Homeostasis and Physiological Control: Overview - PDF
Document Details
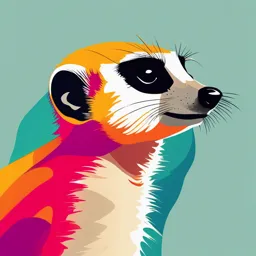
Uploaded by VividBiography3877
Trent University
Tags
Summary
This document overviews homeostasis, the internal environment regulation in animals. It introduces essential components, including sensors and effectors. The document explores cellular processes, including membrane transport, and moves onto physiological control, including the endocrine and nervous systems. It goes on to discuss respiration, circulation, and animal movements.
Full Transcript
Homeostasis chapter 33.3 Environmental changes Animals must deal with constantly changing environments ◦ External ◦ Endogenous (self imposed) must maintain suitable internal environment ◦ Homeostasis Definition: the active regulation and maintenance of a stable internal physiolog...
Homeostasis chapter 33.3 Environmental changes Animals must deal with constantly changing environments ◦ External ◦ Endogenous (self imposed) must maintain suitable internal environment ◦ Homeostasis Definition: the active regulation and maintenance of a stable internal physiological state in the face of changing external environment Factors in homeostasis Skin temperature Breathing rate Heart rate Sweating Heat production Homeostasis allows animals to invade “physiologically unfriendly” environments Essential components 1. Sensors ( receptors for temperature, pH, touch ) 2. Effectors ( muscles, sweat glands ) 3. Response ( hear production ) all tied together into the negative feedback loop Example: thermostat Stimulus: cold Sensor: thermostat Effector: heater Negative feedback loop Response: heat Organisms fall into two categories when responding to environmental changes 1. Conformers ( do not maintain homeostasis ) 2. Regulators (do maintain homeostasis ) Environmental changes proceeds at different rates and evokes different responses Minutes to hours Physiological adjustment Exercise - almost instantaneous Temperature - easily reversed Sun-light Weeks to months Acclimatization Altitude. - slower, over many days Day length. - reversible Geologic time Evolutionary change New habits. - selection on new traits Climate change - non-reversible Cellular homeostasis chapter 1.3; 3.1-.3 All organisms are made of a single cell or an ensemble of cells Cells are defined by membranes Membranes: 1. separate the inside of the cell from the outside 2. Surround many internal structures 3. Composed of lipids, proteins and carbohydrates Membranes contain 2 phospholipid layers Hydrophilic ◦ Polar head group Hydrophobic ◦ Nonpolar tails Phospholipids arrange themselves spontaneously ◦ Bilayer Membrane phospholipids are able to move in the membrane ◦ Membrane is fluid ◦ Individual fatty acid chains are also able to flex or bend ◦ Fluidity depends on the fatty acids present ‣ Double bonds, length of tails ◦ Saturated fatty acid chains lack double bonds resulting in phospholipids with a straight structure that favours tight packing ii ii unsaturated: oleic acid ◦ Unsaturated fatty acids have one or more double bonds that introduce kinks in phospholipids, reducing tightness and packing unsaturatedoleicacid Cold-water species have more double-bonds to maintain fluid membranes i i Plasma membrane critical 4homeostasis Characteristics Feature in ALL cells Defines the. Cell boundary Separates internal contents from surrounding environments Plasma membrane is a selective barrier Certain items can move freely, some others only under certain conditions; others cannot reasons for limited permeability Lipid builayer is hydrophobic - prevents ion movement Many macromolecules are too large Gasses, lipids, small polar molecules can cross Selective permeability is key to maintaining homeostasis Passive transport - simple diffusion Facilitated diffusion Water moves in & out of cells via osmosis Membrane allows passage of water but not solute Aquaporins = protein channels allowing for facilitated diffusion Active transport is movement against a concentration gradient Passive transport works only when rye concentration gradient is in the right direction Eg., nutrients: higher on the outside to lower on the inside Eg.m waste: higher on the inside and lower on the outside Two kinds of active transport 1. Primary active transport 2. Secondary active transport 1. primary active transport uses energy of ATP Eg., sodium-potassium pump (Na+/K + ATPase) Step 1 & 2: three sodium ions are pumped out of the cell against their concentration gradient. Step 3 & 4: two potassium ions are pumped into the cell against their concentration gradient Antiporter: ions moving in opposite directions Symporter: co-transporter, ions move in same direction 2. Secondary active transport uses ATP indirectly Protons are pumped across the membrane by primary active transport The proton pump generates an electrochemical gradient, with higher concentration of protons outside the cell in a lower concentration of protons inside the cell An anti-porter uses the proton electrochemical gradient to move different molecule out of the cell against its concentration gradient 888s pertonic Isotonic Iypotonic Solute concentration in extra cellular space Physiological control Chapter 35.1-35.5 In animals homeostasis is maintained by the endocrine and nervous system Endocrine System ◦ Realeases hormones ‣ A chemical substance released into bloodstream, circulates throughout the body and exerts influence on distant cells ◦ Response is slow and widespread Nervous system ◦ Composed of neurons (nerve cells ) ◦ Signals are fast and targeted ◦ Neurons typically make contact with the target cell Endocrine system Works via negative feedback Endocrine cells ◦ Typically organized into endocrine glands ‣ Eg., thyroid gland, adrenal gland ◦ glands are ductless - release hormones into capillaries among doctrine cells ◦ Target cells possess receptor molecules that recognize the hormone and can bind the hormone ◦ Only cells and tissues with the receptor can respond Long distance signaling Release of a hormone into the bloodstream that affects distant cells Example: Stimulus: high glucose (right after a meal ) Sensor: pancreasβ cells Effector: insulin Body cells take up glucose. Muscle and liver take up glucose and store it as glycogen 3 Y response: decrease in blood glucose Nervous system Fundamental unit is the neuron 1. Stimuli are received by the dendrites and cell body 2. Synaptic stimuli are summed at the axon hillock, where an action potential is triggered 3. Action potentials are conducted to the axon terminal, where they cause the release of neurotransmitters that are stored in vesicles. Bind to receptors on the postsynpatic cell membrane, creating a new signal in the post synaptic neuron. Network of interconnected cells Neuron = nerve cell Neuron resting membrane potential The Na+ -K pump moves Na+ ions out of the cell and K+ ions into the cell K+ channels allow K+ ions to “leak” out of the cell, resulting in a negative resting potential on the inside relative to the outside of the cell Membrane potential can be measured with small glass electrodes When a neuron is excited, inside becomes less negative: depolarized Depolarization starts in dendrites, in response to a neurotransmitter and travels to cell body If depolarization at the axon hillock exceeds the threshold voltage (potential), the cell fires an action potential Depolarization and repolarization Mv membrane potential 1. Some input depolarizes the cell membrane at the axon hillock above the threshold potential 2. Voltage gated Na+ channels open, and Na+ rapidly enters the cell, causing a positive spike in the membrane potential. K+ channels open more slowly. 3. As the voltage rises +40 MV, Na+ channels close and are in activated and voltage gated K+ channels remain open, allowing K+ ions to leave the cell and causing the membrane potential to become more negative 4. In overshoe in the amount of K+ ions that leave the cell causes the cell membrane to be hyperpolarized this results in a refractory period. 5. Gradually, the membrane returns to resting as access K+ ions are returned to the cell assisted by Na+ - K+ pumps. Refractory Period The period during which the inside membrane voltage falls below, and then returns to the resting potential A neuron cannot fire a second action potential, because: ◦ Voltage-gated Na+ channels are closed/inactive ◦ Voltage-gated K+ channels are open ◦ The duration of the refractory period varies, but limits the fastest firing frequency action potentials propagate a long the axon by sequentially opening and closing adjacent voltage gated Na+ and K+ channels They are self propagating and travel in only One Direction Saltatory propagation 1. Synaptic transmission begins with the action potential conduction to the axon terminal 2. Depolarization of the axon terminal opens voltage gated Ca2+ channels 3. Vesicles respond by fusing with the presynaptic membrane, releasing neuro transmitters into the synaptic cleft 4. Neural transmitters bind with receptors on the postsynaptic cell that are ligand gated ion channels, opening the channels to allow and ions and causing a change in membrane potential 5. Neural transmitters are actively real absorbed into the presynaptic terminal and stored in vesicles until the next action potential arrives Signals can be excitatory or inhibitory Binding of neurotransmitter to postsynaptic cells result an opening of ion channels: ◦ Generate postsynaptic potential ( graded potential) The effect of Neuro transmitter binding depends on types of postsynaptic cell. ◦ If cell depolarized - potential is excitatory EPSP ‣ Opening of ligand gated Na+ channels ◦ if cell hyperpolarized - potential is inhibitory I PSP ‣ Opening ligand gated CI- or K+ channels Signals or pass between neurons are junctions called synapses Sensory transduction Sensory receptor cells Chemoreceptors Mechanoreceptors Vertebrate photoreceptors ◦ Example: hearing relies on mechanoreceptors Respiration & Circulation chapter 38 Bulk Flow All eucaryotic organisms require O2 for ATP production Single celled organisms and simple, multicellular animals exchange compounds with the environment by diffusion Larger animals rely on a combination of diffusion and bulk flow for gas exchange Bulk flow has two steps ◦ Ventilation: movement of a medium ( air or water ) over respiratory surface (lung or gill) ◦ Circulation: movement of body fluids, containing dissolved gases (require pumps) The goal of gas transport it, so deliver oxygen to mitochondria- bulk flow maximizes gradients Bulk flow/diffusion 1. Ventilation buy bulk flow breathing moves air containing oxygen into the lungs and air containing carbon dioxide out of the lungs. 2. Diffusion across the respiratory surface, oxygen diffuses from the lungs into blood and carbon dioxide diffuses out of the blood into lungs 3. Circulation buy, bulk, flow, oxygen, and carbon dioxide are transported by the circulatory system to and from cells 4. Diffusion between blood and cells oxygen diffuses from the blood into the cells and carbon dioxide defuses out of the cells into the blood Ventilation can be active or passive Goal is to reduce formation of static boundary layers Active: ◦ Animal created ventilatory currents that flows across gas exchange surface ◦ Uses suction or positive pressure ◦ Expends, metabolic energy Passive ◦ Environmental air or water currents, Indus flow to, and from the gas exchange membrane ◦ No use of metabolic energy Gas exchange organs in aquatic animals Examples: ◦ Tube worm: external gills ◦ Aquatic salamander: external gills ◦ Fish: internal gills Bony fish pump water across gills; some species ventilate by swimming ( ram ventilation) Fish have unidirectional respiration and countercurrent blood flow Concurrent exchange There are many examples in biology and physics where properties such as heat or oxygen are exchanged between two moving fluids Land animals can achieve higher oxygen uptake rates 1. Oxygen content of air is much higher than water 2. Oxygen diffuses 8000 times faster in air than water 3. Air is less dense and less vicious than water; requires less energy to pump Most land vertebrates use tidal ventilation of lungs ◦ Negative pressure draws air into lungs ◦ Positive pressure expels air from lungs mammals increase their lung volume by actively expanding their thoracic cavity to draw oxygen rich air into the lungs (inhalation) and expire oxygen-poor air from the lungs using passive elastic recoil (exhalation) In mammals gas exchange is in the alveoli Alveolar sacs are blind ended; never fully emptied ◦ Amount of O2 and CO2 in alveoli differ from environment lungs contain stale air : ◦ On inspiration, fresh air pushes stale air deeper into the lungs ◦ End of resting inhalation, 12% and airwaves is fresh and 88% left over from previous breaths surfactants reduce surface tension in alveoli (allow for easier inflation of the lungs) Alveoli surrounded by net work of capillaries Birds use Uni directional ventilation and cross current flow 1. First inhalation draws oxygen, rich air into posterior air sacs 2. First exhalation moves fresh air into lung 3. Second inhalation moves, still, oxygen, depleted air from lung into anterior, air sacs 4. Second exhalation moves air out of anterior air sacs Chemo receptors in the brain stem, detect CO2 and H+ Carotid and aortic body is detect 02 and H+ If CO2 is too high chemoreceptors in brain stem, stimulate respiratory muscles Components of circulatory systems What’s oxygen diffuses into an animals blood it must be transported to the tissues and cells Circulatory systems, move fluids by increasing the pressure of the fluid in one part of the body Fluid flow through the body down pressure gradient Three main components are needed: ◦ Fluid that circulates through the system ◦ System of tubes, channels, or spaces ◦ Pump or propulsive structures Vertebrate blood A mammal ◦ Plasma 55% ◦ White blood cells 1% ◦ Red blood cells 45% = hematocrit Fish ◦ Plasma 65% ◦ White blood cells 1% ◦ Red blood cells 30% hematocrit % Fraction of blood made up by red blood cells Affects resistance Hemoglobin reversibly binds oxygen O2 and CO2 can dissolve in plasma; the amount dissolved is a measure of the gas’s solubility ◦ Solubility of O2 < CO2 Vertebrates and invertebrates evolved hemoglobin (Hb) ◦ Globular protein with 4 subunits ( in vertebrates) ◦ Each subunit surrounds a heme group, containing iron ◦ Each heme group binds to one O2 molecule ◦ In red blood cells of vertebrates; in hemolymph of invertebrates Transportation differs between O2 and CO2 ◦ In vertebrates, O2diffuses into blood, then into RBC, and binds reversibly to heme group for transport ◦ CO2 carried in plasma as bicarbonate (HCO3-) and H+ Hemoglobin exhibits cooperative binding - favours binding at lungs and unloading at tissues Open circulatory systems Blood flows through a vessel with muscular thickenings that act as a pump Blood empties into an open body cavity to supply the tissues with nutrients and is returned to the circulation ◦ Insect do not use blood supply for transportation oxygen Closed circulatory system Blood flows through the connected blood vessels pumped by the muscular hearts, The blood flows through vessels to supply tissues with nutrients Vessel types Arteriole qiiiiffr Venule Capillary bed Capillary in Fish have two heart chambers and a single-circuit circulation 1. Deoxygenated blood enters the atrium from a main vein and then the ventricle 2. Deoxygenated blood is pumped from the ventricle into a main artery Land vertebrate circulatory systems 1. Hearts evolved with > 2 chambers, which separate circulation to the gas exchange organs fro, circulation to the body tissues 2. Double-circuit circulation 3. More efficient gas exchange and increases O2 delivery A. Oxygen is extracted from air ( not water ) 4. higher metabolic rates and greater activity Anatomy of 4-chambered heart & blood flow through heart 1. deoxygenated blood enters the right atrium from the inferior and superior venae cavae 2. Deox. Blood passes through the right AV valve and enters the right ventricle 3. Deox. Blood is pumped into the pulmonary arteries through the pulmonary valve 4. Oxygenated blood returns from the lungs to the left atrium 5. Oxygenated blood enters the left ventricle through the left AV valve 6. Oxygenated blood is pumped by the left ventricle through the aortic valve into the systemic circulation Circulation in mammals and birds separated into pulmonary and systemic circuits ◦ Allows for: ‣ Increased supply of oxygenated blood to tissues(pumped at a high pressure) ‣ Increased uptake, O2 gas exchange surface (due to lower pressure and more time for extraction) Coordinating contraction in heart muscle cells Cardiac muscle must have coordinated contracts Cardiac muscle cells differ from skeletal muscle cells: 1. specialized cardiac cells generate action potentials on their own, independently of the nervous system A. Pacemaker B. Sinoatrial (SA) and atrioventricular (AV) nodes 2. cardiac muscle cellls are electrically coupled via gap junctions Gap junctions transmit electrical signals cenz EFFI membranes Depolarization in pacemaker results in contraction 1. The SA-pacemaker cells generate action potentials that spread through the atria. Atria contract 2. Signals from the SA pacemaker reach the AV node, which activates fires 3. APs are transmitted through a set of modified muscle fibres A. Depolarization spreads from the modified muscle fibres through entire ventricle the ventricles contract Animal energetically ch 39.1 Metabolic rates The overall rate of energy use by an organism Can be measured as oxygen consumption Cott20260 6H 0 CQ ATP Affected by many factors: 1. activity level 2. Body size 3. Body temperature Metabolic rate increases with activity At the start of exercise, oxygen consumption rises, and then levels off When I exercise ends oxygen consumption false gradually to resting levels Cells are re-synthesizing ATP store; Metabolizing lactic acid Mobile ankle rate increases with body size Metabolic rate is linked with temperature ◦ Chemical reactions in an animals, body speeds or slows down with changing temperatures animals have a thermal strategy ◦ Combination of behavioural bio, chemical, and physiological responses that ensure body temperature is within an acceptable limit T B Terminologies of thermal strategies 1. source of heat A. Endotherm a. Generates internal heat to maintain body temperature B. ectotherm a. Environment determines bodies temperature 2. response to environmental temperature change A. Homeotherm a. Stable, body temperature (balance heat gain/loss) B. poikilotherm a. Variable body temperature Maintaining optimal body temperature Ectotherm an endotherm’s can regulate temperature through behaviour Common behavioural mechanisms ◦ Orienting toward/away from sun ◦ Seeking shelter, breezes etc. endotherms use behaviour as first line of defense against thermal stress Controlling blood flow to the body surface affects heat exchange Normal/cool body temperature ◦ Constriction of Arterioles reduces blood flow to skin surface High body temperature ◦ Decrease constriction of Arterioles ‣ Vessels dilate ‣ Blood directed to skin surface a) response to cold temperature B) response to high temperature Heat production : endotherms Shivering thermogenesis ◦ Skeletal muscles pull against each other ◦ ATP converted to ADP ( heat is released) nonshivering thermogenesis ◦ Occurs in mammals (and some birds) ◦ Brown adipose tissue (BAT) Heat conversion: endotherm evolutionary adaptations Large body size Reduced extremities insulation Fur and feathers Traps air(when dry) Requires grooming Avoiding overheating Evaporative cooling ◦ Direct contact with water ◦ Sweating or panting (dehydration; generates heat) heat windows - rabbit ears Survival is easier when temperature < the thermoneutral zone (TNZ) than > TNZ Ectothermy & endothermy Ectotherm’s benefit from a lower metabolic rates Expend little on thermal regulation Invest in growth and reproduction Less time forging benefits balanced by costs Limited ability to regulate body temperature Limits activity (duration, and season) Limits geographic distribution Limited burst of high activity Thermal regulation relies on a regulatory system is it in? At high temperatures metabolic rate may increase ( panting ) Animal Movement Ch 36-36.2 Muscles 1. Skeletal muscle 2. Cardiac muscle i ENDstriated striped 3. Smooth muscle ei.ie Biological motors of body Composed of multinucleated muscle cells ( fibers ) Use ATP generated through cellular respiration Use contractile proteins (Actin & myosin ) to generate force Striated - appear striped actin and myosin arranged in regularly, repeating pattern Smooth - appear uniform Walls of arteries, digestive and excretory systems Actin and myosin arranges in irregular pattern Organization of skeletal muscle Compose of elongate cells called muscle fibres that are embedded in surroundings of connective tissues Individual fibres have many microfibrils with striated appearance due to the regular molecular organization Myofibrils contain thin and thick filaments Thin ( actin ) filament 880888 ftp.gyosin Actin molecule Troponin Fetterman EE.tn ini tne Tropomyosin 88T Thichmyosinfilament Myosin molecule Thick filament ( myosin ) Muscle fibre = muscle cell ◦ Sacromere ‣ Contractile unit of muscle ‣ Defined as region between two Z-discs Structure of muscle Actin ( thin filament ) Myosin ( thick filament ) Titin (prevents overstretching ) EM: dark colour = overlap Lengths of myosin and actin do not change Muscle Shortening Interactions between myosin and actin cause muscle fibres (cells ) to shorten and produce force Two Heads of the myosin molecules bind to actin at specific sites to form cross bridges Myosin filaments pull actin using cross bridges Key is for myosin filaments to slide is their ability to undergo confirmation change (pivoting back-and-forth) Cross bridge cycle = forming, and breaking bonds between actin and myosin 1. The myosin head binds ATP Leading to detachment from actin 2. The myosin head catalyzes the hydrolysis of ATP, forming ADP and Pi, and cocking the myosin head back 3. The myosin head binds actin forming a cross bridge 4. ADP and PI are released producing a power stroke that generates Forrest and causes the thin filament to slide relative to the thick filament, and then the sacromere to shorten Excitation contraction coupling Muscle cells are electrically excitable Skeletal muscle fibres are activated by impulse transmitted by motor nerves to the skeletal muscle cell at the fibres motor endplate 1. an action potential from a motor neuron leads to the release of acetylcholine and depolarization of the skeletal muscle cell 2. The depolarization is conducted into the interior of the fibre by the infoldings of the plasma membrane Actin and myosin filaments conform cross bridges only when the myosin binding sites on actin are exposed Depolarization causes the myosin binding sites to be available for actin binding Depolarization (excitation) leads to shortening (contraction) 1. an action potential from the motor neuron leads to the release of acetylcholine and depolarization of the skeletal muscle cell 2. The depolarization is conducted into the interior of the fibre by the infoldings of the cell membrane 3. Depolarization leads to the release of CA2+ from the sacroplasmic reticulum 4. Ca2+ fines to troponins which causes movement of tropomyosin , exposure of myosin-binding sites on actin and formation of cross bridges to generate force and produce shortening of the muscle Force and velocity are inversely related Muscles shorten fastest when there is little force High contraction velocities, if you are cross bridges form, reducing force Large force production requires low velocity Muscle contractions can be shortening, lengthening, or stay same(isometric) Muscle force depends on stimulation frequency An action potential from a motor nerve causes a muscle fibre to produce a twitch contraction with a slight delay in force For twitch contraction to occur, Caa++ must be released from sacroplasmic reticulum (SR) ; Caa++ then pump back into SR Force summation and tetanus Muscle force sums to a higher levels when action potential stimulate the muscle at higher rates, reaching a tetanus Motor Units = motor neuron and the population of muscle fibres (cells) it innervates Motor unit size determines how finely a muscles force can be controlled Muscles force depends on: A. Stimulation frequency B. The number of motor units (the number of muscle fibres) that are activated Types of Skeletal muscle fibers Fast twitch glycolytic (white) fibres are stand light yellow Slow twitch oxidative(red) fibres are stained red Fast twitch fibers Large Generate more force than slow twitch Energy supplied by anaerobic glycolysis ◦ Few microchrondia or capillaries or myoglobin ◦ Develop force rapidly; fatigue quickly Slow twitch fibres Develop force more slowly; resist, fatigue Aerobic, respiration, and oxidative phosphorylation Mitochondria, well supplied with oxygen ◦ Surrounded by capillaries ◦ Contain abundant myoglobin ‣ Muscle oxygen storage molecule; gives slow twitch fibres a red colour Cheetahs are glycolyic fast twitch Antelopes are oxidative, slow twitch + specialized, fast twitch