dangerous to circuit components. Some automotive relays already include that diode inside the relay case. Alternatively, a contact protection network, consisting of a capacitor and resistor in series, may absorb the surge. If the coil is designed to be energized with AC, a small copper ring can be crimped to the end of the solenoid. This \"shading ring\" creates a small out of phase current, which increases the minimum pull on the armature during the AC cycle. By analogy with the functions of the original electromagnetic device, a solid-state relay is made with a thyristor or other solid-state switching device. To achieve electrical isolation an optocoupler can be used which is a light-emitting diode (LED) coupled with a photo transistor. Relay Types - ### Latching relay  A latching relay has two relaxed states (bitable). These are also called \'keep\' or \'stay\' relays. When the current is switched off, the relay remains in its last state. This is achieved with a solenoid operating a ratchet and cam mechanism, or by having two opposing coils with an over-center spring or permanent magnet to hold the armature and contacts in position while the coil is relaxed, or with a remnant core. In the ratchet and cam example, the first pulse to the coil turns the relay on and the second pulse turns it off. In the two coil example, a pulse to one coil turns the relay on and a pulse to the opposite coil turns the relay off. This type of relay has the advantage that it consumes power only for an instant, while it is being switched, and it retains its last setting across a power outage. - ### Lockout Relay Lock-out Relay 86 lock out relay is hand reset used for mechanical protection (permanent fault) is an electrically operated, hand or electrically reset, auxiliary relay that is operated upon the occurrence of abnormal conditions to maintain associated equipment or devices out of service until it is reset. - ### Reed relay  A reed relay has a set of contacts inside a vacuum or inert gas filled glass tube, which protects the contacts against atmospheric corrosion. The contacts are closed by a magnetic field generated when current passes through a coil around the glass tube. Reed relays are capable of faster switching speeds than larger types of relays but have low switch current and voltage ratings. See also reed switch. - ### Mercury-wetted A mercury-wetted reed relay is a form of reed relay in which the contacts are wetted with mercury. Such relays are used to switch low-voltage signals (one volt or less) because of its low contact resistance, or for high-speed counting and timing applications where the mercury eliminates contact bounce. Mercury wetted relays are position-sensitive and must be mounted vertically to work properly. Because of the toxicity and expense of liquid mercury, these relays are rarely specified for new equipment. See also mercury switch. - Machine tool Relay ------------------  A machine tool relay is a type standardized for industrial control of machine tools, transfer machines, and other sequential control. They are characterized by a large number of contacts (sometimes extendable in the field) which are easily converted from normally-open to normally-closed status, easily replaceable coils, and a form factor that allows compactly installing many relays in a control panel. Although such relays once were the backbone of automation in such industries as automobile assembly, the programmable logic controller (PLC) mostly displaced the machine tool relay from sequential control applications. - Solid-state -----------  Solid-state relay, which has no moving parts. 25 amp or 40 amp solid-state contactors A solid-state relay (SSR) is a solid state electronic component that provides a similar function to an electromechanical relay but [does not have any moving components], increasing long-term reliability. With early SSR\'s, the tradeoff came from the fact that every transistor has a small voltage drop across it. This voltage drop limited the amount of current a given SSR could handle. As transistors improved, higher current SSR\'s, able to handle 100 to 1,200 amps, have become commercially available. Compared to electromagnetic relays, they may be falsely triggered by transients. - Buchholz Relay -------------- 1. Motorized tap changer ===================== 2. Pressure Relief Device (PRD) Relay ================================== 3. Buchholz Relay ============== 4. Sudden (or Ramp) Pressure Rise Relay In the field of electric power distribution and transmission, a Buchholz relay, also called a gas relay or a sudden pressure relay, is a safety device mounted on some oil-filled power transformers and reactors, equipped with an external overhead oil reservoir called a conservator. The Buchholz Relay is [used as a protective device sensitive to the effects of dielectric failure inside the equipment.] The relay has two different detection modes. On a slow accumulation of gas, due perhaps to slight overload, gas produced by decomposition of insulating oil accumulates in the top of the relay and forces the oil level down. A float operated switch in the relay is used to initiate an alarm signal. This same switch will also operate on low oil level, such as a slow oil leak. If an arc forms, gas accumulation is rapid, and oil flows rapidly into the conservator. This flow of oil operates a switch attached to a vane located in the path of the moving oil. This switch normally will operate a circuit breaker to isolate the apparatus before the fault causes additional damage. Buchholz relays have a test port to allow the accumulated gas to be withdrawn for testing. Flammable gas found in the relay indicates some internal fault such as overheating or arcing, whereas air found in the relay may only indicate low oil level or a leak. Buchholz relays have been applied to large power transformers at least since the 1940\'s. The relay was first developed by Max Buchholz (1875-1956) in 1921. - Buchholz Relay Principle of Operation ------------------------------------- The Buchholz relay is sited in the pipework between the transformer and its conservator and is filled with oil during normal transformer operation. When gas is generated in the transformer it rises towards the conservator and collects in the upper chamber of the relay. The oil level drops and the top float triggers alarm switch. If gas continues to be generated then the second float operates the second switch which is normally used to isolate the transformer. Also connected to the second switch is the rapid oil movement vein. The operation of this vein can be adjusted to suit the transformer design and the speed of the oil. In the event of an oil leak the Buchholz relay will only operate after the conservator has exhausted all of its oil. In order to check this eventuality it is recommended that an RDR Mk Il automatic shutter valve is fitted between the Buchholz and the conservator.  - Forced-guided contacts relay ---------------------------- A forced-guided contacts relay has relay contacts that are mechanically linked together, so that when the relay coil is energized or de-energized, all of the linked contacts move together. If one set of contacts in the relay becomes immobilized, no other contact of the same relay will be able to move. The function of forced-guided contacts is to enable the safety circuit to check the status of the relay. Forced-guided contacts are also known as \"positive-guided contacts\", \"captive contacts\", \"locked contacts\", or \"safety relays\". - Overload protection relay -------------------------  Electronic Overload Protection Relay One type of electric motor overload protection relay is operated by a heating element in series with the electric motor. The heat generated by the motor current operates a bi-metal strip or melts solder, releasing a spring to operate contacts. Where the overload relay is exposed to the same environment as the motor, a useful though crude compensation for motor ambient temperature is provided. Applications Relays are used to and for: - - - - - - - Relay Application Considerations -------------------------------- A large relay with two coils and many sets of contacts, used in an old telephone switching system.  Several 30-contact relays in \"Connector\" circuits in mid 20th century [1 XB switch] and [5XB switch] telephone exchanges; cover removed on one. Selection of an appropriate relay for a particular application requires evaluation of many different factors: - Number and type of contacts - normally open, normally closed, (double throw) - Contact sequence \"Make before Break\" or \"Break before Make\". For example, the old style telephone exchanges required Make-before-break so that the connection didn\'t get dropped while dialing the number. - Rating of contacts - small relays switch a few amperes, large contactors are rated for up to 3000 amperes, alternating or direct current - Voltage rating of contacts - typical control relays rated 300 VAC or 600 VAC, automotive types to 50 VDC, special high-voltage relays to about 15000V - Coil voltage - machine-tool relays usually 24 VAC, 120 or 250 VAC, relays for switchgear may have 125 V or 250 VDC coils, \"sensitive\" relays operate on a few milliamperes - Coil current - Usually in the range of 40 - 200 mA for 0 - 24 VDC coils. Package/enclosure - open, touch-safe, double-voltage for isolation between circuits, explosion proof, outdoor, oil and splash resistant, washable for printed circuit board assembly - Assembly - Some relays feature a sticker that keeps the enclosure sealed to allow PCB post soldering cleaning agents. Which is removed once assembly is complete. - Mounting - sockets, plug board, rail mount, panel mount, through-panel mount, enclosure for mounting on walls or equipment - Switching time - where high speed is required - \"Dry\" contacts - when switching very low level signals, special contact materials may be needed such as gold-plated contacts - Contact protection - suppress arcing in very inductive circuits - Coil protection - suppress the surge voltage produced when switching the coil current - Isolation between coil circuit and contacts - Expected mechanical loads due to acceleration - some relays used in aero space applications are designed to function in shock loads of 50 g or more - Accessories such as timers, auxiliary contacts, pilot lamps, test buttons Regulatory approvals - Stray magnetic linkage between coils of adjacent relays on a printed circuit board.  Table of content SN SUBJECT PAGE NUMBER ---- ------------------------------- ------------- 1 Introduction 115 2 Time-delay Relays 116 3 normally-open, timed-closed 116 4 normally-open, timed-open 117 5 normally-closed, timed-open 118 6 normally-closed, timed-close 119 7 Time Delay and Logic Circuits 120 Objectives SN Objectives ---- ----------------------------------------------- 1 To understanding Time-delay Relays operation 2 Introduction to Time Delay and Logic Circuits Introduction ============ Time-delay Relays ================= Some relays are constructed with a kind of \"shock absorber\" mechanism attached to the armature which prevents immediate, full motion when the coil is either energized or de-energized. This addition gives the relay the property of time-delay actuation. Time-delay relays can be constructed to delay armature motion on coil energization, de-energization, or both. Time-delay relay contacts must be specified not only as either normally-open or normally-closed, but whether the delay operates in the direction of closing or in the direction of opening. The following is a description of the four basic types of time delay relay contacts. +-----------------------------------------------------------------------+ | What does the *normal* status of an electrical switch refer to? | | Specifically, what is the difference between a *normally-open* switch | | and a *normally-closed* switch? | | | | The "normal" status of a switch refers to the open or closed status | | of the contacts when there is no actuating force applied to the | | switch. | +-----------------------------------------------------------------------+ Normally-Open, Timed-Closed First we have the normally-open, timed-closed (NOTC) contact. This type of contact is normally open when the coil is unpowered (de-energized). The contact is closed by the application of power to the relay coil, but only after the coil has been continuously powered for the specified amount of time. In other words, the direction of the contact\'s motion (either to close or to open) is identical to a regular NO contact, but there is a delay in closing direction. Because the delay occurs in the direction of coil energization, this type of contact is alternatively known as a normally-open, on-delay: The following is a timing diagram of this relay contact\'s operation: Normally-Open, Timed-Open The normally-open, timed-open (NOTO) contact. Like the NOTC contact, this type of contact is normally open when the coil is unpowered (de-energized) and closed by the application of power to the relay coil. However, unlike the NOTC contact, the timing action occurs upon de-energization of the coil rather than upon energization. Because the delay occurs in the direction of coil de-energization, this type of contact is alternatively known as a normally-open, off delay: The following is a timing diagram of this relay contact\'s operation: Normally-Closed, Timed-Open The normally-closed, timed-open (NCTO) contact. This type of contact is normally closed when the coil is unpowered (de-energized). The contact is opened with the application of power to the relay coil, but only after the coil has been continuously powered for the specified amount of time. In other words, the direction of the contact\'s motion (either to close or to open) is identical to a regular NC contact, but there is a delay in the opening direction. Because the delay occurs in the dire