Introduction to Autonomic Pharmacology PDF
Document Details
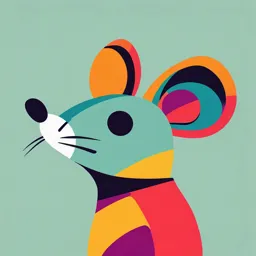
Uploaded by MerryGyrolite2116
University of Kentucky
Mohammed Hassan Alnazeer
Tags
Summary
This document provides an introduction to autonomic pharmacology, exploring the nervous system, chemical transmission, and functional organization. It delves into the anatomy of the autonomic nervous system, discussing sympathetic and parasympathetic divisions, and the enteric nervous system. Key topics covered include neurotransmitter chemistry, specifically focusing on cholinergic and adrenergic transmission and related pharmacology.
Full Transcript
Autonomic Pharmacology Mohammed Hassan Alnazeer B.pharm, M.pharm (clinical pharmacy), BCPS UofK, Dept of Pharmacology What are the reactions of your bodies?? What are the reactions of your bodies?? The Nervous System The nervous system is conventionally divided...
Autonomic Pharmacology Mohammed Hassan Alnazeer B.pharm, M.pharm (clinical pharmacy), BCPS UofK, Dept of Pharmacology What are the reactions of your bodies?? What are the reactions of your bodies?? The Nervous System The nervous system is conventionally divided into the central nervous system (the brain and spinal cord), and the peripheral nervous system (neuronal tissues outside the CNS). The peripheral nervous system can be divided into sensory and motor neurons. The motor (efferent) portion of the nervous system can be divided into two major subdivisions: autonomic nervous system somatic nervous system. Nervous system Nervous system The autonomic nervous system (ANS) is largely independent (autonomous) in that its activities are not under direct conscious control. It is concerned primarily with visceral functions such as cardiac output, blood flow to various organs, and digestion, which are necessary for life. Evidence is accumulating that the ANS, especially the vagus nerve, also influences immune function and some CNS functions such as seizure discharge. The somatic subdivision is largely concerned with consciously controlled functions such as movement, respiration, and posture. In the nervous system, chemical transmission occurs between nerve cells, Also between nerve cells and their effector cells. Chemical transmission takes place through the release of small amounts of transmitter substances from the nerve terminals into the synaptic cleft. The transmitter crosses the cleft by diffusion and activates or inhibits the postsynaptic cell by binding to a specialized receptor molecule. In a few cases, retrograde transmission may occur from the postsynaptic cell to the presynaptic neuron terminal and modify its subsequent activity. Electrical Transmission Electrical & Chemical Transmission Functional Organization of Autonomic Activity Reflex Arc Reflex Arc can be Classified Functionally into: 1. Somatic Reflexes 2. Autonomic reflexes Autonomic function is integrated and regulated at many levels, from the CNS (Brain and/or spinal cord) to the effector cells. Most regulation uses negative feedback, but several other mechanisms have been identified. Negative feedback is particularly important in the responses of the ANS to the administration of autonomic drugs. At the highest level, midbrain and medulla, the ANS and the endocrine system are integrated with each other, with sensory input, and with information from higher CNS centers, including the cerebral cortex. Example for CVS Integration Anatomy Of The ANS What is Anatomical Difference between Somatic and Autonomic nervous system? Somatic vs. Autonomic NS Anatomy of the ANS The ANS lends itself to division on anatomic grounds into two major portions: the sympathetic (thoracolumbar) division and the parasympathetic (craniosacral) division. Picture Vagal Nerve The sympathetic preganglionic fibers leave the CNS through the thoracic and lumbar spinal nerves. Most sympathetic preganglionic fibers are short and terminate in ganglia located in the paravertebral chains that lie on either side of the spinal column. The remaining sympathetic preganglionic fibers are somewhat longer. From the ganglia, postganglionic sympathetic fibers run to the tissues innervated. Paravertebral chains The parasympathetic preganglionic fibers leave the CNS through the cranial nerves (especially the third, seventh, ninth, and tenth) and the third and fourth sacral spinal nerve roots. Some preganglionic parasympathetic fibers terminate in parasympathetic ganglia located outside the organs innervated. However, the majority of parasympathetic preganglionic fibers terminate on ganglion cells distributed diffusely or in networks in the walls of the innervated organs. Sympathetic vs. Parasympathatic NS Sympathetic vs. Parasympathatic NS Sympathetic “Fight or flight” Exercise, Excitement, Emergency, and Embarrassment Parasympathetic “Rest and digest” Digestion, Defecation, and Diuresis The enteric nervous system (ENS) is sometimes considered a third division of the ANS. It is a large and highly organized collection of neurons located in the walls of the GIT. It is found in the wall of the GI tract from the esophagus to the distal colon and is involved in both motor and secretory activities of the gut. The ENS includes the myenteric plexus and the submucous plexus. These neuronal networks receive fibers from the parasympathetic and sympathetic systems. Also, they receive sensory input from within the wall of the gut. Enteric Nervous System Enteric Nervous System Fibers from the neuronal cell bodies in these plexuses travel forward, backward, and in a circular direction to the smooth muscle of the gut. Sensory fibers transmit chemical and mechanical information from the mucosa and from stretch receptors to motor neurons in the plexuses and to postganglionic neurons in the sympathetic ganglia. Neurotransmitter Chemistry of the ANS The traditional classification of autonomic nerves is based on the primary transmitter molecules, acetylcholine (Ach) or norepinephrine (NE)— released from their terminal varicosities. A large number of peripheral ANS fibers synthesize and release (Ach) ; they are cholinergic fibers; that is, they work by releasing (Ach) , these include all pre-ganglionic efferent autonomic (symmpathetic & parasymmpathetic) fibers and the somatic (non-autonomic) motor fibers to skeletal muscle as well. Thus, almost all efferent fibers leaving the CNS are cholinergic. Picture Most parasympathetic postganglionic fibers are cholinergic. A significant No. of parasympathetic postganglionic neurons utilize nitric oxide or peptides as transmitters or as cotransmitters. Most postganglionic sympathetic fibers release (NE) (also known as noradrenaline); they are noradrenergic (often called simply “adrenergic”) fibers; that is, they work by releasing (NE) , some sympathetic fibers release (Ach) or Dopamine rather than EP…. Check the examples???? Adrenal medullary cells are embryologically analogous to post- ganglionic sympathetic fibers, release both epinephrine and (NE) Picture Cholinergic Transmission Cholinergic Transmission The terminals of cholinergic neurons contain large numbers of small membrane-bound vesicles concentrated near the synaptic portion, they contain most of the (Ach). Vesicles are provided with vesicle-associated membrane proteins (VAMPs), which serve to align them with release sites on the inner neuronal cell membrane and participate in triggering the release of transmitter. (Ach) is synthesized in the cytoplasm from acetyl-CoA, which is is synthesized in mitochondria, and from choline through the reaction of the enzyme choline acetyltransferase (ChAT). Choline is transported from the extracellular fluid into the neuron terminal by a sodium-dependent membrane choline transporter ( CHT). This symporter can be blocked by a group of research drugs called hemicholiniums. Once synthesized, (Ach) is transported from the cytoplasm into the vesicles by a vesicle-associated transporter (VAT) that is driven by proton efflux. This antiporter can be blocked by the research drug vesamicol. Storage of (Ach) into vesicle is accomplished by the packaging of “quanta” of (Ach) molecules. Most of the vesicular (Ach) is bound to negatively charged vesicular proteoglycan (VPG). Structure of acetylcholine?? + + H Physiologic release of transmitter from the vesicles is dependent on extracellular calcium and occurs when an action potential reaches the terminal and triggers sufficient influx of calcium ions via N-type calcium channels. Calcium interacts with the VAMP, known as synaptotagmin, on the vesicle membrane and triggers fusion of the vesicle membrane with the terminal membrane and opening of a pore into the synapse. The opening of the pore and inrush of cations results in release of the (Ach) from the proteoglycan and exocytotic expulsion into the synaptic cleft. In addition to (Ach) , several cotransmitters are released at the same time. Co-transmitters Examples for co-transmitters The (Ach) vesicle release process is blocked by botulinum toxin through the enzymatic removal of two amino acids from one or more of the fusion proteins. Botulinum toxin is a protein and neurotoxin produced by the bacterium Clostridium botulinum. Read about Botulism?? (causes, presentation, and TTT) Read about medical and cosmetic use of Botulinum toxin? Picture After release from the presynaptic terminal, (Ach) molecules may bind to and activate an (Ach) receptor ( cholinoceptor ). Eventually (and usually very rapidly), all of the (Ach) released diffuses within range of an acetyl-cholinesterase (AChE) molecule. AChE very efficiently splits (Ach) into choline and acetate, neither of which has significant transmitter effect, and thereby terminates the action of the transmitter. Most cholinergic synapses are richly supplied with acetylcholinesterase; the half-life of (Ach) molecules in the synapse is therefore very short (a fraction of a second). Acetylcholinesterase is also found in other tissues, eg, RBCs. Other cholinesterases with a lower specificity for (Ach) , including butyrylcholinesterase [pseudocholinesterase], are found in blood plasma, liver, glia, and many other tissues.) Effects of drugs in Cholinergic transmission Adrenergic Transmission The terminals of adrenergic neurons contain large numbers of small membrane-bound vesicles concentrated near the synaptic portion, they contain most of the (NE). Adrenergic neurons transport a precursor amino acid (tyrosine) into the nerve ending, then synthesize the catecholamine transmitter, and finally store it in membrane-bound vesicles. In most sympathetic postganglionic neurons, (NE) is the final product. In the adrenal medulla and certain areas of the brain, some (NE) is further converted to epinephrine. In dopaminergic neurons, synthesis terminates with dopamine. L - Dopa Decarboxylase Adrenal Medulla Tyrosine Hydroxylase Several processes in these nerve terminals are potential sites of drug action. One of these, the conversion of tyrosine to dopa, is the rate-limiting step in catecholamine transmitter synthesis. It can be inhibited by the tyrosine analog metyrosine. A high-affinity antiporter for catecholamines located in the wall of the storage vesicle (vesicular monoamine transporter, VMAT) can be inhibited by the reserpine alkaloids. Reserpine causes depletion of transmitter stores. Another transporter (NE transporter, NET) carries (NE) and similar molecules back into the cell cytoplasm from the synaptic cleft. NET is also commonly called uptake 1 or reuptake 1 and is partially responsible for the termination of synaptic activity. NET can be inhibited by cocaine and tricyclic antidepressant drugs, resulting in an increase of transmitter activity in the synaptic cleft. Release of the vesicular transmitter store from noradrenergic nerve endings is similar to the calcium-dependent process previously described for cholinergic terminals. In addition to the primary transmitter (NE), adenosine triphosphate (ATP), dopamine-β-hydroxylase, and peptide cotransmitters are also released into the synaptic cleft. Indirectly acting and mixed sympathomimetics, eg, tyramine, amphetamines, and ephedrine, are capable of releasing stored transmitter from noradrenergic nerve endings by a calcium- independent process. These drugs are poor agonists (some are inactive) at adrenoceptors, but they are excellent substrates for monoamine transporters. As a result, they are avidly taken up into noradrenergic nerve endings by NET. In the nerve ending, they are then transported into the vesicles, displacing (NE) , which is subsequently expelled into the synaptic space by reverse transport via NET. Amphetamines also inhibit monoamine oxidase and have other effects that result in increased (NE) activity in the synapse. Their action does not require vesicle exocytosis. Adrenergic transmission How drugs affect adrenergic transmission?? (NE) and epinephrine can be metabolized by several enzymes, Monoamine oxidase (MAO) (Types of MAO??) which is abundant in noradrenergic nerve terminals and many other places, such as liver and intestinal epithelium and Catechol- O- methyltransferase (COMT) which is absent from noradrenergic neurons but present in the adrenal medulla and many other cells and tissues. Because of the high activity of monoamine oxidase (MAO) in the mitochondria of the nerve terminal, there is significant turnover of (NE) even in the resting terminal. The metabolic products are excreted in the urine. However, metabolism is not the primary mechanism for termination of action of (NE) physiologically released from noradrenergic nerves. Termination of noradrenergic transmission results from two processes: simple diffusion away from the receptor site (with eventual metabolism in the plasma or liver) and reuptake into the nerve terminal by NET or into perisynaptic glia or other cells. Think about the following: What is the clinical significance of measuring: 1. 24 hrs urine VMA? 2. 24 hrs Urine homonanillic acid? Phyoechromocytoma? Neuroblastoma? Effects of drugs in Adrenergic transmission Name the following enzymes?? 1 2 3 4 Autonomic Receptors Autonomic Receptors The primary (Ach) receptor subtypes were named after the alkaloids originally used in their identification: muscarine and nicotine, thus muscarinic and nicotinic receptors. The term cholinoceptor denotes receptors (both muscarinic and nicotinic) that respond to acetylcholine. In the case of receptors associated with noradrenergic nerves, the term adrenoceptor is widely used to describe receptors that respond to catecholamines such as NE. The general class of adrenoceptors can be further subdivided into α- adrenoceptor, β - adrenoceptor, and dopamine receptor types on the basis of both agonist and antagonis selectivity and on genomic grounds. Non-adrenergic, Non-cholinergic (NANC) neurons It has been known for many years that autonomic effector tissues (eg, gut, airways, bladder) contain nerve fibers that do not show the histochemical characteristics of either cholinergic or adrenergic fibers. Although peptides are the most common transmitter substances found in these nerve endings, other substances, eg, nitric oxide and purines, are also present in many nerve terminals. The enteric system in the gut wall is the most extensively studied system containing NANC neurons in addition to cholinergic and adrenergic fibers. In the small intestine, for example, these neurons contain one or more of the following: nitric oxide synthase (which produces nitric oxide; NO), calcitonin gene-related peptide, cholecystokinin, dynorphin, enkephalins, gastrin-releasing peptide, 5- hydroxytryptamine (serotonin), neuropeptide Y, somatostatin, substance P, and vasoactive intestinal peptide (VIP). Nonadrenergic Noncholinergic Transmitters Pre-synaptic Regulation of transmitter release The principle of negative feedback control is also found at the presynaptic level of autonomic function. A well-documented mechanism involves the α 2 receptor located on noradrenergic nerve terminals. This receptor is activated by NE and similar molecules; activation diminishes further release of NE from these nerve endings. Conversely, a presynaptic β receptor appears to facilitate the release of NE from some adrenergic neurons. Presynaptic receptors that respond to the primary transmitter substance released by the nerve ending are called autoreceptors. Autoreceptors are usually inhibitory, but in addition to the excitatory β receptors on noradrenergic fibers, many cholinergic fibers, especially somatic motor fibers, have excitatory nicotinic autoreceptors. Control of transmitter release is not limited to modulation by the transmitter itself. Nerve terminals also carry regulatory receptors (heteroreceptors) that respond to many other substances. Such heteroreceptors may be activated by substances released from other nerve terminals that synapse with the nerve ending. For example, some vagal fibers in the myocardium synapse on noradrenergic nerve terminals and inhibit NE release. Alternatively, the ligands for these receptors may diffuse to the receptors from the blood or from nearby tissues. Autoreceptor, heteroreceptor, and modulatory effects on nerve terminals Post-synaptic Regulation Postsynaptic regulation can be considered from two perspectives: modulation by previous activity at the primary receptor (which may up- or down-regulate receptor number or desensitize receptors; and modulation by other simultaneous events. The first mechanism has been well documented in several receptor-effector systems. Up-regulation and down-regulation are known to occur in response to decreased or increased activation, respectively, of the receptors. In skeletal muscle, Surgical denervation results in marked proliferation of nicotinic cholinoceptors. Why?? Also, the prolonged administration of large doses of reserpine, a norepinephrine depleter, can cause increased sensitivity of the smooth muscle and cardiac muscle effector cells. Why?? The second mechanism involves modulation of the primary transmitter-receptor event by events evoked by the same or other transmitters acting on different postsynaptic receptors. Think about the following: The effect of Hypothyroidism and hyperthyroidism (Thyroid hormone level) on the regulation of post-synaptic adrenoceptors??