Inhalation Agents PDF
Document Details
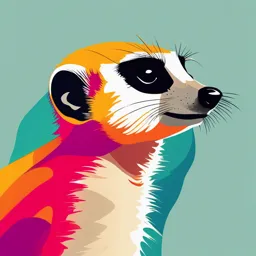
Uploaded by UnwaveringEpitaph
Tags
Summary
These notes provide an overview of inhalation agents, including required readings, objectives, and introductory information related to anesthesia. The document is focused on the chemical and pharmacological aspects of the agents used in anesthesia.
Full Transcript
Inhalation Agents Required Readings: Katzung Chpt 25 Nagelhout Chapters 7& 8 Morgan & Mikhail Chapter 8 Recommended Supplemental Reading Hemmings Chapter 3 Objectives 1. Discuss signs & stages of general anesthesia 2. Discuss MAC and relationship to...
Inhalation Agents Required Readings: Katzung Chpt 25 Nagelhout Chapters 7& 8 Morgan & Mikhail Chapter 8 Recommended Supplemental Reading Hemmings Chapter 3 Objectives 1. Discuss signs & stages of general anesthesia 2. Discuss MAC and relationship to potency. Identify MAC value for each of the inhalation agents, factors that increase, decrease and have no effect on MAC. 3.Discuss the proposed mechanisms & sites of action of anesthetic gases, and effect of isomerism. 4. Understand the blood : gas partition coefficient and how this factor affects the rate at which partial pressure of the anesthetic in which the alveoli increase Objectives 5. List contraindications to the use of nitrous oxide. Understand why diffusion hypoxia occurs and what can be done to avoid its occurrence. 6.Identify key effects associated with nitrous oxide, halothane, sevoflurane, enflurane, desflurane, isoflurane including Coronary steal, LFT elevations associated with halothane malignant hyperthermia, analgesic action 7. Discuss the physiologic, pathophysiologic, and clinical variables that affect uptake and distribution gases. Introduction Goals of Therapy “ The state of ‘general anesthesia’ generally includes: analgesia, amnesia, loss of consciousness, inhibition of autonomic reflexes, skeletal muscle relaxation”. Introduction Qualities of an ideal inhalation agent – non-flammable – rapid, smooth onset & recovery – high therapeutic index – no adverse effects Introduction Introduction General Anesthesia: Reversible state of “loss of consciousness” Stages and Signs of anesthesia stage 1- analgesia stage 2- delirium stage 3-surgical anesthesia stage 4-respiratory paralysis Stages and Signs of anesthesia – stage 1- analgesia: higher cortical center is mildly depressed; this stage is suitable for surgical procedures not requiring extensive muscular relaxation – stage 2- delirium: excitement resulting from depression of the cortical motor center; may result in extensive involuntary activity – stage 3-surgical anesthesia: composed of 4 “planes” which correspond to progressive increases in depth of anesthesia: (1) loss of spinal reflexes, (2) decreased muscle reflexes, (3) paralysis of intercostal muscles, (4) disappearance of muscle tone – stage 4-respiratory paralysis: usually refers to as toxic or overdose stage Definitions & Background Gases & Vapors Inhalation anesthetic agents are either gases or vapors. In the gaseous phase, a compound is considered a vapor when it is below its critical temperature and a gas when it is above its critical temperature. The critical temperature is the above which it is no longer possible to liquify a vapor by applying pressure; the substance is in the gas phase above critical temperature. Every substance has a critical temperature above which the substance can only exist as a gas, no matter how much pressure is applied. Gases & Vapors Volatile anesthetics have low vapor pressures and relatively high boiling points so they are liquids at room temperature (20o C) Nitrous oxide (N2O) and Xeon have high vapor pressure and low boiling points so they are gases at room temperature For simplicity, they are all referred to as “gases”. Note: all are unionized and have low molecular weights Partial Pressure Partial Pressure : the component of total pressure that each gas exerts (Dalton’s Law of Partial Pressures: the partial pressure of a gas in a mixture of gases is the pressure that gas would exert if it occupied the total volume of the mixture) PT = P1 + P2+ P3 + P4 + P5 + … Units of Pressure Std atmospheric pressure (atm): 14.7 lbs per square inch; 760 mm Hg sea level Torr: atmospheric pressure required to support 1 mm of Hg Pascal (Pa): metric unit of pressure ( 1 newton/m2); kPa Example of Partial Pressure Mixture of gases at 760 mm Hg 70% Nitrous Oxide Partial Pressure of gas mix = Ppgas partial pressure = 532 mm Hg 0.7 x 760 = 532 PPgas= % composition x P total Concentration of gas ∞ partial pressure 29% O2 Partial pressure = 220 mm Hg 1% isoflurane Partial pressure = 7.6 mm Hg Pharmacokinetics Inhalation anesthetic agents must pass through many barriers between the anesthesia machine and the brain. Citation: Chapter 8 Inhalation Anesthetics, Butterworth IV JF, Mackey DC, Wasnick JD. Morgan & Mikhail’s Clinical Anesthesiology, 7e; 2022. Available at: https://accessanesthesiology.mhmedical.com/content.aspx?bookid=3194§ionid=266517982 Accessed: September 18, 2023 Copyright © 2023 McGraw-Hill Education. All rights reserved Pharmacokinetics Depth of anesthesia partial pressure of anesthetic in the brain CNS Pinhaled P alveoli Pblood PCNS Gases equilibrate based on partial pressures Effect of Partial Pressure The higher the partial pressure of a gas in inhaled air (lungs), the more rapidly the anesthetic gas achieves therapeutic concentration in the blood (loading dose) and, ultimately, CNS Pharmacokinetics Equilibration of partial pressures in alveoli and CNS is the result of 3 factors – Bidirectional transfer via lungs to/from blood to/from CNS as partial pressures equilibrate – Plasma & tissues have low capacity to absorb inhaled anesthetic such that concentrations are quickly attained or removed – Minimal metabolism , excretion, and redistribution relative to rate of delivery means CNS concentration is easy to maintain Partition Coefficient Partition coefficient refers to how a gas will partition itself between 2 phases after equilibrium has been reached ( partial pressure is equal in both phases) The ratio of the amount of a gas in equal volumes of two phases at a certain temperature when the 2 phases are in equilibrium. Dependent on the solubility of the gas in each phase Partition Coefficient Example: consider a gas with a “blood/gas coefficient” of 1.4 At equilibrium the concentration of the gas in the blood will be 1.4 times greater than the concentration in the alveoli The more soluble an anesthetic, the more slowly the partial pressure rises The speed of induction of anesthesia is inversely proportional to the solubility of the agent (blood : gas partition coefficient) The speed of induction of anesthesia is directly proportional to the speed at which the agent equilibrates with the blood. Agents with a low partition coefficient equilibrate faster than agents with a greater blood solubility Recall : Henry’s Law “At constant temperature, the amount of gas dissolved in a liquid is directly proportional to the partial pressure of that gas at equilibrium with the liquid.” S= kP where S is concentration (amount of gas dissolved in a liquid), P is the partial pressure, and k is the solubility (Henry’s Law Constant) constant that depends on the gas, solvent, and temperature (as temperature increases, Recall: Solubility Solubility - how much of a particular solute can dissolve in a certain solvent at a specified temperature Factors which affect solubility: 1. Polarity of solute and solvent The more different they are, the lower the solubility 2. Temperature Increase in temperature usually increases solubility (solids in liquids; Gases are most soluble at low temperatures) 3. Pressure Solubility of a gas in liquid is directly proportional to applied pressure. Gases are most soluble at high pressure blood/gas Blood/gas partition coefficient = b/g solubility anesthetics with higher blood/gas solubility coefficient ( blood/gas) are more soluble in blood and stay in blood more extensively than agents with a low blood/gas solubility coefficient slower onset of anesthesia slower elimination of anesthetic Know blood/gas partition coefficients for inhalation agents FA /Fi Fractional concentration of alveolar/ fractional conc of inhaled The driving force for uptake of an inhaled anesthetic is the ratio between alveolar and inspired concentration. The greater the inhaled partial pressure, the more rapidly the alveolar pressure approaches the inspired partial pressure = concentration effect Low blood/gas anesthetics achieve a lung concentration faster than higher b/g anesthetics Question Options Sevoflurane Isoflurane Halothane Desflurane Increased ventilation is more effective in decreasing induction time by raising alveolar concentration of anesthetics having higher blood/gas solubilities Anesthetic uptake by blood from the lungs = x Q x P (A-v) = partition coefficient (solubility) of anesthetic in the blood higher solubility → slower the partial pressures equilibrate Anesthetic uptake by blood from the lungs = x Q x P (A-v) Q= Cardiac Output/Perfusion increased pulmonary blood flow results in larger blood volume being exposed to the anesthetic causing an increase in blood “capacity” and slower rate of rise in arterial tension; especially in agents with higher blood/gas solubility anesthetic uptake by blood from the lungs = x Q x P (A-v) P (A-v) = alveolar to venous anesthetic difference in partial pressure (difference of anesthetic between arterial and venous blood) – The difference of anesthetic concentration (partial pressure difference) between arterial and venous blood is due to tissue uptake. Uptake of anesthetic by tissues from the blood = T x Q x P (a-t) factors determining tissue uptake of anesthesia – tissue solubility (tissue/blood partition coefficient) – blood flow to that tissue uptake is initially greatest in tissue with greatest perfusion, vessel-rich group > muscle > fat - arterial to tissue anesthetic partial pressure difference Summary of Factors Affecting FA/FI Rise Summary fast, deep breathing generally results in faster onset and elimination of anesthesia ventilation deficits or/and perfusion problems impairs administration of inhalants ventilation is more effective in decreasing induction time by raising alveolar concentration of anesthetics having higher blood/gas solubilities because they are more subject to uptake “Second Gas Effect” a concentration effect Uptake of a high concentration of a faster gas in higher concentration (ex N2O) can speed the onset of a slower gas (ex: isoflurane). N2O is most responsible for concentrating effect The uptake of the faster gas in the alveoli increases the alveolar concentration of a second slower gas (ex: halothane) when given together From: A Second Look at the Second Gas Effect Anesthesiology. 2018;128(6):1053-1054. doi:10.1097/ALN.0000000000002206 The second gas effect is a consequence of the concentration effect where a “first gas” that is soluble in plasma, such as nitrous oxide, moves rapidly from the lungs to plasma. This increases the alveolar Figure Legend: concentration and hence Image: J. P. Rathmell. rate of uptake into plasma of the “second gas.” Date of download: 9/15/2022 Copyright © 2022 American Society of Anesthesiologists. All rights reserved. Mechanisms of Action of Inhaled Anesthetics Mechanism and site of action have not been completely explained Most evidence indicate that the primary site of action is by binding directly to ion channels on cell membrane Potentiating effects on inhibitory GABAA and glycine receptors and K+ channels Inhibitory effects on excitatory ion channel (NMDA) Na+ and Ca2+. The gaseous anesthetics nitrous oxide and xenon have predominant NMDA-type glutamate receptor blocking effects Sites of Volatile Anesthetic Action Hemmings MAC The alveolar concentration of an inhaled anesthetic in volumes percent at steady state as measured by anesthetic gas monitors from sampled expired alveolar gases at one atmosphere of pressure that prevents movement in 50% of patients responding to a standard stimulus (surgical incision) – EC50 corresponds to the potency of the agent Correlates to high lipid solubility Drugs that cross BBB easily will be more potent Actions of Volatile Anesthetics Hemmings Lipid theory (Meyer & Overton) a direct relationship exists between the lipid solubility of an agent and its potency (potency of agent agent’s lipid solubility) plot log of MAC value of anesthetics vs log (oil: gas partition coefficient) the greater the solubility of an anesthetic in oil, the greater that anesthetic’s potency The greater the solubility of an inhaled anesthetic in olive Oil , the more potent it is The oil/gas partition coefficient of an agent is inversely correlated to MAC MAC of Inhaled Anesthetics MAC % : percentage of 1 atmosphere MAC 1.3 MAC prevents movement in at least 95% of patients (EC95) 0.33 MAC Awake is associated with awakening from anesthesia 1.5 MACBAR Prevents adrenergic responses in 50% of patients MAC values are additive 0.5 MAC of N2O + 0.5 MAC isoflurane = 1 MAC MAC values may be affected by physiological and pharmacological factors MAC as a Partial Pressure Factors Affecting MAC Factors that Increase MAC hyperthermia hypernatremia drugs that CNS catecholamine levels (ex: acute cocaine) Chronic alcohol use Factors Affecting MAC Factors that Reduce MAC hypothermia pregnancy lithium 2 agonists (clonidine) hypotension increasing age acute ethanol ingestion IV anesthetics/analgesics Factors that do not change MAC duration of anesthesia gender extent of anesthetic metabolism hypertension Question Options Sevoflurane Isoflurane Halothane Desflurane Nitrous Oxide Note Structures Properties of Inhaled Anesthetics Barash Overview FYI Hemmings Overview FYI Hemmings Comparison See M-M Page 155 Nitrous Oxide (N2O) Nitrous Oxide (N2O) chemistry & physical properties Nitrous oxide is an inorganic inhalation agent. It is a gas at room temperature and pressure since its critical temperature is above room temperature. not spontaneously flammable but will burn; colorless; odorless low solubility in blood , = 0.47 MAC 105 Nitrous Oxide (N2O) general pharmacological characteristics currently used as an adjunct anesthetic wide range of analgesic equivalent: 20%; unconscious 30-80% MOA: NMDA receptor antagonist elimination: exhalation Nitrous Oxide (N2O) general pharmacological characteristics Prolonged exposure ( 24 hrs): megaloblastic anemia (pernicious anemia), peripheral neuropathies Nitrous Oxide (N2O) pernicious anemia Nitrous Oxide (N2O) possible teratogen may alter immune response second gas effect diffusional hypoxia analgesic effects Nitronox Provides maternity patients in labor the ability to control their pain relief Nitronox is a demand flow system that provides the patient with a preset mixture of 50% nitrous oxide and 50% oxygen. The patient self- administers the gas by holding the mask to her face and breathing normally. The patient remains alert and able to follow instructions. Nitrous Oxide (N2O) Much more diffusible than nitrogen so N2O enters air filled cavities much faster than nitrogen or oxygen can leave the cavity to enter blood (Hypoxia) Result: Increased volume or pressure of air filled cavity – Bowel – Pneumothorax – Middle tympanic membrane – Air emboli – Eye – Nasal sinuses Comparison See M-M Page 155 Nitrous Oxide (N2O) cardiovascular symptoms N2O depresses myocardial contractility directly, but stimulates the release of catecholamines; net result is little effect on HR, BP, CO Nitrous Oxide (N2O) respiratory symptoms RR, TV hypoxic drive Refer to M-M table 8-6 compare to other agents Nitrous Oxide (N2O) Cerebral cerebral blood flow ICP Neuromuscular not much effect on skeletal muscle relaxation thought not to trigger malignant hyperthermia Nitrous Oxide (N2O) renal in renal blood flow RVR UO hepatic slight blood flow GI may cause post-op N/V via activation of the CTZ & vomiting center in the medulla Halogenated Anesthetics Note structure : alkane vs ether Note halogens Halogenated Anesthetics halogenation with fluoride stabilizes the compound and reduces metabolism halogenation increases potency and solubility nonflammable, non-explosive all are potent & effective ethers are associated with less propensity to cause arrhythmias Halogenated Anesthetics Elimination is primarily by exhalation Halothane Metabolism Hemmings See M-M Page 155 Halothane (Fluothane ) Halothane (Fluothane ) chemistry & physical properties halogenated alkane (note structure) non-flammable, colorless liquid with a sweet odor not stable in light; thymol (0.01%) is added as a preservative metabolism & elimination: 20%-40% hepatically metabolized via cytochrome p-450 enzymes; exhalation Halothane (Fluothane ) general pharmacological characteristics not irritating to airway permits a rapid increase in inspired gas concentration (smooth, rapid onset) = 2.3; MAC = 0.75% oxidized via P450-2e1 in the liver to metabolite, trifluoroacetic acid (TFA) Halothane (Fluothane ) halothane hepatitis: rare; middle aged, obese women are risk; immune- system mediated (Ab response to TFA) drug interactions: theophylline, labetalol, epinephrine, NE, terbutaline, isoproterenol, midazolam, verapamil Halothane (Fluothane ) cardiovascular effects ↓ HR; due to blocked reflex tachycardia; halothane slows SA node conduction, prolongs the QT interval ↓ CO ; myocardial depression related to intracellular Ca++ by blocking calcium channels, impairs the uptake and release of Ca from the endoplasmic reticulum (acts negative inotrope) Halothane (Fluothane ) BP sensitizes heart to effects of epinephrine due to endogenous release of catecholamine Halothane (Fluothane ) respiration effects causes rapid, shallow breathing, TV, PaCO2 , hypoxic drive bronchodilator, mucociliary function cerebral CVR CBF ICP blunts autoregulation of CBF Halothane (Fluothane ) muscular system relaxes skeletal muscle; effect of NMB Most potent trigger of malignant hyperthermia renal RBF UO hepatic hepatic blood flow; note metabolism Enflurane (Ethrane ) Enflurane (Ethrane ) chemistry & physical properties methyl ethyl ether; clear, colorless, nonflammable liquid; pungent odor Intermediate onset & recovery Oxidized in the liver ( p450-2E1) to produce fluoride ions that could be nephrotoxic Enflurane (Ethrane ) cardiovascular BP corresponding to depth of anesthesia contractility, O2 demand by the heart does not affect HR, does not potentiate arrhythmias Cerebral associated with occurrence of szs enflurane does not affect catecholamine concentration Isoflurane (Forane ) Isoflurane (Forane ) chemistry & physical properties – isomer of enflurane; pungent odor – not flammable, physically stable compound – minimal metabolism Isoflurane (Forane ) general pharmacological characteristics induction is moderately fast = 1.4, MAC = 1.2 % Isoflurane (Forane ) cardiovascular increasing depth anesthesia BP, no change CO generally coronary blood flow is maintained; coronary steal theoretically may occur HR but does not precipitate arrhythmias minimal sensitization of the heart to the effect of catecholamines (epi < 4.5mcg/kg) Rapid increases in isoflurane concentration can cause increased sympathetic activity with associated tachycardia and HTN Isoflurane (Forane ) renal GFR resolves quickly post-op; less fluoride released from metabolism repeat exposures to isoflurane are not associated with renal injury not contraindicated in renal disease hepatic & GI post-op N& V little effect on GI and liver blood flow; has not been associated with hepatoxicity Desflurane (Suprane ) Desflurane (Suprane ) chemistry & physical properties halogenated ether; vapor pressure = 664 mm Hg (boils at room temperature in Denver) need to use a special heated vaporizer nonflammable, stable, noncorrosive to metals FYI: Vaporizers Miller Desflurane (Suprane ) general pharmacological characteristics = 0.42, MAC= 6%; very pungent, and irritating to the airway; coughing, sialorrhea, laryngospasm, breath holding, can induce bronchoconstriction in asthmatics the alveolar (or blood) conc. 80% of that from the vaporizer in about 5 minutes; pt may respond to commands ≈ 10-15 minutes after desflurane is dc’ed Desflurane (Suprane ) cardiovascular increasing depth anesthesia BP, little effect on CO HR moderate, does not precipitate arrhythmias coronary blood flow is maintained; coronary steal has not been reported Rapid increases in desflurane concentration can cause increased sympathetic activity with associated tachycardia and HTN Desflurane (Suprane ) respiratory significant respiratory depression; pt may cease spontaneous breathing at a MAC of 2% Carbon monoxide (CO) formation can occur from the degradation of desflurane by dry CO 2 absorbents cerebral cerebral vascular resistance ICP, cerebral metabolic rate, maintains autoregulation of CBF to CO2 ; convulsive EEG activity is not observed Desflurane (Suprane ) muscle enhances effect of neuromuscular blocking agents renal lack of renal toxicity; minimal metabolite formation urinary fluoride is not increased not contraindicated in renal disease hepatic desflurane is not contraindicated in hepatic dz Sevoflurane (Ultane ) Sevoflurane (Ultane ) chemistry & physical properties non-flammable, halogenated ether general pharmacological characteristics = 0.68, MAC = 2%; rapid on, rapid off; non-pungent; non-irritating to airway Sevoflurane (Ultane ) cardiovascular mild in contractility; little CO, no change in HR; no coronary steal respiratory as depth of anesthesia respiration; reverses bronchospasm similar to isoflurane Sevoflurane (Ultane ) muscular good neuromuscular skeletal relaxation for intubation Sevoflurane (Ultane) renal slight RBF; fluoride metabolites (via p450-2E1) have been associated with impaired renal tubule function ( conc. urine) – Actual use in patients with renal insufficiency has not been a significant problem sevoflurane can be decomposed by soda lime to form compound A ( olefin) which can cause nephrotoxicity in rats; has not shown an issue with humans. Sevoflurane (Ultane) WARNINGS Sevoflurane (Ultane) Animal and human studies demonstrate that sevoflurane administered for more than 2 MAC·hours and at fresh gas flow rates of < 2 L/min may be associated with proteinuria and glycosuria. During sevoflurane anesthesia the clinician should adjust inspired concentration and fresh gas flow rate to minimize exposure to Compound A. To minimize exposure to Compound A, fresh gas flow rates < 1 L/min are not recommended Xenon MAC% = 65%, = 0.15 Chemically stable Rapid induction and emergence Does not affect the ozone layer Lack of cardiac depression Does not trigger malignant hyperthermia Cost 1 MAC for 4 hrs = $167.00 Summary Naglehout Summary Recall signs & stages of general anesthesia Understand MAC and relationship to potency. Identify MAC value for each of the inhalation agents, factors that increase, decrease and have no effect on MAC. Recall the proposed mechanisms & sites of action of anesthetic gases, and effect of isomerism. Understand the blood gas: gas partition coefficient and how this factor affects the rate at which partial pressure of the anesthetic in which the alveoli increase Summary List contraindications to the use of nitrous oxide. Recall why diffusion hypoxia occurs and what can be done to avoid its occurrence. Identify key effects associated with nitrous oxide, halothane, sevoflurane, enflurane, desflurane, isoflurane including: coronary steal, LFT elevations associated with halothane malignant hyperthermia, analgesic action Understand the physiologic, pathophysiologic, and clinical variables that affect uptake and distribution gases