Inorganic Chemistry - Chapter 5: Chemical Bonding - PDF
Document Details
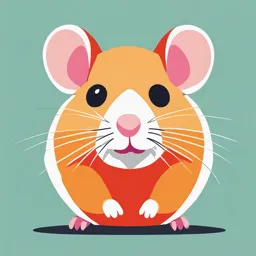
Uploaded by FinerColumbus3217
Damanhour University
Tags
Summary
This document details chemical bonding concepts using the Lewis theory. It covers different types of bonds, including ionic and covalent bonds, and explores the electronic theory of valence. The chapter also discusses the octet rule and exceptions to it.
Full Transcript
5 C H A P T E R Chemical Bonding – Lewis Theory C O N T E N T S ELECTRONIC THEORY OF VALENCE IONIC BOND EXAMPLES OF IONIC COMPOUNDS CHARACTERISTICS OF IONIC COMPOUNDS COVALENT BOND CONDITIONS FOR FORMATION OF COVALENT BOND EXAMPLES...
5 C H A P T E R Chemical Bonding – Lewis Theory C O N T E N T S ELECTRONIC THEORY OF VALENCE IONIC BOND EXAMPLES OF IONIC COMPOUNDS CHARACTERISTICS OF IONIC COMPOUNDS COVALENT BOND CONDITIONS FOR FORMATION OF COVALENT BOND EXAMPLES OF COVALENT COMPOUNDS CHARACTERISTICS OF COVALENT COMPOUNDS TERMS AND DEFINITIONS CO-ORDINATE COVALENT BOND Chemical Bond EXAMPLES OF COORDINATE Molecules of chemical substances are made of two or more COMPOUNDS OR IONS atoms joined together by some force, acting between them. This DIFFERENCES BETWEEN IONIC force which results from the interaction between the various atoms AND COVALENT BONDS that go to form a stable molecule, is referred to as a Chemical POLAR COVALENT BONDS Bond. HYDROGEN BONDING (H-bonding) A chemical bond is defined as a force that acts between two or more atoms to hold them together as a stable molecule. EXAMPLES OF HYDROGEN- BONDED COMPOUNDS As we will study later, there are three different types of bonds recognised by chemists : CHARACTERISTICS OF HYDROGEN-BOND COMPOUNDS (1) Ionic or Electrovalent bond EXCEPTIONS TO THE OCTET (2) Covalent bond RULE (3) Coordinate covalent bond VARIABLE VALENCE There is a fourth type of bond, namely, the metallic bond which we will consider later in this chapter. METALLIC BONDING Definition of Valence GEOMETRIES OF MOLECULES The term valence (or valency) is often used to state the VSEPR THEORY potential or capacity of an element to combine with other elements. 151 152 5 PHYSICAL CHEMISTRY At one time, it was useful to define valence of an element as : the number of hydrogen atoms or twice the number of oxygen atoms with which that element could combine in a binary compound (containing two different elements only). In hydrogen chloride (HCl), one atom of chlorine is combined with one atom of hydrogen and the valence of chlorine is 1. In magnesium oxide (MgO), since one atom of magnesium holds one atom of oxygen, the valence of magnesium is 2. By the above definition, we would assign a valence of 2 to sulphur in H2S, but 4 to sulphur in SO2. Some elements have fractional valence in certain compounds, while there are elements that have variable valencies. The concept of valence as a mere number could not explain these facts. This concept, in fact, was very confusing and has lost all value. As already stated, there are three different type of bonds that are known to join atoms in molecules. Although no precise definition of valence is possible, we can say that : Valence is the number of bonds formed by an atom in a molecule. Valence Electrons The electrons in the outer energy level of an atom are the ones that can take part in chemical bonding. These electrons are, therefore, referred to as the valence electrons. The electronic configuration of Na is 2, 8, 1 and that of Cl is 2, 8, 7. Thus sodium has one valence electron and chlorine 7. It is important to remember that for an A group element of the periodic table (H, O, K, F, Al etc.) the group number is equal to the number of valence electrons. Bonding and Non-bonding Electrons The valence electrons actually involved in bond formation are called bonding electrons. The remaining valence electrons still available for bond formation are referred to as non-bonding electrons. Thus : Bonding electrons Cl Cl Nonbonding electrons Lewis Symbols of Elements A Lewis symbol of an element consists of an element’s symbol and surrounding dots to represent the number of valence electrons. In this notation, the symbol of an element represents the nucleus plus the inner normally filled levels (or shells) of the atom. For illustration, the symbol Na stands for the nucleus of sodium atom plus 2, 8 electrons in the inner two levels. Figure 5.1 The Lewis symbol Na represents the nucleus and the electrons arranged in the inner two levels as 2, 8, minus the valence electrons. CHEMICAL BONDING - LEWIS THEORY 153 To represent a Lewis symbol for an element, write down the symbol of the element and surround the symbol with a number of dots (or crosses) equal to the number of valence electrons. The position of dots around the symbol is not really of any significance. The bonding electrons are shown at appropriate positions, while the rest of the electrons are generally given in pairs. The Lewis symbols for hydrogen, chlorine, oxygen and sulphur may be written as : H Cl O S The structural formulae of compounds built by union of Lewis symbols for the component atoms, are referred to as Electron-dot formulas, or Electron-dot structures or Lewis structures. For this purpose, the valence electrons actually involved in bond formation may be shown by crosses (x) or dots (.) for the sake of distinction. Now we will proceed to discuss the common types of chemical bonds in the light of the electronic theory of valence. ELECTRONIC THEORY OF VALENCE As Bohr put forward his model of the atom so electronic configuration of elements was known. G.N. Lewis and W. Kossel, working independently, used this knowledge to explain ‘why atoms joined to form molecules’. They visualised that noble gas atoms had a stable electronic configuration, while atoms of all other elements has unstable or incomplete electronic configuration. In 1916, they gave the electronic theory of valence. It states that : In chemical bond formation, atoms interact by losing, gaining, or sharing of electrons so as to acquire a stable noble gas configuration. Each noble gas, except helium, has a valence shell of eight electrons (Table 5.1). TABLE 5.1. ELECTRONIC CONFIGURATION OF NOBLE GASES Noble gas At. No. Electrons in principal shells He 2 2 Ne 10 2, 8 Ar 18 2, 8, 8 Kr 36 2, 8, 18, 8 Xe 54 2, 8, 18, 18, 8 Rn 86 2, 8, 18, 32, 18, 8 While atoms of noble gases possess a stable outer shell of eight electrons or octet, atoms of most other elements have incomplete octets. They may have less than 8 electrons or in excess. Thus, the electronic theory or valence could well be named as the Octet theory of Valence. It may be stated as : Atoms interact by electron-transfer or electron-sharing, so as to achieve the stable outer shell of eight electrons. The tendency for atoms to have eight electrons in the outer shell is also known as the Octet Rule or the Rule of Eight. Since helium has two electrons in the outer shell, for hydrogen and lithium, having one and three (2, 1) electrons respectively, it is the Rule of two which will apply. We will see later in this chapter that there are quite a few exceptions to the rule of eight in covalent compounds. IONIC BOND This type of bond is established by transfer of an electron from one atom to another. Let us consider a general case when an atom A has one electron in the valence shell and another atom B has seven electrons. A has one electron in excess and B has one electron short than the stable octet. Therefore, A transfers an electron to B and in this transaction both the atoms acquire a stable electron- octet. The resulting positive ion (cation) and negative ion (anion) are held together by electrostatic attraction. 154 5 PHYSICAL CHEMISTRY LINUS CARL PAULING Linus Pauling won the Nobel Prize in Chemistry in 1954 for his work on chemical bonding. He also received the Nobel Peace Prize in 1962 for his campaign against nuclear testing. Linus Carl Pauling (February 28, 1901 – August 19, 1994) was an American quantum chemist and biochemist. Pauling is widely regarded as the premier chemist of the twentieth century. He pioneered the application of quantum mechanics to chemistry and in 1954 was awarded the Nobel Prize in chemistry for his work describing the nature of chemical bonds. He also made important contributions to crystal and protein structure determination, and was one of the founders of molecular biology. He came near to discovering the “double helix,” the ultrastructure of DNA, when Watson and Crick made the discovery in 1953. Pauling received the Nobel Peace Prize in 1962 for his campaign against above-ground nuclear testing, and is the only person to win two unshared Nobel prizes. Later in life, he became an advocate for greatly increased consumption of vitamin C and other nutrients. He generalized his ideas to define orthomolecular medicine, which is still regarded as unorthodox by conventional medicine. He popularized his concepts, analyses, research and insights in several successful but controversial books centered around vitamin C and orthomolecular medicine. + + A B A + B or A + B (1 Valence (7 Valence Cation Anion electron) electrons) Electrovalent bond + + + A + B A B or A B The electrostatic attraction between the cation (+) and anion (–) produced by electron-transfer constitutes an Ionic or Electrovalent bond. The compounds containing such a bond are referred to as Ionic or Electrovalent Compounds. CONDITIONS FOR FORMATION OF IONIC BOND The conditions favourable for the formation of an ionic bond are : (1) Number of valence electrons The atom A should possess 1, 2 or 3 valence electrons, while the atom B should have 5, 6 or 7 valence electrons. The elements of group IA, IIA and IIIA satisfy this condition for atom A and those of groups VA, VIA, and VIIA satisfy this condition for atom B. CHEMICAL BONDING - LEWIS THEORY 155 (2) Net lowering of Energy To form a stable ionic compound, there must be a net lowering of the energy. In other words energy must be released as a result of the electron transfer and formation of ionic compound by the following steps : (a) The removal of electron from atom A (A – e– → A+) requires input of energy, which is the ionization energy (IE). It should be low. (b) The addition of an electron to B (B + e– → B – ) releases energy, which is the electron affinity of B (EA). It should be high. (c) The electrostatic attraction between A+ and B – in the solid compound releases energy, which is the electrical energy. It should also be high. If the energy released in steps (b) and (c) is greater than the energy consumed in step (a), the overall process of electron transfer and formation of ionic compound results in a net release of energy. Therefore, ionisation of A will occur and the ionic bond will be formed. For example, in case of formation of sodium chloride (NaCl), we have Na – e– ⎯⎯ → Na + – 119 kcal Cl + e– ⎯⎯ → Cl – + 85 kcal + Na + + Cl – ⎯⎯ → Na Cl – + 187 kcal The net energy released is 187 + 85 – 119 = 153 kcal. Since the overall process results in a lowering of energy, the ionic bond between Na and Cl will be formed. (3) Electronegativity difference of A and B From the line of argument used in (2), we can say that atoms A and B if they have greatly different electronegativities, only then they will form an ionic bond. In fact, a difference of 2 or more is necessary for the formation of an ionic bond between atoms A and B. Thus Na has electronegativity 0.9, while Cl has 3.0. Since the difference is (3.0 – 0.9) = 2.1, Na and Cl will form an ionic bond. FACTORS GOVERNING THE FORMATION OF IONIC BOND (1) Ionisation Energy The ionisation energy of the metal atom which looses electron(s) should be low so that the formation of +vely charged ion is easier. Lower the ionisation energy greater will be the tendency of the metal atom of change into cation and hence greater will be the ease of formation of ionic bond. That is why alkali metals and alkaline earth metals form ionic bonds easily. Out of these two, alkali metals form ionic bonds easily as compared to alkaline earth metals. In a group the ionisation energy decreases as we move down the group and therefore, the tendency to form ionic bond increases in a group downward. Due to this reason Cs is the most electropositive atom among the alkali metals. (2) Electron Affinity The atom which accepts the electron and changes into anion should have high electron affinity. Higher the electron affinity more is the energy released and stable will be the anion formed. The elements of group VI A and VII A have, in general, higher electron affinity and have high tendency to form ionic bonds. Out of these two, the elements of group VII A (halogens) are more prone to the formation of ionic bond than the elements of group VI A. In moving down a group the electron affinity decreases and, therefore, the tendency to form ionic bond also decreases. (3) Lattice Energy After the formation of cations and anions separately, they combine to form ionic compound. 156 5 PHYSICAL CHEMISTRY + + A + B A B + Lattice energy In this process, energy is released. It is called Lattice Energy. It may be defined as “the amount of energy released when one mole of an ionic compound is formed from its cations and anions.” Greater the lattice energy, greater the strength of ionic bond. The value of lattice energy depends upon the following two factors : (a) Size of the ions In order to have the greater force of attraction between the cations and anions their size should be small as the force of attraction is inversely proportional to the square of the distance between them. (b) Charge on Ions Greater the charge on ions greater will be the force of attraction between them and, therefore, greater will be the strength of the ionic bond. Necessary for the formation of an ionic bond between atoms A and B. Thus Na has electronegativity 0.9, while Cl has 3.0. Since the difference is (3.0 – 0.9) = 2.1, Na and Cl will form an ionic bond. SOME EXAMPLES OF IONIC COMPOUNDS Here we will discuss the formation of Lewis formula or Electron dot formula of some binary ionic compounds, for illustration. Sodium Chloride, NaCl A simple sodium chloride molecule is formed from an atom of sodium (Na) and one atom of chlorine (Cl). Na (2, 8, 1) has one valence electron, while Cl (2, 8, 7) has seven. Na transfers its valence electron to Cl, and both achieve stable electron octet. Thus Na gives Na+ and Cl gives Cl– ion, and the two are joined by an ionic bond. - Na + Cl Na+ + Cl or Na+Cl- Sodium 2,8,1 2,8,7 2,8 2,8,8 chloride Ionic Compounds Exist as Crystals. The (+) and (–) ions attract each other with electrostatic force that extends in all directions. This means that ions will be bonded to a number of oppositely charged ions around them. Therefore in solid state, single ionic molecules do not exist as such. Rather many (+) and (–) ions are arranged systematically in an alternating cation-anion pattern called the crystal lattice. The crystal lattice of NaCl is shown in Fig. 5.2. It will be noticed that here a large number of Na+ and Cl– ions are arranged in an orderly fashion so as to form a cubic crystal. Each Na+ ion is surrounded by 6 Cl– ions and each Cl– ion is surrounded by 6 Na+ ions. This makes a network of Na+ and Cl– ions which are tightly held together by electrostatic forces between them. CHEMICAL BONDING - LEWIS THEORY 157 Although discrete molecules Na+Cl– do not exist in the solid form of ionic compounds, independent molecules do exist in the vapour form of such compounds. Figure 5.2 Ionic crystal of Sodium Chloride. Magnesium Chloride, Mg 2+ Cl12- (MgCl2) Magnesium (Mg) has two valence electrons, while chlorine (Cl) has seven. The magnesium atom transfers its two electrons, one to each chlorine atom, and thus all the three atoms achieve the stable octet. In this way Mg atom gives Mg2+ ion and the two Cl atoms give 2Cl1–, forming Mg 2 + Cl1– 2 (or MgCl2). 2+ - - Mg Cl Cl Mg Cl Cl 2, 8, 2 2, 8, 7 2, 8, 7 2, 8 2, 8, 8 2, 8, 8 2+ - 2+ or Mg 2Cl Mg 2Cl or Mg Cl 2 Calcium Oxide, Ca2+O2– (CaO) Calcium (Ca) has two valence electrons, while oxygen (O) has six. Calcium atom transfers its two valence electrons to the same oxygen atom. Thus both Ca and O achieve the stable electron-octet, forming Ca2+ and O2– ions. Thus is obtained the molecule of calcium oxide, Ca2+O2–. 158 5 PHYSICAL CHEMISTRY xx 2+ 2 2+ 2 Ca O Ca + O or Ca O Calcium 2, 8, 8, 2 2, 6 2, 8, 8 2, 8 oxide Aluminium Oxide, Al32 + O 32 – (Al2O3) Here the aluminium atom (Al) has three electrons in the valence shell (2, 8, 3), while oxygen has six (2, 6). Two atoms of aluminium transfer their six electrons to three oxygen atoms. Thus are the electron- octets of the two Al atoms and three O atoms achieved. The two Al atoms deprived of three electrons each, give 2Al3+ ions, while the three O atoms having gained two electrons each give 3O2– ions. In this way, we get Al 32 + O 32 – or Al2O3. CHARACTERISTICS OF IONIC COMPOUNDS The ionic compounds are made of (+) and (–) ions held by electrostatic forces in a crystal lattice. Each ion is surrounded by the opposite ions in alternate positions in a definite order in all directions. This explains the common properties of ionic compounds. 2 x O x O 3+ Al Al 2 x O + x O 3+ Al Al 2 x O x O 3+ 2 3+ 2 or 2Al 3O 2Al 3O or 2Al2 O3 Aluminium oxide (1) Solids at Room Temperature On account of strong electrostatic forces between the opposite ions, these ions are locked in their allotted positions in the crystal lattice. Since they lack the freedom of movement characteristic of the liquid state, they are solids at room temperature. (2) High Melting Points Ionic compounds have high melting points (or boiling points). Since the (+) and (–) ions are tightly held in their positions in the lattice, only at high temperature do the ions acquire sufficient kinetic energy to overcome their attractive forces and attain the freedom of movement as in a liquid. Thus ionic compounds need heating to high temperatures before melting. (3) Hard and brittle The crystals of ionic substances are hard and brittle. Their hardness is due to the strong electrostatic forces which hold each ion in its allotted position. These crystals are made of layers of (+) and (–) ions in alternate positions so that the opposite ions in the various parallel layers lie over each other. When external force is applied to a layer of ions (Fig. 5.3), with respect to the next, even a slight shift brings the like ions in front of each other. The (+) and (–) ions in the two layers thus repel each other and fall apart. The crystal cleaves here. CHEMICAL BONDING - LEWIS THEORY 159 Figure 5.3 (a) Two layers of (+) and (–) ions in a crystal. (b) When force is applied to one layer it slips over the other so that similar ions come above one another and electrical repulsions between them cause cleavage of the crystal. (4) Soluble in water When a crystal of an ionic substance is placed in water, the polar water molecules detach the (+) and (–) ions from the crystal lattice by their electrostatic pull. These ions then get surrounded by water molecules and can lead an independent existence and are thus dissolved in water. By the same reason, non-polar solvents like benzene (C 6H 6) and hexane (C 6H 14 ) will not dissolve ionic compounds. Figure 5.4 Solvation of NaCl in water. (5) Conductors of electricity Solid ionic compounds are poor conductors of electricity because the ions are fixed rigidly in their positions. In the molten state and in water solutions, ions are rendered free to move about. Thus molten ionic compounds or their aqueous solutions conduct a current when placed in an electrolytic cell. (6) Do not exhibit isomerism The ionic bond involving electrostatic lines of force between opposite ions, is non-rigid and non- directional. The ionic compounds, therefore, are incapable of exhibiting stereoisomerism. (7) Ionic reactions are fast Ionic compounds give reactions between ions and these are very fast. 160 5 PHYSICAL CHEMISTRY COVALENT BOND The electron transfer theory could not explain the bonding in molecules such as H2, O2, Cl2 etc., and in organic molecules, that had no ions. It was G.N. Lewis who suggested that two atoms could achieve stable 2 or 8 electrons in the outer shell by sharing electrons between them. Let us consider a general case where an atom A has one valence electron and another atom B has seven valence electrons. As they approach each other, each atom contributes one electron and the resulting electron pair fills the outer shell of both the atoms. Thus A acquires stable 2 electrons and B, 8 electrons in the outer shell. The shared pair is indicated by a dash (–) between the two bonded atoms. A shared pair of electrons constitutes a Covalent bond or Electron-pair bond. In fact, the positive nuclei of atoms A and B are pulled towards each other by the attraction of the shared electron pair. At the same time, the nuclei of two atoms also repel each other as do the two electrons. It is the net attractive force between the shared electrons and the nuclei that holds the atoms together. Thus an alternative definition of a covalent bond would be : The attractive force between atoms created by sharing of an electron-pair. The compounds containing a covalent bond are called covalent compounds. CONDITIONS FOR FORMATION OF COVALENT BOND The conditions favourable for the formation of covalent bonds are : (1) Number of valence electrons Each of the atoms A and B should have 5, 6 or 7 valence electrons so that both achieve the stable octet by sharing 3, 2 or 1 electron-pair. H has one electron in the valence shell and attains duplet. The non-metals of groups VA, VIA and VIIA respectively satisfy this condition. (2) Equal electronegativity The atom A will not transfer electrons to B if both have equal electronegativity, and hence electron sharing will take place. This can be strictly possible only if both the atoms are of the same element. (3) Equal sharing of electrons The atoms A and B should have equal (or nearly equal) electron affinity so that they attract the bonding electron pair equally. Thus equal sharing of electrons will form a nonpolar covalent bond. Of course, precisely equal sharing of electrons will not ordinarily occur except when atoms A and B are atoms of the same element, for no two elements have exactly the same electron affinity. SOME EXAMPLES OF COVALENT COMPOUNDS The construction of Lewis structures of simple covalent compounds will be discussed. Hydrogen, H2 Hydrogen molecule is made of two H atoms, each having one valence electron. Each contributes an electron to the shared pair and both atoms acquire stable helium configuration. Thus stable H2 molecule results. CHEMICAL BONDING - LEWIS THEORY 161 Chlorine, Cl2 Each Cl atom (2, 8, 7) has seven valence electrons. The two Cl atoms achieve a stable electron octet by sharing a pair of electrons. Water, H2O Oxygen atom (2, 6) has six valence electrons and can achieve the stable octet by sharing two electrons, one with each H atom. Thus Lewis structure of water can be written as : Sharing electrons Unshared electron pair xx xx x x H x Ox H H xOx H or H O H xx xx x x Water Ammonia, NH3 Nitrogen atom (2, 5) has five valence electrons and can achieve the octet by sharing three electrons, one each with three H atoms. This gives the following Lewis structure for ammonia : Sharing electrons Unshared electron pair xx x xx x x x H x Nx H H N H or N H x x H H H Ammonia Methane, CH4 Carbon atom (2, 4) has four electrons in the valence shell. It can achieve the stable octet by sharing these electrons with four H atoms, one with each H atom. Thus the Lewis structure of methane can be written as : Sharing electrons C C C Methane 162 5 PHYSICAL CHEMISTRY EXAMPLES OF MULTIPLE COVALENT COMPOUNDS In many molecules, we find that in order to satisfy the octet, it becomes necessary for two atoms to share two or three pairs of electrons between the same two atoms. The sharing of two pairs of electrons is known as a Double bond and the sharing of three pairs of electrons a Triple bond. Let us consider some examples of compounds containing these multiple covalent bonds in their molecules. Oxygen, O2 The conventional Lewis structure of oxygen is written by sharing of two pairs of electrons between two O atoms (2, 6). In this way both the O atoms achieve the octet. Sharing electrons Double bond xx xx xx x x O xx O x x O xx O or x x O O Oxygen 2, 8, 6 2, 8, 6 2, 8, 8 2, 8, 8 The above structure of oxygen implies that all the electrons in oxygen, O2, are paired whereby the molecule should be diamagnetic. However, experiment shows that O2 is paramagnetic with two unpaired electrons. This could be explained by the structure. O O Although writing Lewis structures work very well in explaining the bonding in most simple molecules, it should be kept in mind that it is simply the representation of a theory. In this case, the theory just doesn’t work. Nitrogen, N2 The two atoms of nitrogen (2, 5), each having five electrons in the valence shell, achieve the octet by sharing three electron pairs between them. N + N N N or N N Nitrogen (3 Electrons shared by each N atom) Carbon Dioxide, CO2 Carbon (2, 4) has four valence electrons. It shares two electrons with each O atom (having six valence electrons). Thus the C atom and both the O atoms achieve their octet. x O + x x C xx + O O x C xx O or O C O Carbon dioxide CHARACTERISTICS OF COVALENT COMPOUNDS While the atoms in a covalent molecule are firmly held by the shared electron pair, the individual molecules are attracted to each other by weak van der Waals forces. Thus the molecules can be separated easily as not much energy is required to overcome the intermolecular attractions. This explains the general properties of covalent compounds. (1) Gases, liquids or solids at room temperature The covalent compounds are often gases, liquids or relatively soft solids under ordinary conditions. This is so because of the weak intermolecular forces between the molecules. (2) Low melting points and boiling points Covalent compounds have generally low melting points (or boiling points). The molecules are CHEMICAL BONDING - LEWIS THEORY 163 held together in the solid crystal lattice by weak forces. On application of heat, the molecules are readily pulled out and these then acquire kinetic energy for free movement as in a liquid. For the same reason, the liquid molecules are easily obtained in the gaseous form which explains low boiling points of covalent liquids. (3) Neither hard nor brittle While the ionic compounds are hard and brittle, covalent compounds are neither hard nor brittle. There are weak forces holding the molecules in the solid crystal lattice. A molecular layer in the crystal easily slips relative to other adjacent layers and there are no ‘forces of repulsion’ like those in ionic compounds. Thus the crystals are easily broken and there is no sharp cleavage between the layers on application of external force. (4) Soluble in organic solvents In general, covalent compounds dissolve readily in nonpolar organic solvents (benzene, ether). The kinetic energy of the solvent molecules easily overcomes the weak intermolecular forces. Covalent compounds are insoluble in water. Some of them (alcohols, amines) dissolve in water due to hydrogen-bonding. (5) Non-conductors of electricity Since there are no (+) or (–) ions in covalent molecules, the covalent compounds in the molten or solution form are incapable of conducting electricity. (6) Exhibit Isomerism Covalent bonds are rigid and directional, the atoms being held together by shared electron pair and not by electrical lines of force. This affords opportunity for various spatial arrangements and covalent compounds exhibit stereoisomerism. (7) Molecular reactions The covalent compounds give reactions where the molecule as a whole undergoes a change. Since there are no strong electrical forces to speed up the reaction between molecules, these reactions are slow. CO-ORDINATE COVALENT BOND In a normal covalent bond, each of the two bonded atoms contributes one electron to make the shared pair. In some cases, a covalent bond is formed when both the electrons are supplied entirely by one atom. Such a bond is called co-ordinate covalent or dative bond. It may be defined as : a covalent bond in which both electrons of the shared pair come from one of the two atoms (or ions). The compounds containing a coordinate bond are called coordinate compounds. If an atom A has an unshared pair of electrons (lone pair) and another atom B is short of two electrons than the stable number, coordinate bond is formed. A donates the lone pair to B which accepts it. Thus both A and B achieve the stable 2 or 8 electrons, the lone pair being held in common. The atom A which donates the lone pair is called the donor, while B which accepts it the acceptor. The bond thus established is indicated by an arrow pointing from A to B. Although the arrow head 164 5 PHYSICAL CHEMISTRY indicates the origin of the electrons, once the coordinate bond is formed it is in no way different from an ordinary covalent bond. The molecule or ion that contains the donor atom is called the ligand. SOME EXAMPLES OF COORDINATE COMPOUNDS OR IONS Lewis structures of some common molecules or ions containing a coordinate covalent bond are listed below. Ammonium ion, NH+4 In ammonia molecule, the central N atom is linked to three H atoms and yet N has an unshared pair of electrons. The H+ ion furnished by an acid has no electron to contribute and can accept a pair of electrons loaned by N atom. Thus, NH3 donates its unshared electrons to H+ forming ammonium ion. + H H H + + H N H H N H or H N H Hydrogen H ion H H Ammonia Ammonium ion molecule (N has a lone pair) All the N–H bonds in NH +4 are identical, once the coordinate bond N→H+ is established. Hydronium ion, H3O+ The oxygen atom in water molecule is attached to two H atoms by two covalent bonds. There are still two unshared pairs of electrons with the O atom. The O atom donates one of these pairs of electrons to H+ ion and the hydronium ion is thus formed. + + H O H+ H O H or H3O H H Hydronium ion Fluoroborate ion, B F4– It is formed when a boron trifluoride molecule (BF3) shares a pair of electrons supplied by fluoride ion (F–). F F F B F F B F or BF4 F Fluoride F ion Boron Fluoroborate trifluoride ion (B acts as Acceptor) Addition compound of NH3 with BCl3 The N atom of ammonia molecule (NH3) has lone pair while B atom in boron trichloride (BCl3) is short of two electrons than stable octet. An addition compound is formed as the N atom donates its lone pair to B atom of BCl3. CHEMICAL BONDING - LEWIS THEORY 165 H Cl H Cl H N B Cl H N B Cl H Cl H Cl (N is Donor) (B is Acceptor) Addition compound Nitromethane, CH3NO2 The Lewis structure of nitromethane is shown below. Here the N atom has five valence electrons, three of which are used in forming a covalent bond with C atom and two covalent bonds with O atom. The N atom is still left with two unshared electrons which are donated to another O atom. H H O O x x H C N or H C N x O H O H Nitromethane Sulphur dioxide, SO2, and Sulphur trioxide, SO3 Sulphur achieves its octet by forming two covalent bonds with one O atom, giving SO. The S atom in SO has two lone pairs, one of which is shared with a second O atom to form sulphur dioxide, SO2. The S atom in SO2 still has one lone pair which it donates to a third O atom forming the sulphur trioxide (SO3) molecule. xx x x x O x S xx or O S O Sulphur dioxide O x x O S O O O S O Sulphur trioxide Aluminium Chloride, Al2Cl6 Aluminium atom has three valence electrons which it shares with three Cl atoms, forming three covalent bonds. Thus the Al atom acquires six electrons in its outer shell. Now Cl atom has three lone pairs, one of which is donated to the Al atom of another molecule AlCl3. Thus both Al atoms achieve octet and stable Al2Cl6 results. Cl Cl Cl Al Al Cl Cl Cl 2– Sulphate ion, SO4 Sulphur has six valence electrons (2, 8, 6) and achieves the octet by gaining two electrons from metal atoms (say two Na atoms). The four pairs of electrons around the S atom are then donated to four oxygen atoms each of which has six electrons. Thus the Lewis structure for SO24 – ion may be written as : 166 5 PHYSICAL CHEMISTRY 2 O 2 O xx O xx S xx O or O S O xx O O S gains two electrons Sulphate ion from metal atoms and completes octet Ozone, O3 Oxygen molecule is made of two oxygen atoms joined by two covalent bonds. Each O atom in O2 has two unshared pairs of electrons. When one pair of these is donated to a third O atom which has only six electrons, a coordinate bond is formed. Thus the Lewis structure of ozone may be represented as : xx xx x x O O xx + O O O O Oxygen Ozone (2 Atoms of O) Carbon Monoxide, CO Carbon atom has four valence electrons while oxygen atom has six. By forming two covalent bonds between them, O atom achieves octet but C atom has only six electrons. Therefore O donates an unshared pair of electron to C, and a coordinate covalent bond is established between the two atoms. Lewis structure of CO may be written as : xx xx x C + x O xx C x x O xx C O COMPARISON OF IONIC AND COVALENT BONDS Ionic Bond Covalent Bond 1. Formed by transfer of electrons from a metal 1. Formed by sharing of electrons between to a non-metal atom. nonmetal atoms. 2. Consists of electrostatic force between (+) 2. Consists of a shared pair of electrons and (–) ions. between atoms. 3. Non-rigid and non-directional; cannot cause 3. Rigid and directional : causes isomerism. stereoisomerism. Properties of Compounds Properties of Compounds 1. Solids at room temperature. 1. Gases, liquids or soft solids. 2. High melting and boiling points. 2. Low melting and boiling points. 3. Hard and brittle. 3. Soft, much readily broken 4. Soluble in water but insoluble in organic 4. Insoluble in water but soluble in organic solvents. solvents. 5. Conductors of electricity 5. Non-conductors of electricity. 6. Undergo ionic reactions which are fast. 6. Undergo molecular reactions which are slow. CHEMICAL BONDING - LEWIS THEORY 167 POLAR COVALENT BONDS In the H2 or Cl2 molecule, the two electrons constituting the covalent bond are equally shared by the two identical nuclei. Due to even distribution of (+) and (–) charge, the two bonded atoms remain electrically neutral. Such a bond is called nonpolar covalent bond. However, when two different atoms are joined by a covalent bond as in HCl, the electron pair is not shared equally. Nonpolar covalent Polar covalent bond bond Cl Cl H Cl Bonding pair ( : ) Bonding pair ( : ) equally shared unequally shared Due to a greater attraction of one nucleus (Cl) for the electrons, the shared pair is displaced towards it. This makes one end of the bond partially positive (δ+ ) and the other partially negative (δ–). H Cl or H Cl A covalent bond in which electrons are shared unequally and the bonded atoms acquire a partial positive and negative charge, is called a polar covalent bond. A molecule having partial positive and negative charge separated by a distance is commonly referred to as a Dipole (two poles). The dipole of a bond is indicated by an arrow from positive to negative end with a crossed tail as shown above in HCl molecule. Since two atoms of different elements do not have exactly the same attraction for electrons in a bond, all bonds between unlike atoms are polar to some extent. The amount of polarity of a bond is determined by the difference of electronegativity (or tendency to attract electrons) of the two bonded atoms. The greater the difference of electronegativity between two atoms, greater the polarity. A graph showing the % age ionic character and difference in electronegativity between the two atoms is shown in Fig. 5.5. As a matter of fact, if this difference is around 1.9 and 2.9, the bond is generally ionic, meaning that one atom has gained complete control of the electron pair in the bond. The percentage ionic character of a bond can be calculated by using the equation %age ionic character = 16 [XA – XB] + 3.5 [XA – XB]2 This equation was given by Hannay and Smith. 168 5 PHYSICAL CHEMISTRY 100% Ionic 100 character 80 % Ionic character 60 50% Ionic character 40 20 0 0 0.5 1.0 1.5 2.0 2.5 3.0 3.5 Difference in electronegativity Figure 5.5 Graph between % ionic character and difference in electronegativity. SOLVED PROBLEM. Calculate the percentage ionic character of C–Cl bond in CCl4 if the electronegativities of C and Cl are 3.5 and 3.0 respectively. SOLUTION %age ionic character = 16 [XA – XB] +3.5 [XA + XB]2 Give XA = 3.5 and XB = 3.0 ∴ %age ionic character = 16 (3.5 – 3.0) + 3.5 (3.5 – 3.0)2 = 8.0 + 0.875 = 8.875% Examples of Polar Covalent Bonds Water molecule (H2O) contains two O–H covalent bonds. The electronegativity of O is 3.5 and that of H is 2.1. Thus both the bonds are polar and water has a polar molecule. δ+ H Polar covalent δ bond O N δ δ+ δ+ δ+ δ+ H H H H Water Ammonia In ammonia molecule, there are three N–H bonds. The electronegativity of N is 3.0 and that of H is 2.1. Therefore all the N–H bonds are polar and ammonia has a polar molecule. The electronegativity of fluorine (F) is 4.0 and that of H is 2.1. The difference of electronegativities being very great, the molecule H–F has a strong dipole. CHEMICAL BONDING - LEWIS THEORY 169 HYDROGEN BONDING (H-Bonding) When hydrogen (H) is covalently bonded to a highly electronegative atom X (O, N, F), the shared electron pair is pulled so close to X that a strong dipole results. X H or X H Dipole Since the shared pair is removed farthest from H atom, its nucleus (the proton) is practically exposed. The H atom at the positive end of a polar bond nearly stripped of its surrounding electrons, exerts a strong electrostatic attraction on the lone pair of electrons around X in a nearby molecule. Thus : Electrostatic attraction X H + X H X H X H Hydrogen bond or X H X H The electrostatic attraction between an H atom covalently bonded to a highly electronegative atom X and a lone pair of electrons of X in another molecule, is called Hydrogen Bonding. Hydrogen bond is represented by a dashed or dotted line. POINTS TO REMEMBER (1) Only O, N and F which have very high electronegativity and small atomic size, are capable of forming hydrogen bonds. (2) Hydrogen bond is longer and much weaker than a normal covalent bond. Hydrogen bond energy is less than 10 kcal/mole, while that of covalent bond is about 120 kcal/mole. (3) Hydrogen bonding results in long chains or clusters of a large number of ‘associated’ molecules like many tiny magnets. (4) Like a covalent bond, hydrogen bond has a preferred bonding direction. This is attributed to the fact that hydrogen bonding occurs through p orbitals which contain the lone pair of electrons on X atom. This implies that the atoms X–H...X will be in a straight line. CONDITIONS FOR HYDROGEN BONDING The necessary conditions for the formation of hydrogen bonding are (1) High electronegativity of atom bonded to hydrogen The molecule must contain an atom of high electronegativity such as F, O or N bonded to hydrogen atom by a covalent bond. The examples are HF, H2O and NH3. (2) Small size of Electronegative atom The electronegative atom attached to H-atom by a covalent bond should be quite small. Smaller the size of the atom, greater will be the attraction for the bonded electron pair. In other words, the polarity of the bond between H atom and electronegative atom should be high. This results in the formation of stronger hydrogen bonding. For example, N and Cl both have 3.0 electronegativity. But hydrogen bonding is effective in NH3 in comparison to that in HCl. It is due to smaller size of N atom than Cl atom. 170 5 PHYSICAL CHEMISTRY EXAMPLES OF HYDROGEN-BONDED COMPOUNDS When hydrogen bonding occurs between different molecules of the same compound as in HF, H2O and NH3, it is called Intermolecular hydrogen bonding. If the hydrogen bonding takes place within single molecule as in 2-nitrophenol, it is referred to as Intramolecular hydrogen bonding. We will consider examples of both types. Hydrogen Fluoride, HF The molecule of HF contains the strongest polar bond, the electronegativity of F being the highest of all elements. Therefore, hydrogen fluoride crystals contain infinitely long chains of H–F molecules in which H is covalently bonded to one F and hydrogen bonded to another F. The chains possess a zig-zag structure which occurs through p orbitals containing the lone electron pair on F atom. Hydrogen bond H F H F H F Hydrogen fluoride molecules Water, H2O In H2O molecule, two hydrogen atoms are covalently bonded to the highly electronegative O atom. Here each H atom can hydrogen bond to the O atom of another molecule, thus forming large chains or clusters of water molecules. Hydrogen bond H O H O H O H O H H H H Water Liquid water molecule Each O atom still has an unshared electron pair which leads to hydrogen bonding with other water molecules. Thus liquid water, in fact, is made of clusters of a large number of molecules. Ammonia, NH3 In NH3 molecules, there are three H atoms covalently bonded to the highly electronegative N atom. Each H atom can hydrogen bond to N atom of other molecules. CHEMICAL BONDING - LEWIS THEORY 171 Hydrogen bond H H H H H N H N H N H N H H H H Ammonia molecule 2-Nitrophenol Here hydrogen bonding takes place within the molecule itself as O–H and N–H bonds are a part of the same one molecule. TYPES OF HYDROGEN-BONDING Hydrogen bonding is of two types : (1) Intermolecular Hydrogen bonding This type of hydrogen bonding is formed between two different molecules of the same or different substances e.g. hydrogen bonding in HF, H2O, NH3 etc. It is shown in the following diagram (Fig. 5.6). Hydrogen bond H F H F H F Hydrogen fluoride molecule Hydrogen bond Hydrogen H H H bond O H O H O H N H N H N H H H H H H Water molecule Ammonia molecule Figure 5.6 Intermolecular hydrogen bonding in HF, H2 O and NH3. 172 5 PHYSICAL CHEMISTRY This type of hydrogen bonding results in the formation of associated molecules. Generally speaking, the substances with intermolecular hydrogen bonding have high melting points, boiling points, viscosity, surface tension etc. (2) Intramolecular Hydrogen bonding This type of hydrogen bonding is formed between the hydrogen atom and the electronegative atom present within the same molecule. It results in the cyclisation of the molecule. Molecules exist as discrete units and not in associated form. Hence intramolecular hydrogen bonding has no effect on physical properties like melting point, boiling point, viscosity, surface tension, solubility etc. For example intramolecular hydrogen bonding exists in o-nitrophenol, 2-nitrobenzoic acid etc. as shown below : Figure 5.7 Intramolecular hydrogen bonding. CHARACTERISTICS OF HYDROGEN-BONDED COMPOUNDS (1) Abnormally high boiling and melting points The compounds in which molecules are joined to one another by hydrogen bonds, have unusually high boiling and melting points. This is because here relatively more energy is required to separate the molecules as they enter the gaseous state or the liquid state. Thus the hydrides of fluorine (HF), oxygen (H2O) and nitrogen (NH3) have abnormally high boiling and melting points compared to other hydrides of the same group which form no hydrogen bonds. In Fig. 5.8 are shown the boiling points and melting points of the hydrides of VIA group elements plotted against molecular weights. It will be noticed that there is a trend of decrease of boiling and melting points with decrease of molecular weight from H2Te to H2S. But there is a sharp increase in case of water (H2O), although it has the smallest molecular weight. The reason is that the molecules of water are ‘associated’ by hydrogen bonds between them, while H2Te, H2Se and H2S exist as single molecules since they are incapable of forming hydrogen bonds. CHEMICAL BONDING - LEWIS THEORY 173 o H2 O 100 Melting Boiling points Temperature oC points H2 Te H2 Se o H2 O 0 H2 S H2 Te H2 Se H2 S o –100 0 60 120 Molecular weights Figure 5.8 Boiling and melting point curves of the hydrides of VIA group showing abrupt increase for water (H2O) although it has the lowest molecular weight. (2) High solubilities of some covalent compounds The unexpectedly high solubilities of some compounds containing O, N and F, such as NH3 and CH3OH in certain hydrogen containing solvents are due to hydrogen bonding. For example, ammonia (NH3) and methanol (CH3OH) are highly soluble in water as they form hydrogen bonds. H Hydrogen bond H Hydrogen bond H N H O H C O H O H H H H H Ammonia Water Methanol Water (3) Three dimensional crystal lattice As already stated, hydrogen bonds are directional and pretty strong to form three dimensional crystal lattice. For example, in an ice crystal the water molecules (H2O) are held together in a tetrahedral network and have the same crystal lattice as of diamond. This is so because the O atom in water has two covalent bonds and can form two hydrogen bonds. These are distributed in space like the four covalent bonds of carbon. The tetrahedral structural units are linked to other units through hydrogen bonds as shown in Fig. 5.6. Since there is enough empty space in its open lattice structure ice is lighter than water, while most other solids are heavier than the liquid form. Water as an Interesting Liquid Water is very interesting solvent with unusual properties. It dissolves many ionic compounds and polar organic compounds. It has high heat of vaporisation, high heat of fusion, high specific heat with melting point 273 K and boiling point 373 K. Its structure as shown above is very interesting as it explains many properties : 174 5 PHYSICAL CHEMISTRY (1) Ice (solid) is lighter than water (Liquid) The structure of water is tetrahedral in nature. Each oxygen atom is linked to two H-atoms by covalent bonds and other two H-atoms by hydrogen bonding. In this solid state (Ice), this tetrahedral structure is packed resulting in open cage like structure with a number of vacant space. Hence in this structure the volume increases for a given mass of liquid water resulting in lesser density. Due to this reason ice floats on water. (2) Maximum density of water at 277 K (4ºC) On melting ice, the hydrogen bonds break and water molecules occupy the vacant spaces. This results in decrease in volume and increase in density (d = m/v). Hence density of water keeps on increasing when water is heated. This continues upto 277 K (4ºC). Above this temperature water molecules start moving away from one another due to increase in kinetic energy. Due to this volume increases again and density starts decreasing. This behaviour of water is shown in the fig. 5.9. 1.0 Density 273 274 275 276 277 278 279 280 281 282 Temperature (K) Figure 5.9 A plot of density versus temperature (water). EXCEPTIONS TO THE OCTET RULE For a time it was believed that all compounds obeyed the Octet rule or the Rule of eight. However, it gradually became apparent that quite a few molecules had non-octet structures. Atoms in these molecules could have number of electrons in the valence shell short of the octet or in excess of the octet. Some important examples are : (1) Four or six electrons around the central atom A stable molecule as of beryllium chloride, BeCl2, contains an atom with four electrons in its outer shell. x Be x + 2 Cl Cl Be Cl (4 Electrons about Be) The compound boron trifluoride, BF3, has the Lewis structure : Cl x x B x + 3 Cl Cl B Cl (6 Electrons about B) CHEMICAL BONDING - LEWIS THEORY 175 The boron atom has only six electrons in its outer shell. Beryllium chloride and boron trifluoride are referred to as electron-deficient compounds. (2) Seven electrons around the central atom There are a number of relatively stable compounds in which the central atom has seven electrons in the valence shell. A simple example is chlorine dioxide, ClO2. O + Cl + O O Cl O Chlorine dioxide The chlorine atom in ClO2 has seven electrons in its outer shell. Methyl radical (CH3) has an odd electron and is very short lived. When two methyl free radicals collide, they form an ethane molecule (C2H6) to satisfy the octet of each carbon atom. Any species with an unpaired electron is called a free radical. H H H 2H C H C C H H H H (C has 7 electrons) Ethane (3) Ten or more electrons around the central atom Non-metallic elements of the third and higher periods can react with electronegative elements to form structures in which the central atom has 10, 12 or even more electrons. The typical examples are PCl5 and SF6. Cl F F F Cl Cl P S F F Cl Cl F (10 Electrons about P) (12 Electrons about S) The molecules with more than an octet of electrons are called superoctet structures. In elements C, N, O and F the octet rule is strictly obeyed because only four orbitals are available (one 2s and three 2p) for bonding. In the elements P and S, however, 3s, 3p, and 3d orbitals of their atoms may be involved in the covalent bonds they form. Whenever an atom in a molecule has more than eight electrons in its valence shell, it is said to have an expanded octet. VARIABLE VALENCE Some elements can display two or more valences in their compounds. The transition metals belong to this class of elements. The Electronic Structure of some of these metals is given below : 176 5 PHYSICAL CHEMISTRY TABLE 5.2. ELECTRONIC STRUCTURE OF THE TWO OUTERMOST SHELLS OF SOME TRANSITION METALS Sc 3d 1 4s 2 Ag 4d 10 5s 1 Cr 3d 5 4s 1 La 5d 1 6s 2 Mn 3d 5 4s 2 Os 5d 6 6s 2 Fe 3d 6 4s 2 Ir 5d 7 6s 2 Co 3d 7 4s 2 Pt 5 d 96 s 2 Cu 3d 10 4s 1 Au 5d 10 6s 1 Most of the transition metals have one or two outer-shell electrons and they form monovalent or bivalent positive ions. But because some of the d electrons are close in energy to the outermost electrons, these can also participate in chemical bond formation. Thus transition metals can form ions with variable valence. For example, copper can form Cu1+ and Cu2+ ions and iron can form Fe2+ and Fe3+ ions. The complete electronic configuration of an iron atom is Fe = 1s2 2s2 2p6 3s2 3p6 4s2 3d 6 It can form Fe2+ by losing two 4s electrons, Fe2+ = 1s2 2s2 2p6 3s2 3p6 3d 6 When iron loses two 4s electrons and one of the three 3d electrons, if forms Fe3+ ion Fe3+ = 1s2 2s2 2p6 3s2 3p6 3d 5 1+ 2+ Copper form Cu and Cu ions by losing one 4s electron, and one 4s and 3d electron respectively Cu = 1s2 2s2 2p6 3s2 3p6 3d10 4s1 Cu1+ = 1s2 2s2 2p6 3s2 3p6 3d10 Cu2+ = 1s2 2s2 2p6 3s2 3p6 3d 9 It may be noted that the structures of Fe2+, Fe3+, Cu1+, Cu2+, Cr3+, etc., are not isoelectronic with any of the noble gases, and hence the d electrons being unstable are available for bond formation. (The atoms and ions that have the same number of electrons are said to be Isoelectronic). METALLIC BONDING The valence bonds that hold the atoms in a metal crystal together are not ionic, nor are they simply covalent in nature. Ionic bonding is obviously impossible here since all the atoms would tend to give electrons but none are willing to accept them. Ordinary covalent bonding is also ruled out as, for example, sodium atom with only one outer-shell electron could not be expected to form covalent bonds with 8 nearest neighbouring atoms in its crystal. The peculiar type of bonding which holds the atoms together in metal crystal is called the Metallic Bonding. Many theories have been proposed to explain the metallic bonding. Here we will discuss the simplest of these : The Electron Sea Model. THE ELECTRON SEA MODEL Metal atoms are characterised by : (1) Low ionization energies which imply that the valence electrons in metal atoms can easily be separated. (2) A number of vacant electron orbitals in their outermost shell. For example, the magnesium atom with the electron configuration 1s2 2s2 2p6 3s2 3p0 has three vacant 3p orbitals in its outer electron shell. CHEMICAL BONDING - LEWIS THEORY 177 There is considerable overlapping of vacant orbitals on one atom with similar orbitals of adjacent atoms, throughout the metal crystal. Thus it is possible for an electron to be delocalized and move freely in the vacant molecular orbital encompassing the entire metal crystal. The delocalized electrons no longer belong to individual metal atoms but rather to the crystal as a whole. As a result of the delocalization of valence electrons, the positive metal ions that are produced, remain fixed in the crystal lattice while the delocalized electrons are free to move about in the vacant space in between. The metal is thus pictured as a network or lattice of positive ions of the metal immersed in a ‘sea of electrons’ or ‘gas of electrons’. This relatively simple model of metallic bonding is referred to as the Electron Sea model or the Electron Gas model (Fig. 5.10.) Figure 5.10 The Electron Sea model of metallic bonding. A metallic bond is the electrostatic force of attraction that the neighbour positive metallic ions have for the delocalized electrons. e- + + e- The electron sea model of metallic bonding explains fairly well the most characteristic physical properties of metals. (1) Luster or Reflectivity. The delocalized mobile electrons of the ‘electron sea’ account for this property. Light energy is absorbed by these electrons which jump into higher energy levels and return immediately to the ground level. In doing so, the electrons emit electromagnetic radiation (light) of the same frequency. Since the radiated energy is of same frequency as the incident light, we see it as a reflection of the original light. (2) Electric Conductivity. Another characteristic of metals is that they are good conductors of electricity. According to the electron sea model, the mobile electrons are free to move through the vacant space between metal ions. When electric voltage is applied at the two ends of a metal wire, it causes the electrons to be displaced in a given direction. The best conductors are the metals which attract their outer electrons the least (low ionization energy) and thus allow them the greatest freedom of movement. 178 5 PHYSICAL CHEMISTRY e- e- e- e- Exiting e- electricity Entering e- e- e- electricity e- e- e- Figure 5.11 Electrical conductivity by flow of electrons based on Electron Sea model. (3) Heat Conductivity. If a metal is heated at one end, the heat is carried to the other end. The mobile electrons in the area of the ‘electron sea’ around one end of the metal easily absorb heat energy and increase their vibrational motion. They collide with adjacent electrons and transfer the added energy to them. Thus the mobility of the electrons allows heat transfer to the other end (Fig. 5.12). e- e- e- e- e- Heat energy e- e- e- e- Figure 5.12 Heat conduction through a metal. (4) Ductility and Malleability. The ductility and malleability of metals can also be explained by the electron sea model. In metals the positive ions are surrounded by the sea of electrons that ‘flows’ around them. If one layer of metal ions is forced across another, say by hammering, the internal structure remains essentially unchanged (Fig. 5.13). The sea of electrons adjusts positions rapidly and the crystal lattice is restored. This allows metals to be ductile and malleable. However, in ionic crystals of salts e.g., sodium chloride, displacement of one layer of ions with respect to another brings like charged ions near to each other. The strong repulsive forces set up between them can cause the ionic crystals to cleave or shatter. Thus ionic crystals are brittle. e- e- e- e- e- e- e- e- e- e- e- e- e- e- e- e- Before After Figure 5.13 When force is applied to the upper layer of cations it slips to the right without changing the environments. illustration of malleability and ductility. CHEMICAL BONDING - LEWIS THEORY 179 (5) Electron Emission. When enough heat energy is applied to a metal to overcome the attraction between the positive metal ions and an outer electron, the electron is emitted from the metallic atom. When the frequency and, therefore, the energy of the light that strikes the metal is great enough to overcome the attractive forces, the electron escapes from the metal with a resultant decrease in the energy of the incident photon (Photoelectric effect). GEOMETRIES OF MOLECULES So far we have depicted molecules by Lewis structures in the flat plane of paper. But all molecules containing three or more atoms are three-dimensional. The shape of a particular molecule is determined by the specific arrangement of atoms in it and the bond angles. Molecular shapes may be linear, bent (or angular), trigonal planar, pyramidal or tetrahedral. The shapes of molecules can be determined in the laboratory by modern methods such as X-ray and electron diffraction techniques. Molecular shapes are important because they are helpful in the investigation of molecular polarity, molecular symmetry or asymmetry. Physical and chemical properties of compounds depend on these factors. VSEPR theory throws light on the three dimensional shapes of molecules. VSEPR THEORY The Lewis structure of a molecule tells us the number of pairs of electrons in the valence shell of the central atom. These electron pairs are subject to electrostatic attractions between them. On this basis, R.G.Gillespie (1970) proposed a theory called the Valence-Shell Electron Pair Repulsion or VSEPR (pronounced as ‘Vesper’) theory. It states that : The electron pairs (both lone pairs and shared pairs, surrounding the central atom will be arranged in space as far apart as possible to minimise the electrostatic repulsion between them. o o o 90 120 180 Central atom Two electron Two electron Two electron o o o pairs at 90 pairs at 120 pairs at 180 Figure 5.14 o o Arrangement of two electron pairs on circle at 90 , 120o and at 180. o Placement of electron pairs at 180 puts them the farthest apart, thereby minimising the electrostatic repulsion. Let us consider the simplest case of an atom with two electron pairs. We wish to place the electron pairs on the surface of a sphere such that they will be as far apart as possible so as to minimise repulsion between them. Fig. 5.14 illustrates it by showing some possible placements of the two electron pairs. The arrangement in which the electron pair-central atom-electron pair angles is 180º, makes the electron pairs farthest apart. This arrangement is called linear because the electron pairs and the central atom are in a straight line. VSEPR theory is simple but remarkably powerful model for predicting molecular geometries and bond angles. While working out the shapes of molecules from this theory, it must be remembered : (1) Multiple bonds behave as a single electron-pair bond for the purpose of VSEPR. They represent a single group of electrons. 180 5 PHYSICAL CHEMISTRY (2) Order of repulsions between lone pair and lone pair (lp-lp), lone pair and bonding pair (lp- bp), and bonding pair and bonding pair (bp-bp) is lp – lp > > lp – bp > bp – bp When a molecule has lone pairs of electrons, the bonding electron pairs are pushed closer and thus the bond angle is decreased. Now we proceed to work out the shapes of some common molecules with the help of VSEPR theory. (1) Linear Molecules (a) Beryllium chloride, BeCl2. It has the Lewis structure Repel one o another 180 Cl Be Cl Cl Be Cl Lewis structure VSEPR Model Figure 5.15 Geometry of BeCl2 molecule. The central atom Be has two bonding electron pairs and no unshared electron. According to VSEPR theory, the bonding pairs will occupy positions on opposite sides of Be forming an angle of 180º. An angle of 180º gives a straight line. Therefore, BeCl2 molecule is linear. In general, all molecules as A–B–A which have only two bonds and no unshared electrons are linear. (b) Carbon dioxide, CO2. It has the structure Counts the same o as single bond 180 O C O O C O O C O Lewis structure Modified VSEPR Model structure Figure 5.16 Geometry of CO2 molecule. The central C atom has no unshared electron. We know that a double bond counts the same as a single bond in VSEPR model. Thus CO2 is a linear molecule. Similarly, it can be shown that hydrogen cyanide (H – C ≡ N) and acetylene (H – C ≡ C – H) are linear molecules. (2) Trigonal Planar Molecules (a) Boron trifluoride, BF3. Its Lewis structure shown that the central atom B has three bonding electron pairs and no unshared electrons. VSEPR theory says that the three bonding electron pairs will be as far apart as possible. This can be so if these electron pairs are directed to the corners of an equilateral triangle. Thus VSEPR model of BF3 molecule has three F atoms at the corners of the triangle with B atom at its centre. All the four atoms (three F and one B) lie in the same plane. Therefore, the shape of such a molecule is called trigonal planar. The bond angle is 120º. CHEMICAL BONDING - LEWIS THEORY 181 F Equilateral F triangle F o F B or 120 F B B Lewis formula F F F F VSEPR Model Figure 5.17 Geometry of BF3 molecule. (b) Sulphur trioxide, SO3. In the Lewis structure of SO3, the central S atom is joined with two O atoms by covalent bonds. The third O atom is joined with S by a double bond. But a double bond is counted as a single electron pair for the purpose of VSEPR model. Therefore, in effect, S has three electron pairs around it. Thus like BF3, SO3 has trigonal planar geometry. Counted as Surrounded by single electron pair 3 electron pairs O O O 120 o S O S O S O O O O Lewis structure Modified VSEPR Model structure (trigonal, planar) Figure 5.18 Geometry of SO3 molecule. (3) Tetrahedral Molecules (a) Methane, CH4. Lewis structure of methane shows that the central C atom has four bonding electron pairs. These electron pairs repel each other and are thus directed to the four corners of a regular tetrahedron. A regular tetrahedron is a solid figure with four faces which are equilateral triangles. All bond angles are 109.5º. H Tetrahedron Has four H electron pairs o 109.5 C C H C H H H H H Lewis structure Electron pairs directed VSEPR Model to the corners of a (tetrahedral) tetrahedron Figure 5.19 Geometry of CH4 molecule. Similarly, CCl4 in which the central C atom is bonded to four other atoms by covalent bonds has tetrahedral shape. 182 5 PHYSICAL CHEMISTRY (b) Ammonium ion, NH4+ , and Sulphate ion, SO42–. The N atom in NH +4 and S atom in SO24 – have four electron pairs in the valence shell. These are directed to the corners of a tetrahedron for maximum separation from each other. Thus both NH +4 and SO 24 – have tetrahedral shape. H O o o 109.5 +1 109.5 +2 N S H H O O H O + VSEPR Model of NH 4 2 VSEPR Model of SO 4 Figure 5.20 + 2 Geometry of NH 4 ion and SO4 ion. (4) Pyramidal Molecules (a) Ammonia molecule. The Lewis structure of NH3 shows that the central N atom has three bonding electrons and one lone electron pair. The VSEPR theory says that these electron pairs are directed to the corners of a tetrahedron. Thus we predict that H–N–H bond angle should be 109.5º. But the shape of a molecule is determined by the arrangement of atoms and not the unshared electrons. Thus, if we see only at the atoms, we can visualise NH3 molecule as a pyramid with the N atom located at the apex and H atoms at the three corners of the triangular base. According to VSEPR theory, a lone pair exerts greater repulsion on the bonding electron pairs than the bonding pairs do on each other. As a result, the bonds of NH3 molecule are pushed slightly closer. This explains why the observed bond angle H–N–H is found to be 107.3º instead of 109.5º predicted from tetrahedral geometry. Lone pair Pyramid H N H N N H H H 107.3 H o H Lewis formula H H Pyramidal shape Pyramidal shape with of NH3 bond angle decreased Figure 5.21 to 107.3 o Geometry of NH3 molecule. All molecules in which the N atom is joined to three other atoms by covalent bonds, have pyramidal shape. For example, amines RNH2, R2NH and R3N have pyramidal shape. CHEMICAL BONDING - LEWIS THEORY 183 More repulsion N N o o 109.5 107.3 Less repulsion Tetrahedral geometry The bond angle decreases predicts H—N—H because of greater repulsion o angle of 109.5 of lone electron pair Figure 5.22 Why the angle H—N—H in NH3 molecule is 107.3o o while the tetrahedral angle is 109.5 ? (b) Phosphorus trichloride, PCl3. The structural formula indicates that the central phosphorus atom has three bonding electron pairs and one lone electron pair. Thus, like NH3 it has pyramidal shape and the observed bond angle Cl–P–Cl is 100º. P o Cl P Cl Cl 0 Cl 10 Cl Cl Lewis formula Pyramidal PCl3 molecule Figure 5.23 Geometry of PCI3 molecule. (5) Bent or Angular Molecules (a) Water, H2O. In the structural formula of H2O, the O atom is bonded to two H atoms by covalent bonds and has two lone pairs. Thus O is surrounded by two bonding electron pairs and two Has 4 electron pairs H O H O O o H 105 H H Predicted bond angle Bent molecule of H2 O with o 109.5 o H observed bond angle 105 Figure 5.24 Geometry of H2O molecule. unshared electron pairs. VSEPR theory says that in order to secure maximum separation between them, the four electron pairs are directed to the corners of a tetrahedron. If we look at the three atoms (and ignore the unshared pairs), the atoms HOH lie in the same plane and the predicted bond angle is 109.5º. But with two unshared pairs repelling the bonding pairs, the bond angle is compressed to 105º, the experimental value. Thus the H2O molecule is flat and bent at an angle at the O atom. Such a molecule is called a bent molecule or angular molecule. 184 5 PHYSICAL CHEMISTRY (b) Sulphur dioxide, SO2. The Lewis structure of SO2 is given below. The S atom is bonded to one O by a double bond and to the other O by a single bond. It has an unshared electron pair. In VSEPR model a double bond is counted as a single electron pair. That way, the S atom is surrounded by three electron pairs, two bonding pairs and one unshared pair. For maximum separation the three electron pairs are directed to the corners of an equilateral triangle. The predicted bond angle is 120º. But with the unshared electron pair repelling the bonding electron pairs, the bond angle is actually reduced somewhat. Thus SO2 has a planar bent molecule with the observed bond angle 119.5º. O S O S S Lewis structure o 120 O O O O VSEPR Model Bent molecule of SO2 with bond angle 119.5 o Figure 5.25 Geometry of SO2 molecule. SUMMARY : SHAPES OF MOLECULES Methane (CH4) Ammonia (NH3) Water (H2O) Hydrogen fluoride (HF) The directional nature of covalent bonds is shown in the diagrams of molecules above. The shape of the methane molecule is tetrahedral because the four bonding pairs of electrons repel each other equally, and the equilibrium position of all four bonding electron pairs is tetrahedral. HOW TO WORK OUT THE SHAPE OF A MOLECULE It is possible to work out the shape of a small molecule that has a formula XYn by applying a few simple rules. We will use ammonia as an example to illustrate the idea. Rule 1 First find the number of bonding pairs of electrons in the molecule. The number of bonding pairs of electrons in the molecule NH3 can be seen in the formula. There must be three bonding pairs of electrons holding the three hydrogens onto the nitrogen. Rule 2 Find the number of valence electrons (electrons in the outer energy level) on an atom of the central atom (The one of which there is only one.) Nitrogen is in group V, so the nitrogen has five electrons in the outer energy level. Rule 3 Find the number of lone pairs on the central atom by subtracting the number of bonding pairs (3) from the valence electrons (5) to find the number of electrons (2) that will make up lone pairs of electrons. Divide this number by 2 to find the number of lone pairs, 2/2 = 1. Rule 4 Distribute all the electron pairs around the central atom and learn the angles they will make from molecules with no lone pairs. Rule 5 Learn that the repulsion between lone pairs of electrons is greater than the repulsion between bonding pairs, and subtract 2o from the bond angles for every lone pair. Rule 6 Learn the names of the shapes. The shapes are named from the position of the atoms and not the position of the orbitals. CHEMICAL BONDING - LEWIS THEORY 185 TABLE OF SHAPES Formula BeCl2 BCl3 CH4 NH3 H2O Beryllium Boron Methane Ammonia Water chloride trichloride Bonding Pairs 2 3 4 3 2 Valence Electrons 2 3 4 5 6 Lone Pairs 0 0 0 1 2 Angles between bonding pairs 180° 120° 109.5° 107° 105° Name of shape Linear Trigonal Tetrahedral Trigonal