Introduction to visual pathways and circuits 2024.pptx
Document Details
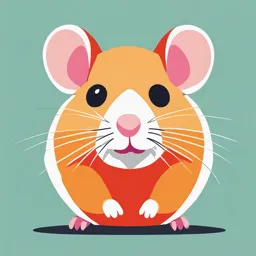
Uploaded by EnthusiasticChrysoprase4588
2024
Tags
Full Transcript
Introduction to visual pathways and circuits From light to sensation Prof. Evelyne Sernagor Biosciences Institute BMS2011 Lecture 10 9 February 2024 © MPI f Biological cybernetics ...
Introduction to visual pathways and circuits From light to sensation Prof. Evelyne Sernagor Biosciences Institute BMS2011 Lecture 10 9 February 2024 © MPI f Biological cybernetics Vision: the transduction of visible light into neural signals, creating the perception of ‘what’ is ‘where.’ 2001 Lippincott, Williams, & Wilkins Electromagnetic waves spectrum Where? Spatial location c V2 V1 What? Object identification Our visual system is sensitive to the visible light range of the electromagnetic wave spectrum, from 380 nm (purple) to 740 nm (red) EM Wave 740 nm 380 nm Why are we, humans, specifically sensitive to this range of the EM wave spectrum? - Most EM energy emitted by the sun is within the visible range - Our visual system is optimally tuned to receive the right amount of information emitted by the sun - Our perception of the world is thus filtered - Other animal species see the world through a different filter - Bees see the world through UV filter, optimised to their own environmental niche. - Central flower pattern not seen under normal visible light. - Helps bees find the nectar rich area. daylight UV light Vision starts in the eye Retina Ciliary muscle Coroid iris Lens Pupi l Fovea Anterior Vitreous humor chamber Optic nerve Suspensory Cornea ligament Brain Optic disc Zonule fibers Sclera The retina Outpost of the brain, part of the CNS Complex, layered structure Outer nuclear layer (ONL) Seven cell types, organised in three Photoreceptors: rods and cones nuclear layers, two synaptic layers Inner nuclear layer (INL) Horizontal cells, bipolar cells, amacrine cells Ganglion cell layer (GCL) ganglion cells (output cells, axons form the optic nerve) Glial cells: Müller cells Synaptic layers Outer plexiform layer (OPL) Synaptic connections between photoreceptors, horizontal and bipolar cells Inner plexiform layer (IPL) Synaptic connections between bipolar, amacrine and ganglion cells. IPL divided into On and Off layer. Our visual system operates over a very wide range of light levels (up to 1016). rods: -6 to 1 log cd/m2 (darkness → moonlight → candlelight) cones: -3 to 10 log cd/m2 (starlight → bright sunlight) Phototransduction Performed in rods and cones outer segments Central vision Peripheral vision Peripheral versus central vision Peripheral vision -Photoreceptors: rods (120 millions), distributed throughout the retina -Rods are responsible for our ability to see in dim light (scotopic vision) -High sensitivity (can detect one photon) -Scotopic vision is monochromatic (colour-blind) -Low resolution images (poor spatial acuity) -Motion detection Central vision -Photoreceptors: cones (6 millions), concentrated around the fovea -Works only in daylight (photopic vision) -Low sensitivity (but work over much broader range of luminance) -Photopic vision is chromatic (colour vision), spectrally tuned. -High spatial acuity -Narrow angle of coverage Normal rod and cone vision Central retina affected Peripheral retina affected Spectral sensitivity for cones and rods in the primate retina The overall balance of activity in S, M and L cones 3 types of cones – trichromacy determines our perception of colour What happens next? Electrical responses in photoreceptors trigger responses in second order neurones (bipolar and horizontal cells) via glutamatergic neurotransmission, eventually leading to responses in ganglion cells, the output cells of the retina Ganglion cell receptive fields - The brain needs to reconstruct visual scenes - The best way to do it is to use many detectors, each dedicated to a small portion of the visual scene - Each part of the scene is detected by a number of photoreceptors - The part of the visual field an RGC gathers information from is called the cell’s receptive field On and OFF centre receptive fields RGCs do not respond much to diffuse light (photoreceptors do) They respond best to contrast (small spot of light, small ring of light, light-dark edge). They have an antagonistic center- surround receptive field – the center must be mainly light and the surround mainly dark, or vice versa. Receptive field sizes increase with retinal eccentricity Periphery Large RF Fovea Small RF (more details) RGCs in primates Midget (parvocellular - P, 80-90% of RGCs) Parasol (magnocellular - M, 10-20% of RGCs) “K” cells (koniocellular-projecting; small bistratified) Many other (>30) RGC types Functional differences between midget, parasol and K RGCs: Midget and “K” cells are basis for ‘what’ pathway: Form, colour Parasol cells are basis for ‘where’ pathway: Motion, distance The division between these three pathways becomes anatomically evident in the lateral geniculate nucleus (LGN) of the thalamus. Opponent chromatic organisation in primate midget RGC receptive fields -S+(L+M) +S-(L+M) L+ M+ Yellow on, blue off Red on, green off Blue on, yellow off Green on, red off Established through specific synaptic connections between cones, horizontal and bipolar cells Blue-yellow opponent retinal pathway S cones (blue) connect to S-cone bipolar cells (blue). L and M cones connect to other bipolar cells (yellow). B/Y bistratified ganglion cell: excitatory input from S cone bipolars inhibitory input from L+M cone bipolars cell Experiencing colour opponency The retinal code Phototransduction (in rod/cone outer segments) Spikes trains CODE processing action electrical potentials responses in RGCs Brain visual areas The visual and non-visual pathways from the retina retina thalamus primar extrastria lateral geniculate y te nucleus (dLGN) (dorsal) visual visual cortex cortical retinotectal tract areas superior colliculus eye movements pretectum (6 nuclei) pupillary reflexes retinohypothalamic tract Hypothalamus (suprachiasmatic nucleus (SCN circadian rhythm entrainment Left Visual Field Right Visual Field nasal Temporal Temporal Projections from the retina to central targets are organised in a topographic fashion Neighbouring parts of the retina project to neighbouring regions in their central targets (SC, LGN and visual cortex). There is a map of the field of view within the central target The retinal foveal area is magnified in central targets Topographic order is maintained but the central visual field is magnified Lateral geniculate nucleus Koniocellular layers Division between M Alternating layers (parasol RGCs), P from ipsi and (midget RGCs) and contralateral eye i: ipsilateral c: contralateral K pathways Koniocellular layers: input from small bistratified B/Y RGCs LGN receptive fields Centre-surround, like in RGCs So what is the role of the LGN? 1.The LGN brings retinotopic maps from both eyes into register to make it easy for the cortex to combine inputs from the two eyes. 2. Only 10% of inputs to the LGN come from the retina, hence it has many other functions (inputs from brainstem and cortex), not well understood. The primary visual cortex, V1 Input to striate cortex Broadman area Stria (line) of Gennari (band of myelinated axons (from LGN cells) running parallel to the surface of the cortex along the calcarine fissure of the occipital lobe 6 layers, main input from LGN to layer IV The striate cortex is organised in ocular dominance columns, alternating between left and right eye Cells are monocular in layer 4 They are binocular in other layers The functional organisation of V1 Colour processing areas (colour blobs, input from koniocellular layers from LGN) Monocular cells Orientation preference columns Ocular dominance columns hypercolumn: one set of OD + OS columns V1 receptive fields Simple cells Hubel and Wiesel model - Sum inputs from LGN neurons with neighbouring/aligned RFs to build an elongated RF that is most responsive to elongated bars or edges - Orientation selective - Monocular or binocular - Have separate ON and OFF subregions - Perform length summation (bigger responses with increasing bar length until it reaches a plateau) V1 receptive fields Complex and hypercomplex cells Complex cells - Receive input from simple cells - Orientation selective - Spatially homogeneous RFs (no separate ON/OFF subregions) - Nearly all binocular - Perform length summation Hypercomplex cells - Like complex cells with inhibitory flanks on the ends of the receptive field - Response increases with increasing bar length up to some limit - As the bar becomes longer, the response is inhibited (end-stopping) Simple, complex and hypercomplex cells: summary Simple cells Narrow, elongated excitatory and inhibitory zones (ON-OFF) with specific axis of orientation “Line detectors” RFs built from convergent connections of LGN cells Complex cells Large RFs without clear excitatory or inhibitory zones Respond best to moving edge @ specific orientation or direction of motion “Motion detectors” RFs built from the convergent connections of simple cells Hypercomplex cells Very large RFs combining signals from complex cells Respond best to oriented edge that are stopped (no response beyond a specific part of the RF) “angle detectors” Simple, complex and hypercomplex cells: Can work together to decompose the outlines of a visual image into short segments This is the basis of simple and complex object recognition Ventral stream: ‘WHAT’ V1 ventral prestriate cortex inferotemporal cortex Ventral stream Colour & shape Scene understanding & object recognition Long-term representations “Conscious perception” Dorsal stream: ‘WHERE’ V1 dorsal prestriate cortex posterior parietal cortex Motion and coarse depth Dorsal stream Visual control of motor output Moment-to-moment computations Ventral stream “Unconscious control of behaviour”