Introduction To Hydrogeology PDF
Document Details
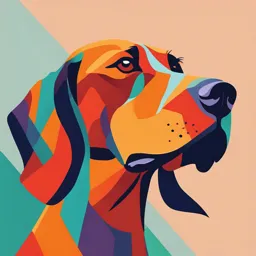
Uploaded by DeservingPinkTourmaline2530
Savitribai Phule Pune University
2003
Johannes C. Nonner
Tags
Summary
This textbook provides an introduction to hydrogeology, a science that explores the occurrence, distribution, movement, and quality of groundwater. It adopts a systems approach, examining the interrelationships within hydrological systems, including the atmosphere, surface water, and unsaturated zone. The book covers topics like groundwater flow, balances, chemistry, and development.
Full Transcript
INTRODUCTION TO HYDROGEOLOGY © 2003 Swets & Zeitlinger B.V., Lisse IHE DELFT LECTURE NOTE SERIES © 2003 Swets & Zeitlinger B.V., Lisse Introduction to Hydrogeology JOHANNES C. NONNER A.A. BALKEMA PUBLISHERS / LISSE / ABINGDON / EXTON (PA) / TOKYO © 2003 Swets & Zeitlinger B.V., Lisse Library...
INTRODUCTION TO HYDROGEOLOGY © 2003 Swets & Zeitlinger B.V., Lisse IHE DELFT LECTURE NOTE SERIES © 2003 Swets & Zeitlinger B.V., Lisse Introduction to Hydrogeology JOHANNES C. NONNER A.A. BALKEMA PUBLISHERS / LISSE / ABINGDON / EXTON (PA) / TOKYO © 2003 Swets & Zeitlinger B.V., Lisse Library of Congress Cataloging-in-Publication Data Nonner, J.C. 1948- Introduction to hydrogeology / J.C. Nonner p. cm. – (The Delft lecture note series) Includes bibliographical references (p. ). ISBN 9026518692 (hardbound) -- ISBN 9026518706 (pbk.) 1. Hydrogeology. I. Title. II. Series. GB1003.2.N65 2002 551.49--dc21 2001056547 © 2003 Swets & Zeitlinger B.V., Lisse All rights reserved. No part of this publication may be reproduced, stored in a retrieval system, or transmitted in any form or by any means, electronic, mechanical, by photocopying, recording or otherwise, without the prior written permission of the publishers. ISBN 90 265 1869 2 (hardbound edition) ISBN 90 265 1930 3 (paperback edition) © 2003 Swets & Zeitlinger B.V., Lisse Contents FOREWORD VII 1 INTRODUCTION 1 1.1 General background 1 1.1.1 The scope of the science 1 1.1.2 The application of hydrogeology 2 1.1.3 Historical background of the science 5 1.1.4 History of groundwater use 8 1.2 Hydrological systems 10 1.2.1 The concept of hydrological systems 10 1.2.2 Water at land surface 15 1.2.3 Surface water systems 21 1.2.4 The unsaturated zone 24 1.2.5 Groundwater systems 28 2 GROUNDWATER CONCEPTS 37 2.1 Basic concepts 37 2.1.1 Physical properties of water and rock 37 2.1.2 Groundwater system terminology 44 2.2 Formation of groundwater systems 50 2.2.1 Groundwater systems in metamorphic and intrusive rock 50 2.2.2 Development of aquifers in volcanic rock 54 2.2.3 Aquifer formation in consolidated sediments 57 2.2.4 Unconsolidated sediments and groundwater systems 64 3 GROUNDWATER MOVEMENT 69 3.1 Principles of groundwater flow 69 3.1.1 Groundwater flow in unconsolidated rock 69 3.1.2 Groundwater flow in consolidated rock 83 3.2 Flow in groundwater systems 87 3.2.1 Basic concepts 87 3.2.2 Regional groundwater flow 91 3.2.3 Local groundwater flow 112 © 2003 Swets & Zeitlinger B.V., Lisse VI Introduction to Hydrogeology 3.3 The flow systems concept 117 3.3.1 Formation of flow systems 117 3.3.2 Flow computations 120 4 GROUNDWATER BALANCES 123 4.1 Groundwater balance concepts 123 4.1.1 The general concept 123 4.1.2 Practical application of groundwater balances 128 4.2 Estimating the groundwater balance 130 4.2.1 Basic concepts and recharge 130 4.2.2 Discharge and storage 138 4.2.3 Groundwater balances using flow computations and modelling 141 4.3 Groundwater balances and their environment 143 4.3.1 Groundwater recharge and climate 143 4.3.2 Groundwater balances and geology 148 5 GROUNDWATER CHEMISTRY 157 5.1 Groundwater and chemical processes 157 5.1.1 Chemical properties of water and rock 157 5.1.2 Development of groundwater chemistry 168 5.2 Groundwater chemistry and rock types 176 5.2.1 Groundwater chemistry in consolidated rocks 176 5.2.2 Groundwater chemistry in unconsolidated rocks 180 6 GROUNDWATER DEVELOPMENT 183 6.1 Groundwater management 183 6.1.1 Water management 183 6.1.2 The role of groundwater 186 6.2 Groundwater planning and investigations 188 6.2.1 Water planning 188 6.2.2 Investigation methods 190 6.2.3 Groundwater monitoring 205 6.3 Groundwater resources studies 205 6.3.1 Regional groundwater availability 205 6.3.2 Groundwater demand 214 6.3.3 Local groundwater development 217 EXERCISES 225 SELECTED ANSWERS TO EXERCICES 239 REFERENCES AND BIBLIOGRAPHY 241 © 2003 Swets & Zeitlinger B.V., Lisse Foreword The Hydrology and Water Resources training programme offered at the International Institute for Infrastructural and Environmental Engi- neering (IHE) in The Netherlands also specialises in groundwater. As a contributor to this programme I had the task to collect instruction material on groundwater which could be used for the training of parti- cipants. Although excellent textbooks on groundwater are available, I could not swiftly identify an introductory textbook on this subject and therefore decided to prepare ‘Lecture Notes’. These notes had been used in groundwater classes until the opportunity arose, in 1998, to convert the lecture material into a textbook. The present ‘Introduction to Hydrogeology’ is the result of this conversion. This opportunity and my wish to assist in passing on current knowledge on groundwater have motivated me to make the time available to compile this text- book. In this textbook a systems approach is followed. This means that the groundwater system has been considered as the framework for the compilation of this Introduction. In Chapter 1, following a histo- rical introduction, the groundwater system is placed within the earth hydrological cycle. Brief introductions are given to the other hydro- logical systems including the atmospheric system, the surface water system and the unsaturated zone. Chapter 2 describes basic properties of ‘water and rock’ in the groundwater system and introduces ‘hydro- geological classifications’. The chapter concludes with an outline on the occurrence of groundwater in various rock types. Chapter 3 is devoted to groundwater flow. In this chapter the emphasis is on the evaluation of regional flow in groundwater systems, rather than on local groundwater in the vicinity of infrastructural works. In Chapter 4, groundwater balances are discussed in general terms, but also in relation to the various rock types and climatological condi- tions on earth. Chapter 5 focuses on groundwater chemistry. The main processes are discussed and the chemistry of groundwater in selected rock types is evaluated. The final Chapter 6 has the title ‘Groundwater Development’. Practical topics are dealt with in this text and the chap- ter includes an extensive outline on field investigations and guidelines © 2003 Swets & Zeitlinger B.V., Lisse VIII Introduction to Hydrogeology for the determination of groundwater availability using a groundwa- ter balance approach. For personal training in various apects of the ‘science of hydrogeology’, a set of groundwater problems has been added. Finally, I would like to thank all persons who have, in some way or another, contributed to this textbook. In the first place I owe a lot to my parents for giving me the opportunity to undertake my studies in Utrecht and Amsterdam, and to my wife and children for their moral support. I would also like to thank Prof. Dr. Ir. J.C. van Dam, Prof. Dr. J.J de Vries, Dr. C.A.J. Appelo and Dr. P.J.M. de Laat for their critical reading of the draft versions. Their comments and suggestions have been very helpful. Finally, I would like to express my gratitude to Hans Emeis for his assistance in the preparation of the drawings, and to staff and participants of IHE who have also made a contribution to this textbook. Johannes C. Nonner © 2003 Swets & Zeitlinger B.V., Lisse CHAPTER 1 Introduction 1.1 GENERAL BACKGROUND 1.1.1 The scope of the science Definition of Hydrogeology can be considered as one of the earth-sciences that has hydrogeology gained in popularity in recent years. Occasionally there has been con- fusion with respect to the precise definition of hydrogeology. Although the term hydrogeology was already used in the 19th century, it was only at the start of the 20th century that the scientist Mead (1919) gave the term a wider meaning. He defined hydrogeology as ‘the study of the occurrence and movement of subterranean waters’. Meinzer (1923) used the term geohydrology to describe in principle the same physical process. The basic definition of hydrogeology as formulated by Mead is a very useful definition. In this textbook, however, a slightly different formulation will be used. Hydrogeology is ‘the study of the occurrence, movement and chemistry of groundwater in its geological environ- ment’. This definition emphasises the role that the science of geology plays in the study of groundwater. A sound knowledge of the geology of an area should form the basis for all hydrogeological assessments. Relation with other Hydrogeology is related to other sciences, as shown in Figure 1.1. sciences There are first relations with basic sciences like mathematics, phys- ics, and chemistry. Knowledge of these subjects is indispensable for a full understanding of hydrogeology. Other earth sciences that need to be mastered by the hydrogeologist are geology, surface water hydrol- ogy and meteorology. It should be said, however, that no scientist can master all these subjects in detail and therefore much of the research done in the field of hydrogeology has been accomplished by joint efforts between hydrogeologists, geologists, hydrologists, and meteor- ologists. Worth mentioning is also the relation between hydrogeology and engineering. Hydrogeology plays an important role in the design work that engineers undertake for the benefit and welfare of mankind. The hydrogeologist can supply information on groundwater tables, © 2003 Swets & Zeitlinger B.V., Lisse 2 Introduction to Hydrogeology mathematics geology water engineering hydraulic engineering physics Hydrogeology drainage engineering environmental Figure 1.1. Hydrogeology and chemistry hydrology engineering its relation with other sciences. available groundwater resources and water quality aspects that the engineer wants to know for the design of, for example, public and domestic water supplies, irrigation schemes, flood protection works, and even office and industrial buildings. 1.1.2 The application of hydrogeology Engineering applications The science of hydrogeology is applied in various fields of engi- neering. The most important fields of civil and agricultural engineer- ing using hydrogeological assessments can be summarised as fol- lows: – Water engineering; i.e. the field of engineering that deals with the supply of water for domestic and industrial use, and irrigation. – Hydraulic engineering; i.e. the engineering that occupies itself with the construction of water works. – Drainage engineering; i.e. the engineering branch that is involved in the drainage of lowland areas and the dewatering of mines. – Environmental engineering; i.e. the engineering that deals with the conservation of the natural environment and the creation of new nature. Water engineering For the design of water engineering works including water wells and well fields, hydrogeological assessments are needed. Wells supply groundwater to user areas to satisfy water demand. In recent decennia, the use and demand have increased considerably. The trend is visible in many countries; in the developing as well as in the developed world. For example, in the United States groundwater consumption was grow- ing at an even faster rate than the population. This is illustrated in Fig- ure 1.2 showing that the groundwater supplies in urban areas, and for irrigation were largely responsible for the fast growth of groundwater consumption in recent times. © 2003 Swets & Zeitlinger B.V., Lisse Introduction 3 water volume or population number irrigation urban water population Figure 1.2. Increase in ground- water consumption versus the increase in population. time It is not surprising that groundwater supplies in urban areas and for irrigation have increased so rapidly. The trend that people move away from rural areas and settle in densely populated urban areas partly explains the increase in water use as delivered by groundwater supply schemes. Also, the increased use of water for personal hygiene (showers, washing machines) and the use of water for the sprinkling of lawns and gardens has put a strain on the water supply schemes in urban areas. In the agricultural sector farmers are increasingly devel- oping areas where crops heavily depend on irrigation from ground- water. In many of the developing countries one can observe similar trends in groundwater consumption. Also in these countries there is a grow- ing urbanisation and awareness of personal hygiene that has lead to additional claims on available groundwater resources. Since it is well known that there is a rapid increase in groundwater irrigation schemes in large parts of the developing world, similar claims on groundwater can be expected from the irrigation sector. This phenomenon is not only visible in arid countries, but also in the humid tropics where irri- gation schemes are implemented to secure the harvest of two or three crops per year. Hydraulic engineering Water works or water- associated works that are designed by hydraulic and civil engineers include diversion weirs in rivers, dams at reser- voirs, dikes and embankments along rivers, road supports and founda- tions for buildings. Taking into account the increasing complexity of these structures and, on the other hand, the tendency to minimise the risk of failure one should be aware that hydrogeology plays an increas- ingly important role in this field of engineering. For example, the spa- tial distribution and fluctuations in groundwater tables in an area are © 2003 Swets & Zeitlinger B.V., Lisse 4 Introduction to Hydrogeology determining factors for the stability computations carried out for the design of water works. Drainage engineering Dewatering and drainage infrastructures are developed by engineering geologists, mining experts, and agricultural engineers. For engineering geologists and mining experts hydrogeological assessments are valu- able when they compute pump capacity to be installed in building pits, and open cast or underground mines. Positioning of these pumps at the correct locations in the pits or mines is essential. Knowledge of hydrogeology is also crucial to the agricultural engineer who wishes to install horizontal drainage systems or vertical drainage schemes con- sisting of wells. For example, in The Netherlands the horizontal drain- age system in the low-lying part of the country (‘the polders’) has been designed on the basis of a thorough hydrogeological study. The system that has been installed is capable of draining away the excess shallow flow of groundwater, while largely preventing the upconing of brack- ish water. Environmental Environmental engineering concerns the setting up of measures and engineering the organisation of operations to prevent and restore adverse effects on groundwater. In particular in the past decennium, there has been full agreement amongst scientists that groundwater is a precious resource which has to be carefully assessed and managed. Not only has this been the case in the minds of scientists, but also in the minds of a much wider public. There are two principal causes for environmental concern related to groundwater resources. First, there is the concern for the decline in groundwater tables and depletion of resources as a result of water engineering activities including the installation of wells and well fields. Secondly, there is the con- cern for a deterioration in groundwater quality caused by human negligence at waste disposal sites, the use of toxic chemicals in agriculture, salt water intrusion or, simply, by a lack of sanitary pro- tection. Increasing role of One conclusion that can be drawn from the above is that the science of hydrogeology hydrogeology is playing a central role in many engineering disciplines. However, it will be clear that not only hydrogeologists and engineers focus on hydrogeology. Increasingly so, other professionals includ- ing ‘politicians and other decision makers’, environmentalists, biolo- gists and even economists have to pay attention to groundwater issues. Proper training in hydrogeological assessments is also an essential part of the game. Figure 1.3 presents a photo showing a group of students to be trained in taking observations at a rock outcrop and conducting geological measurements. Groundwater can not be taken for granted anymore. People will have to pay a larger price for the proper manage- © 2003 Swets & Zeitlinger B.V., Lisse Introduction 5 Figure 1.3. Hydrogeological assessments often start with rock observations. Here, students are inspecting slates and quartz- ites, dissected by joints and fractures. ment and rehabilitation of groundwater resources in the foreseeable future. 1.1.3 Historical background of the science Development of the In the old Greek and Roman civilisations philosophers and scien- science tists have speculated about ‘underground waters’. They were puzzled by springs, and the discharges of water in rivers in dry periods, long after precipitation had stopped. The Greek Plato (427-347 B.C.) and other philosophers offered a solution. They thought that ground- water originated from a cavern which was connected to the ocean. By the action of waves, water of the sea was transported from the ocean upward into caverns and from there to springs and rivers. They assumed that underground purification filtered away the salts in seawater, in order to explain that spring water and river water are fresh. The true explanation for the occurrence of springs and discharges in rivers during dry periods was put forward by the French scientists Per- rault (1608-1680) and Mariotte (1620-1684). Perrault suggested that © 2003 Swets & Zeitlinger B.V., Lisse 6 Introduction to Hydrogeology the amount of precipitation in the Seine catchment in France could eas- ily account for all the discharge in the river Seine. Mariotte carried out infiltration experiments in the catchment and discharge measurements in the Seine. He found that precipitation could indeed infiltrate into the ground and underlying rocks in appreciable quantities. Mariotte concluded that these rocks, acting as a storage medium, could sustain springs and a year around discharge in the Seine. In the 19th century another development drew wide attention from other scientists in the field of hydrogeology. The French water engi- neer Darcy (1803-1858) made a start with groundwater hydraulics (Darcy, 1856). He did experiments with sand filters and formulated his well-known formula to compute the ‘flow rate’ of groundwater in porous rocks. Flow formulae and Since the 19th century many scientists including the mentioned Mead modelling and Meinzer (see section 1.1.1) have made respectful contributions to the science of hydrogeology. Modern trends in the science of hydro- geology concern the development of flow formulae and modelling, the introduction of flow systems analysis, and the focus on hydrochemistry and groundwater contamination. Flow formulae based on ‘Darcy’s Law’ have been developed. Sci- entists including Thiem (1906), Theis (1935) and Jacob (1950) devel- oped formulae for radial flow to wells. The formulae concern the flow of fresh groundwater with constant density. The range of formulae includes expressions describing the flow to wells in various natural environments. Flow formulae for open- and closed groundwater sys- tems, for ‘soft and hard’ rocks and for steady and non-steady condi- tions were developed. Other types of formulae were also developed, for example, those dealing with the flow of groundwater in an environment where water density varies from place to place. In this respect one may think of a phenomenon that can be observed in many coastal areas: the flow of groundwater in fresh water lenses which overlay saline groundwater. Scientists including Van Dam (1983) developed equations for the flow in these fresh water lenses and the position of the fresh-saline inter- face taking into account various stresses acting on the groundwater system. The scientific efforts to develop appropriate formulae for the flow to wells and for the flow in areas with a varying water density were initially based on an analytical approach; i.e. on the application of analytical methods to solve the general groundwater flow equations. From about 1960 less attention has been paid to the development of analytical methods. Models based on numerical methods became rap- idly popular and they form the basis for most of the flow computa- tions presently carried out. Compared with the flow equations based on © 2003 Swets & Zeitlinger B.V., Lisse Introduction 7 analytical methods, models are much better suited to describe ground- water flow in areas where the groundwater system is of a complex nature. Also through the introduction of personal computers, the use of models increased quickly. The ability to process the computations fast is a most essential asset of the modern brand of computers. Figure 1.4, for example, presents an application of a modern flow model. The diagrams show the model-calculated paths of groundwater flow between galleries for artificial recharge and a well field in the complex sandy groundwater system in the eastern part of The Neth- erlands. The model was built to assess the scope for the restoration of groundwater tables in a sensitive area using artificial recharge sys- tems. Flow systems analysis Another recent development in the science of hydrogeology has been the introduction of the flow system concept. Traditionally hydrogeo- logical classifications were mainly based on the rock types in a par- ticular area. In the flow system concept as formulated by Toth (1962), classifications were suggested which are based, not only on rock types, Figure 1.4. Computer printout showing flowlines from artifi- cial recharge galleries to pump- ing wells in the complex sandy groundwater system in the de Pol area, Doetinchem, The Netherlands. Case A: two gal- leries operational, case B: five galleries operational (Abush- aar, 1997). © 2003 Swets & Zeitlinger B.V., Lisse 8 Introduction to Hydrogeology but also take into account groundwater flow distributions. For exam- ple, this concept has been applied in The Netherlands whereby an atlas has been prepared showing the flow systems in various parts of the country. The flow system concept provides a flexible approach towards groundwater analyses; in particular for areas where human interfer- ences in the groundwater system have to be studied. Groundwater chemistry Other recent developments in the science of hydrogeology include and groundwater the work recently done on groundwater chemistry and groundwater contamination contamination. In the field of groundwater chemistry many scientists including Appelo and Postma (1996) have paid attention to the inter- relationship between the chemical constituents dissolved in groundwa- ter and the chemical composition of the associated rock types. Work leading to a classification of groundwater in terms of ‘water types’ was also initiated and developed by Stuyfzand (1999). Research has also increased in the field of groundwater tracing in fractured and karstic rock areas. In this field, the behaviour of chemical components is stud- ied with the objective to assess the flowpaths of groundwater through rocks such as basalts, rhyolites, chalks, limestones and dolomite. As a result of the growing concern of groundwater contamination much work has been done in analysing solute transport in groundwa- ter. Bear and Verruijt (1987), and Kinzelbach (1986) are amongst the scientists who gave impetus to these developments. The phenomenon of dispersion takes a central place in their research, while physico- chemical processes such as adsorption and the decay of chemical com- ponents has also been considered. Perhaps the most recent topic is the coupling of physical and physico-chemical processes in solute trans- port modelling. 1.1.4 History of groundwater use The early history Since the early days of humanity people have used groundwater for domestic consumption and for irrigation. Springs were utilised for these purposes, but also wells were constructed to tap groundwater resources. Centuries ago, well construction practices were first carried out during the old civilisations in China, in the Middle East and in Egypt. Due to the scarcity of water in these areas a lot of effort was applied in constructing these wells which in some cases reached amaz- ing depths. Large diameter wells were often dug to depths over 100 m using primitive equipment. Drilled wells that were installed with augering equipment could reach depths even in excess of 500 m. An amazing achievement in groundwater development concerned the construction of so-called ‘khanats’ that were first installed in Iran more than 2500 years ago. Later on, the art of constructing khanats was also practised in Afghanistan and in Egypt. Figure 1.5 shows a © 2003 Swets & Zeitlinger B.V., Lisse Introduction 9 Figure 1.5. Sketch of a khanat system from Iran. rough sketch of a khanat system. Khanat systems are composed of series of large diameter wells that were dug into sedimentary rocks and alluvial fans. The wells were connected by horizontal galleries (tun- nels) reinforced by natural rock. Khanat systems tap the groundwater table and the intercepted water was, and still is, used for domestic sup- plies and small irrigation schemes. Recent developments Since the 12th century, the construction of wells to provide ground- water became a popular activity in Europe. In particular the suc- cessful installation of a number of free flowing artesian wells in Belgium, England and Italy stimulated the use of drilling techniques to construct wells to guarantee domestic and irrigation water for the local population. Traditional auger drilling and percussion drilling techniques were upgraded. At the end of the 19th century an important break-through was the development of the rotary drilling technique. In rotary drilling which was found to be much faster than conventional drilling, drillers for the first time used heavy bentonite mud to guar- antee hole stability during drilling. The installation of temporary cas- ing was not longer necessary. In the 20th century well drilling tech- niques have been further developed. Reverse rotary drilling methods, © 2003 Swets & Zeitlinger B.V., Lisse 10 Introduction to Hydrogeology air-rotary percussion methods, and geophysical well logging tech- niques were introduced. 1.2 HYDROLOGICAL SYSTEMS 1.2.1 The concept of hydrological systems Oceans, atmosphere and Hydrogeology is defined as the study of the ‘occurrence, movement continents and chemistry of groundwater in its geological environment’ (see sec- tion 1.1). When one studies hydrogeology one has to pay attention to the processes that play a role in the oceans, in the atmosphere and at the continents of the world. There is a rather indirect, but nevertheless significant, relationship with the oceans and the atmosphere. Obvi- ously the science of hydrogeology is directly related to the continents. The occurrence and movement of groundwater very much depends on the structure of the rocks contained in the continents and the morpho- logical and climatic conditions occurring at or near the surface of the continents. One can take a brief look at the properties of the oceans, atmos- phere and continents respectively. Table 1.1 shows that the oceans and seas have a surface area of 361*106 km2, making up about 75% of the global surface. The other part is occupied by the landmass of the five continents. The largest part of the oceans is concentrated south of the equator. The depths of the oceans varies from less than 10 m in peripheral seas to over 11000 m in longitudinal troughs which are mainly located in the Pacific Ocean. The total volume of water in the oceans and seas amounts to about 1.4*109 km3 which is by far the largest water volume on the earth. The atmosphere can be subdivided into various atmospheric layers. Most of the atmospheric water is contained in the bottom layer: the troposphere. The temperature in the troposphere, which has a thick- ness of about 12 km, decreases with an increase in height. The layer above the troposphere is called the stratosphere. Surprisingly, in this Table 1.1. Main world water quantities (Lvovich, 1979). Item Area Volume Percentage (106 km2) (106 km3) (%) Oceans and seas 361 1370 94.2 Icecaps and glaciers 16.2 24 1.65 Atmospheric water 504 0.01 < 0.01 Lakes and reservoirs 1.55 0.13 < 0.01 River channels < 0.1 < 0.01 < 0.01 Groundwater 130 60 4.13 © 2003 Swets & Zeitlinger B.V., Lisse Introduction 11 atmospheric layer the temperature tends to increase with height. Nearly all the atmospheric water is present in the form of gaseous water vapour. Table 1.1 gives an idea of the amount of water vapour in the atmosphere. The computed equivalent amount of water amounts to only 0.01*106 km3. It can be concluded from the table that the amount of water in the atmosphere is very small as compared with the amounts of water ‘stored’ in the oceans and seas, and on the continent. At continents features of interest can be observed at land surface. These features include lakes, reservoirs, streams and rivers. Their pres- ence and their size depend on the climate that prevails in the area in which one is interested. For example, the rivers with the highest dis- charge can be expected in tropical areas where precipitation is highest. No rivers at all or rivers that only flow intermittently are typical for arid areas where precipitation is not abundant. The amount of water stored in lakes, reservoirs, streams and rivers is comparatively small and amounts to a mere 0.13*106 km3. Features below land surface include ‘hard and soft’ rocks, molten magma, and liquid material. For a general orientation one may first have a look at Figure 1.6, presenting the various interior components of the earth. The centre space of the earth is occupied by the so-called ‘earth core’ which consists of iron and nickel compounds. The ‘mantle’ with a thickness of about 3000 km surrounds the core of the earth and is made up of ultra-basic rocks with a density in the order of 3000 to 4000 kg/m3. The earth ‘crust’ is the outermost shell of the earth. With its thickness varying between 12 km below the oceans to 35 km below the continents the rock density in the crust varies between 2500 and 3000 kg/m3. The upper part of the crust at the continents contains zones with water that is generally referred to as groundwater. The types of rocks contained in the continents give an indication of the thickness of these crust continent core groundwater system Figure 1.6. Cross section mantle through the earth showing groundwater systems at the continents. ocean © 2003 Swets & Zeitlinger B.V., Lisse 12 Introduction to Hydrogeology ‘groundwater systems’ (see section 1.2.5). On the average ground- water systems have a thickness between 10 m and 500 m. However, in some areas including coastal plains groundwater systems may be more than 1000 m thick. Table 1.1 shows that on earth the total volume of water stored in groundwater systems is in the order of 60*106 km3. The table indicates that, disregarding the icecaps and glaciers, most of the fresh water reserves of the earth are present in the form of groundwater. The hydrological cycle The volumes of water contained in the various environments of the earth, oceans, atmosphere and continents, have been described above. As the French hydrogeologists Perrault and Mariotte already found out, this water can be transported from one environment to the other. The general process is presented in Figure 1.7. Water evaporates at the oceans and is transported as water vapour in the atmosphere to the continents. Above the continents the water vapour is transformed into precipitation in the form of rain, hail or snow which fall on the sur- face of the earth. Rain, molten hail and snow may be directed towards lakes, streams or rivers or alternatively infiltrate into the ground, where they recharge groundwater systems. From lakes, streams and rivers and from the groundwater systems the water flows back into the oceans and seas. In the oceans and seas water evaporates again, is transported to the continents, etc. The process described is the transport of water in a cyclic process; it is also referred to as ‘the hydrological cycle’. The process outlined above is a very generalised one and in reality it is far more complex. For example, not all the evaporated water from the oceans will be transported to the continents as water vapour, but part of it will return directly to the ocean in the form of precipitation. Also, not all the rain, hail and snow that falls on the earth surface will infiltrate into the ground or be transported to lakes, streams and rivers. El Pl Es Ps R Figure 1.7. Schematic repre- Q Q sentation of the hydrological cycle. © 2003 Swets & Zeitlinger B.V., Lisse Introduction 13 A substantial portion of this water will evaporate from land surface or the soil. In addition, not all the water that recharges the groundwater system will directly flow back to the seas and oceans. The scientists Perrault and Mariotte already concluded that groundwater may also flow from the groundwater system into a river. Table 1.2 shows the inflows and outflows of water or water vapour associated with the oceans and seas, and with the continents. It can be deduced from Table 1.2 that an amount of water in the order of 0.43*106 km3/year evaporates from the oceans and seas. Less than 10% of this amount, in the order of 0.036*106 km3/year is transported as water vapour to the continents. On the continents itself this water vapour, and the water evaporated from land surface comes down as precipitation to the amount of about 0.11*106 km3/year. The amount of water which flows back from lakes, streams and rivers, and ground- water systems to the oceans and seas is equivalent to the amount of water vapour transported to the continents: 0.036*106 km3/year. Figure 1.8 shows one of the major rivers on earth transporting water back to the ocean. The river Ganges portrayed in the picture collects water in the Himalayas and takes the water to the Indian Ocean. Nowa- days, far lesser amounts of water are flowing through the Ganges to the ocean, as a result of the diversion of river water for domestic use and irrigation schemes. The inflows and outflows as outlined in Table 1.2 can also be seen as the terms of so-called ‘water balances’. One can have a closer look at the concept of water balances. Water balances can be set up for pre- defined environments such as the oceans and seas, the continents and even lake, stream and river systems or groundwater systems. A water balance essentially relates the flows of water into an environment to the flows of water leaving this environment. In a state of equilibrium the inflow of water is equal to the outflow of water. In a state of non-equi- librium the difference between inflow and outflow equals the change in water storage. For equilibrium conditions one can formulate the Table 1.2. Flow volumes of water at the globe (Shiklomanov, 1997). Item Flow Flow (106 km3/year) (mm/year) Oceans and seas Evaporation 0.434 (out) 1202 (out) Precipitation 0.398 (in) 1103 (in) Inflow from groundwater and rivers 0.036 (in) Continents Evaporation 0.071 (out) 476 (out) Precipitation 0.107 (in) 718 (in) Outflow via groundwater and rivers 0.036 (out) (out) © 2003 Swets & Zeitlinger B.V., Lisse 14 Introduction to Hydrogeology Figure 1.8. View of the majestic Ganges River near Rishikesh, India. general water balance for the oceans and seas. Considering the water balance terms listed in Table 1.2 one can set up the following expres- sion for the oceans and seas: Ps + (R + Q) = Es (1.1) For the continents one could formulate: P1 = (R + Q) + E1 (1.2) where: Ps = precipitation at seas and oceans (km3/year) Es = evaporation at seas and oceans (km3/year) © 2003 Swets & Zeitlinger B.V., Lisse Introduction 15 Pl = precipitation at continents (km3/year) El = evaporation at continents (km3/year) (R + Q) = groundwater and river discharge to oceans and seas (km3/year) Is the relationship above correct? One could think of other inflows and outflows of water related to oceans and seas, or to continents. For example, one could mention an inflow of water associated with magma originating from the earth mantle. However, it appears that these inflows are small. Alternatively one could imagine an outflow of water stored in oceans and seas, due to the entrapment of water in the sediments on the ocean floor. However, also these quantities are comparatively small or counter-balanced by other effects. 1.2.2 Water at land surface Precipitation Precipitation is an essential parameter to be considered for quite a number of hydrogeological assessments. It is therefore useful to get acquainted with the processes that cause precipitation. Precipitation including rainfall, hail or snow is formed around small nuclei in those parts of the atmosphere where water vapour reaches its saturation point. Three atmospheric processes including lateral moisture transport, con- vective moisture transport, and transport under the influence of orogenic effects, further stimulate the formation of precipitation. The formation of precipitation caused by these processes can be described as follows: i) In particular in the tropics, precipitation can be enormous when large masses of warm moist air move laterally into regions of colder air. ii) Through convection warm moist air can move upward into higher situated layers of cold air and precipitation will follow. iii) Due to the presence of mountain ranges air rich in water vapour will rise upward and precipitation will follow when the air reaches a colder environment. How does one determine precipitation rates that may vary consider- ably from place to place and from time to time? For the proper col- lection of precipitation data, which are required to assess precipita- tion rates, one needs a relatively dense network of observation points. At these observation points cumulative or continuous measurement of precipitation can be carried out. For the daily or monthly recording of precipitation one can use standard rain gauges or pluviometers, and for continuous measurement of precipitation recorders can be installed. Figure 1.9 shows recently developed instrumentation for the meas- urement of precipitation. A computerised solid state recorder is attached to a rainfall collector. Using a pressure sensor, water levels © 2003 Swets & Zeitlinger B.V., Lisse 16 Introduction to Hydrogeology rain gauge rainfall collector water level housing pressure sensor Figure 1.9. Rainfall collector recorder and recorder with pressure sensor. in the collector relating to the amounts of precipitation collected are stored in a computerised memory chip placed in the recorder. The processing of precipitation data can be carried out in vari- ous ways. For example, so-called ‘isohyetal maps’ can be prepared. Isohyets which are contour lines connecting points of equal depth of precipitation give a good insight in the aerial distribution of this parameter. Figure 1.10 shows an isohyetal map for the catchment area of the Fundres river in the Dolomites in Italy. The increase in daily precipitation rate towards the higher areas is a result of the orogenic effects in the river catchment. In addition, graphs of time series of precipitation rates are very use- ful. These graphs may present time series of average daily, monthly, or yearly precipitation rates. However, graphs may also be prepared showing precipitation frequency and precipitation intensities on an hourly or even shorter time basis. Table 1.3 has been prepared to obtain an impression of the precipi- tation rates for the continents. The data in this table are based on infor- mation supplied by Barry (1969) and Anon. (1975). With the exception of South America and Australia, it can be deduced from the table that the average yearly precipitation on the continents is within close range of each other; in the order of 600 to 700 mm/year. Evapotranspiration Evapotranspiration is another parameter that plays a role in hydrogeo- logical assessments. The term evapotranspiration refers to two proc- © 2003 Swets & Zeitlinger B.V., Lisse Introduction 17 C Rossa C. Magrava * 2930 * 3059 5 Mt Dengo * 2698 1600 C Paolo * 4 2605 Rio di Fundres 3 1083 0 1 km Figure 1.10. Map with isohyets for 3059 elevation in m above sea level the mountainous Valle di Fundres area, Italy (Nonner, 1969). 3 isohyet with precipitation in mm/day Table 1.3. Precipitation, evaporation and runoff by continent. Continent Precipitation Actual evapo- Total runoff (mm/year) transpiration (mm/year) (mm/year) Africa 670 510 160 Asia 610 390 220 Europe 600 360 240 N. America 670 400 270 S. America 1350 860 490 Australia/New Zealand 470 410 60 esses: it refers to evaporation and transpiration. Evaporation is the process whereby solar energy releases water molecules directly from free water surfaces. Water vapour is the result. The process occurs when the water molecules have absorbed sufficient energy to escape from the surface tension that holds them in the liquid or solid state. Transpiration is the process whereby the transformation of water into water vapour takes place through the vegetation at land surface. Water is taken up by the roots of plants and trees, and transported to their leaves. As a result of the physical properties of the vegetation itself © 2003 Swets & Zeitlinger B.V., Lisse 18 Introduction to Hydrogeology and the supply of solar energy the water at the leaves can be freed into the atmosphere as water vapour. Evapotranspiration is controlled by meteorological conditions, the type of vegetation, and by the supply of water. If one looks at the supply of water that can be used for evapotranspiration then one can distinguish four phenomena. First, at oceans, lakes, streams and riv- ers, ponds and pools, the supply of water for evaporation is directly delivered from the open water surface. Secondly, in the vegetational cover above land surface water can be supplied for evaporation that is retained on leaves and branches (intercepted precipitation). Third, water that has accumulated, in the upper part of the unsaturated zone (root zone), after precipitation can also be released by direct evapora- tion or by transpiration through plant roots (see also section 1.2.4). Fourth, groundwater present in the saturated groundwater system can be transported to the root zone by capillary flow. This transport only takes place for shallow groundwater conditions (see section 1.2.5). From the root zone the water is released into the atmosphere by evapo- ration or transpiration. Figure 1.11. Picture of an area with high transpiration rates, also resulting from shallow saturated groundwater, Uden, The Netherlands. © 2003 Swets & Zeitlinger B.V., Lisse Introduction 19 Figure 1.11 shows an example of the influence of capillary flow and the resulting transpiration in the south of The Netherlands. The shallow groundwater is caused by the ‘blockage’ of the flow of groundwater against an impermeable fault. The groundwater transported to the root zones transpires in grassland and forest areas. The supply of water that is available for evapotranspiration may vary from almost zero to flow rates in the order of several millimetres per day. For a given set of meteorological and land use (vegetation type) conditions, evapotranspiration rates reach maximum values if the supply of water is unlimited. This maximum rate is referred to as the ‘potential evapotranspiration rate’. Potential rates will not always be reached if one considers evapotranspiration at land surface. Here, the amount of water available for evapotranspiration largely depends on the availability of water in the vegetational cover and the water content in the root zone. Water at land surface may be limited and this means that evapotranspiration rates do not reach the potential rates. One refers to these real rates as ‘actual evapotranspiration rates’. Can one determine evapotranspiration rates in an efficient way? Three of the best known methods to determine this parameter can be described as follows: – The pan evaporation method. Here, the potential evaporation rate is directly measured as the loss of water from a large circular pan. For example, the United States Class A pan has a diameter of 1.21 m and a height of 0.25 m. – The lysimeter technique. This technique involves the setting up of a cylinder, tank or basin filled with sediment having a typical vegeta- tional cover. In these facilities which may have surface areas ranging from less than 1 m2 to more than 1000 m2, field measurement of water content, precipitation and discharge allow the compilation of water balances. From these water balances actual evapotranspiration rates can be computed. – The energy budget method. This method is based on the measure- ment of meteorological parameters such as solar radiation, number of sunshine hours, humidity, windspeed etc. With formulae devel- oped by Penman (1948) and Makkink and Van der Heemst (1965), potential evapotranspiration rates can be determined. The processing of evapotranspiration data can be rather varied. The data may be presented on maps showing the areal distribution of potential or actual evapotranspiration rates, but even more popular are maps presenting the distribution of so-called ‘precipitation sur- plusses’. These surplusses are the differences between precipitation and potential or actual evapotranspiration rates. Contour lines of equal precipitation surplusses may be drawn on the maps. Graphs showing time series of evapotranspiration rates or precipitation surplusses are © 2003 Swets & Zeitlinger B.V., Lisse 20 Introduction to Hydrogeology also prepared to obtain an idea on the temporary fluctuations in these rates. Table 1.3 also presents the actual evapotranspiration rates for the individual continents on earth. The table shows that these rates are in the order of 360 to 510 mm/year with the exception of South America. On this continent the high average precipitation of 1350 mm/year cor- relates with a high average evapotranspiration rate of 860 mm/year. Perhaps the most striking feature of the table is the fact that most of the precipitation on the continents is lost to evapotranspiration. Only a relatively small portion, in the order of 15% to 40%, flows towards rivers and lakes and recharges groundwater systems. Infiltration and surface Infiltration and surface runoff are also important parameters for hydro- runoff geological analyses. Infiltration can be defined as ‘the portion of the precipitation that enters into the unsaturated zone’ and surface runoff is ‘that part of the precipitation that flows over land surface towards a surface water system’ (see section 1.2.3). The first part of the precipita- tion that reaches land surface is retained in the vegetational cover and evaporates. If precipitation proceeds then water starts entering into the unsaturated zone and the amount of water stored in this zone increases. Recharge and groundwater flow may also be stimulated (see sections 1.2.4 and 1.2.5). However, if precipitation is continuing, then surplus water will start forming ponds and pools and eventually water flows over the land surface towards the surface water system. The amount of surface runoff depends on the duration of precipita- tion, but also on precipitation intensity, the abundance and type of veg- etation, the infiltration capacity of the unsaturated zone, and the slope precipitation intensity surface runoff ground water flow soil moisture storage surface retention Figure 1.12. Distribution of precipitation (rainfall) after having reached land surface. time from start of precipitation © 2003 Swets & Zeitlinger B.V., Lisse Introduction 21 and roughness of the land surface. Figure 1.12 shows a diagram indi- cating that an increase in precipitation intensity decreases the portion of the precipitation that is retained in the vegetational cover (surface retention) and increases the part that is transformed into surface runoff. Sparse vegetation leads to small amounts of retained precipitation and also increases surface runoff. The infiltration capacity of the unsaturated zone has a profound effect on the amount of surface runoff (see section 1.2.3). A steep slope and limited roughness of the terrain decrease the ‘resistance to flow’ and may lead to a large surface runoff. 1.2.3 Surface water systems Lakes, streams and rivers Surface runoff flows to a system of lakes, streams and rivers: the sur- face water system. The interaction between surface runoff, and the slope and the geology of an area determine the ‘shape and pattern’ of the surface water system. In particular the slope of the terrain deter- mines the shape of streams and rivers. For example, in the upstream parts of a river catchment with moderate to steep slopes the shape of a river is relatively straight and the water is ‘fast-flowing’. In the downstream parts where the land surface is usually flat, a winding or so-called ‘meandering’ river will be present and water will be moving at a much slower rate. The prevailing geology in a catchment area largely determines the pattern of the surface water system. In a volcanic area, for example, scattered with volcanoes of more or less circular shape, the system will have a radial pattern. In areas with an alteration of ‘soft’ rocks such as shales and slates and ‘hard’ rocks like sandstones and quartzites the streams and rivers will mainly flow in the ‘soft’ rock and break only in places through the ‘hard’ rock. In such areas the surface water system usually has a rectangular appearance. In areas of a more or less homogeneous rock type the system has a so-called ‘dendritic form’. The pattern resembles the branches and trunk of a tree. Stream and river This type of discharge can be defined as ‘the volume of water pass- discharge ing through a cross section of a stream or river per unit of time’. Sur- face runoff taking place after persistent precipitation may contribute significantly to discharge. In a plot of discharge against time, the so- called ‘hydrograph’, the peaks in the graph represent this contribu- tion. Another major contribution to stream or river discharge is the outflow of groundwater originating from recharge into the saturated groundwater system (see section 1.2.5). This portion of the discharge is also called the ‘baseflow’ of a stream or river. The baseflow portion is represented by the bottom part of the hydrograph. Figure 1.13 shows a small stream emerging from volcanic terrain in the Caribbean. The picture shows the baseflow of the stream originat- © 2003 Swets & Zeitlinger B.V., Lisse 22 Introduction to Hydrogeology Figure 1.13. Officer, inspecting the base flow of a stream in densely vegetated terrain in the eastern part of Grenada. ing from the volcanic groundwater system. In the rainy season the discharge of the stream may increase tremendously and cause flooding in the lower parts of the catchment. The effect of precipitation on surface runoff and groundwater out- flow as components of stream or river discharge can be further illus- trated. For this purpose, one may consider the four diagrams presented in Figure 1.14, showing sketches of precipitation events and the shape of the corresponding hydrographs. © 2003 Swets & Zeitlinger B.V., Lisse Introduction 23 discharge discharge discharge A time B time discharge C time D time channel precipitation Figure 1.14. Hydrographs of groundwater outflow river discharge in response to a precipitation (rain) event. surface runoff i) The precipitation intensity is smaller than the infiltration capac- ity of the unsaturated zone and the volume of infiltrated water is smaller than the volume required to saturate this zone. This means that there is no surface runoff and no increased ouflow from the groundwater system. In this case only the so-called ‘channel pre- cipitation’ adds to the discharge in the stream or river. The typical shape of the hydrograph is presented in Figure 1.14A. ii) The precipitation intensity is smaller than the infiltration capac- ity, but the volume of infiltrated water exceeds the water deficit in the unsaturated zone and recharge to the groundwater system takes place. This increases the groundwater flow in this system and will result in an increased contribution of groundwater outflow to the stream or river. The corresponding shape of the hydrograph is shown in Figure 1.14B. iii) The precipitation intensity is larger than the infiltration capacity, and the volume of infiltrated water is smaller than the water deficit in the unsaturated zone. In this case only channel precipitation and surface runoff add to the discharge. The shape of the hydrograph is shown in Figure 1.14C. iv) The precipitation intensity is larger than the infiltration capacity, and the volume of infiltrated water is larger than the water deficit in the unsaturated zone. Channel precipitation, surface runoff and © 2003 Swets & Zeitlinger B.V., Lisse 24 Introduction to Hydrogeology groundwater outflow all contribute to the increase in stream or river discharge resulting in a hydrograph as shown in Figure 1.14D. Special cases are hydrographs of streams that do not continually show the groundwater outflow part. This usually implies that for part of the year there is no discharge at all. Only during short periods after heavy precipitation there are surface runoff events which are represented as isolated peaks in the hydrographs. These types of hydrographs may point to areas where the infiltration capacities are very low as a result of the impermeable nature of the rocks in the contributing areas. Alter- natively, the hydrographs may have been compiled for catchments where the groundwater systems have no hydraulic contact with the surface water systems. What can be said about the measurement of discharges in streams and rivers? Normally, discharges can be measured by stream gauging instruments equipped with propellers or lightweight cups. They mea- sure the actual water velocity at various depths at verticals in a cross section. The equipment can be engaged by wading through the stream or river or it can be handled from a bridge or boat. Related water levels in streams and rivers can be measured with staff gauges that are read by an observer at pre-set time intervals of say, an hour or a day. During extreme floods the levels can be estimated from flood marks in trees or at buildings along the stream or river. For a continuous registration of water levels, recorders may be used. These recorders may consist of mechanical devices working with clocks or they may contain computerised parts. Figure 1.15 presents a front view of a clock-driven chart in a recorder connected to a float in a stilling well. The recorder was installed at the ‘right’ bank of the river Rhine, at the border between Germany and The Netherlands. Figure 1.16, on the other hand, shows the set up for a computerised solid state recorder installed in a pipe, driven in a river bottom. The chart presenting an output of the compu- terised data indicates the tidal fluctuations in the river. 1.2.4 The unsaturated zone The unsaturated system The unsaturated zone below land surface could be introduced as ‘the zone where the open space in the rock is only partly filled with water’. Figure 1.17 shows the major subdivisions in this zone. Directly below land surface one finds the root zone which is usually characterised by the largest fluctuations in water content. This zone containing the net- work of plant roots varies in extent, but is usually less than 2 m thick. Below the root zone an intermediate zone may be present to bridge the gap with the capillary fringe. This intermediate zone can be absent in humid areas, but its thickness can also be large, especially in arid areas. © 2003 Swets & Zeitlinger B.V., Lisse Introduction 25 Figure 1.15. Traditional mechanical recorder showing registration chart. The recorder is installed in Lobith, The Netherlands. The capillary fringe is the zone directly above the groundwater table. The groundwater table may be defined as the ‘level where the groundwater pressure is equal to atmospheric pressure’ (see also sec- tion 2.1.2). In the upper part of the fringe, the rock contains many pockets with air and is not saturated, but the bottom part of the fringe is saturated. The thickness of the capillary fringe usually ranges from 0.01 to 0.5 m and depends on the type of rock. In fine-grained rock © 2003 Swets & Zeitlinger B.V., Lisse 26 Introduction to Hydrogeology water level river bank steel wire perforated pipe Figure 1.16. Left: Cross recorder section through a river with a computerised waterlevel pressure sensor recorder and pressure sensor. Right: Output from the com- puterised electronic memory chip in the recorder. time root zone intermediate zone capillary fringe groundwater system solid rock Figure 1.17. Subdivisions below land surface in the unsaturated and saturated zones. groundwater table © 2003 Swets & Zeitlinger B.V., Lisse Introduction 27 rate Case a January July December rate Case b January July December Figure 1.18. Seasonal variation precipitation in recharge to the groundwater potential evapotranspiration table. Case a: Temperate climate with main recharge phase main recharge in the winter occasional recharge season (December – February). Case b: Arid climate with occa- sional recharge in the rainy sea- son (April – August). material the capillary fringe is considerably thicker than in coarse- grained material. Below the capillary fringe, and thus below the groundwater table, the saturated groundwater system is present (see section 1.2.5). Processes in the Part of the precipitation falling on land surface may infiltrate into the unsaturated zone unsaturated zone and increase the water content of this zone (see sec- tion 1.2.2). When the root zone becomes more and more filled with water from precipitation, surplus water may flow downward through the intermediate zone to the capillary fringe and to the groundwater table. This excess water is also referred to as