Inflammation PDF
Document Details
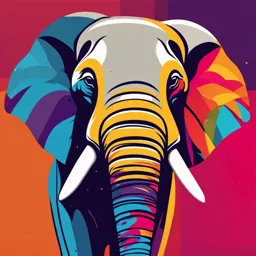
Uploaded by HearteningRelativity
University of Padua
Tags
Summary
This document provides a detailed overview of inflammation. It explains the two main types of inflammation, acute and chronic, and describes the involved processes and mediators. It also highlights the various stimuli that initiate an inflammatory response, such as infectious agents, physical trauma, and chemical agents.
Full Transcript
INFLAMMATION Inflammation is a complex series of responses initiated as a consequence of injury or infection, acting as a cascade activated by various stimuli. The primary goals of inflammation are to eradicate pathogens and facilitate tissue repair, processes in which the immune system excels. There...
INFLAMMATION Inflammation is a complex series of responses initiated as a consequence of injury or infection, acting as a cascade activated by various stimuli. The primary goals of inflammation are to eradicate pathogens and facilitate tissue repair, processes in which the immune system excels. There are many reasons behind: Infectious agents: such as bacteria, viruses, fungi, and parasites. Physical trauma: including burns, frostbite, radiation exposure, and ischemia (restricted blood supply). Chemical agents: involving harmful chemicals and toxins. The inflammatory response plays a critical role in cleaning the aDected area by removing dead cells and promoting tissue reconstruction through immune cell activity. Introduction: Acute and chronic inflammation Inflammation can be categorized into two main types: acute and chronic, diDerentiated not by their duration but by the types of cells involved. Acute Inflammation: Characterized by a massive influx of neutrophils, which are granulocytes identifiable by their purple, multilobed nuclei. These cells are the first responders to injury or infection. Chronic Inflammation: Marked by the dominance of macrophages and plasma cells, which are long, elongated cells with an eccentric nucleus. In chronic inflammation, neutrophils are gradually replaced by lymphocytes, which are small, round, and dark. The presence of lymphocyte clusters forming follicles indicates chronic inflammation 2 ACUTE INFLAMMATION In cases of damaged tissue, neutrophils are typically the first immune cells to arrive. However, during viral infections, lymphocytes may be the initial responders within the first 24 hours. Recognition-PRRs Immune cells need to recognize a pathogen or some sort of stimuli. There are two kind of this stimuli that are DAMPs and PAMPs. There are hundreds of diDerent molecules that can stimulation our immune cells, and as a response our immune cells become activated and start produce diDerent inflammatory mediates. This recognition is mediated by 4 classes of PRRs that recognize DAMPs ad PAMPs. Tool-like Receptors TLRs – they are express in all the immune cells, they are divided in 2 groups located in the cell membrane or intracellular. They are typically bacterial or virus. Activation of TLRs triggers the release of pro-inflammatory cytokines and other mediators, which leads to increased neutrophil infiltration, blood vessel dilation, and edema. These cytokines (like IL-1, TNF-α) recruit more immune cells to the area, which can be seen as a dense concentration of immune cells around the injured or infected area. NOD-like Receptors NLRs – 20 members of this groups, the NLR they are activated by a range of bacterial or viral molecules. NLRs are found inside cells and detect intracellular pathogens or cell damage signals Most of his PRR activate an NK B, and this is the outcome. o NLRPs - 12 members they share some sequence similarity of NLRs, since they are a subgroup of NLRs. they are also ligase that activated these receptors and thy are also activated by ROS. They are essential to generate the inflammasome which is an intracellular complex of immune cells that end up in the activation of caspase-1 to produce the inflammatory cytokines. Activation of inflammasomes is often seen in response to infections and cellular damage, leading to the recruitment of neutrophils and macrophages, evident as cellular infiltrates in tissue. C-type Lectine Receptors CLRs – not really interest for acute inflammation, they are not express at all in neutrophils, lectine are protein that binds carbohydrates, and they are express by microphage. CLRs detect fungal pathogens and some bacterial components. They are important in mounting an immune response against fungi by activating immune cells to produce cytokines. In infections where fungi are present, CLRs help mediate the inflammatory response, leading to the accumulation of immune cells like neutrophils and macrophages at the infection site. In fungal infections, you might also see characteristic granulomatous inflammation (formation of granulomas). 3 The immune cells that recognize these pathogens by opsonization. Fluorescence bacteria become coded by immunoglobulins or complement protein that just bind in a completely unspecific to the bacteria surface membrane. When the immunoglobulins and complement bind to a debris or a bacteria surface it can be recognize by immune cells because they have receptors, and we will have the immune response. Vascular Response When immune cells are stimulated by pathogens or tissue damage, they produce a range of mediators that induce local responses in the vascular system. The vascular responses to inflammation can be primarily categorized into vessel dilation and increased permeability. Vessel dilation occurs in small arteries as a direct consequence of inflammatory mediators released from activated immune cells. Key molecules involved in this process include nitric oxide synthase (NOS) and prostaglandins derived from arachidonic acid. These inflammatory mediators signal smooth muscle cells to relax, leading to an increase in vessel diameter. This dilation causes blood flow to become slower, a phenomenon known as stasis, which results in increased pressure within the vessels. As a consequence of the decreased blood flow and elevated pressure, edema begins to form, characterized by fluid pushing out from the vessels into the surrounding tissue. In the early phase of inflammation, this fluid is often referred to as transudate because it is primarily composed of water with low protein content. In addition to vessel dilation, increased permeability is a critical aspect of the inflammatory response. Endothelial cells, particularly on the postcapillary side of the vessels, are influenced by various mediators such as leukotriene B4 and bradykinin. These substances induce the contraction of endothelial cells, leading to a breakdown of the tight junctions and adherens junctions that normally maintain the integrity of the vascular barrier. Adherens junctions, which contain cadherin proteins, undergo ubiquitination and degradation when stimulated by inflammatory mediators, while tight junctions, made up of proteins like occludins, may be recycled back to the membrane once the stimulus subsides. 4 This disruption in junctional integrity results in increased vascular permeability, allowing immune cells and various molecules to exit the bloodstream and migrate into the inflamed tissue. The breakdown of these junctions facilitates a significant influx of fluids and proteins, leading to exudation. This exudation is characterized by the presence of a substantial amount of edema and a high concentration of proteins, causing the aDected area to appear red and swollen hallmarks of acute inflammation. Overall, the changes in vessel diameter and permeability during inflammation are essential for eDectively recruiting immune cells to the site of injury or infection, thereby enhancing the body's ability to respond to and resolve the inflammatory process. The clinical manifestations of redness, heat, and swelling are direct outcomes of these vascular responses, reflecting the underlying biological eDorts to contain and eliminate pathogens while promoting tissue repair. Inflammation Pain A variety of characteristics associated with inflammation are closely linked to the vascular response. The vascular response plays a crucial role in increasing blood flow to the aWected area, which is responsible for the sensations of heat and redness. Swelling occurs primarily due to exudation, the process through which fluid and proteins leak from the bloodstream into the surrounding tissues. Inflammatory pain arises from two key mechanisms: mechanonociception and chemonociception. Edema contributes to inflammatory pain by inducing mechanonociception, where excess fluid creates pressure on surrounding tissues. This pressure activates mechanoreceptors located in free nerve endings, sending pain signals to the brain. Central neurons respond specifically to this pressure or deformation, leading to the perception of pain. The process of nociception, which encompasses the overall signaling of pain, is mediated by specialized ion channels that respond to various stimuli. In the context of mechanonociception, these ion channels are activated when tissue is deformed or compressed, opening to allow ions to flow into the neuron and generating an action potential. A key player in this process is the Transient Receptor Potential (TRP) channels, which are multifunctional and can detect diDerent types of stimuli, including heat, mechanical stress, and chemical signals. TRP channels are considered polymodal sensors, enabling them to respond to various nociceptive signals. 5 Chemonociception is another mechanism of inflammatory pain, triggered by specific mediators such as prostaglandins and histamine. These substances bind to diDerent nociceptive receptors, leading to pain sensations in the aDected area. This process emphasizes the complexity of pain mechanisms during inflammation, where both mechanical and chemical factors contribute to the overall experience of pain. In summary, the interplay between the vascular response and nociceptive mechanisms underscores the multifaceted nature of inflammatory pain. Increased blood flow causes warmth and redness, while exudation leads to swelling. Both mechanonociception and chemonociception interact to create the painful sensations associated with inflammation, facilitated by specialized ion channels and the action of various mediators. Cellular Response The cellular response is a crucial aspect of inflammation, encompassing several key processes: margination, rolling, adhesion, diapedesis, chemotaxis, and phagocytosis. Phagocytosis is particularly significant, as it involves neutrophil granulocytes that play a vital role in eradicating pathogens through ingestion. Margination refers to the movement of immune cells within the blood. Under normal conditions, these cells circulate in the laminar flow of the bloodstream. However, in areas where blood flow is slowed—often due to stasis caused by increased viscosity as fluid leaks into extracellular spaces—transportation of immune cells becomes more turbulent. This turbulence facilitates margination, allowing immune cells to come into closer contact with the endothelium, where they can bind to specific partners. The binding partners can be categorized into two main groups: selectins and integrins. When immune cells encounter pathogens, they become activated through recognition receptors, leading to the production of various inflammatory mediators. Key mediators such as histamine and thrombin aDect the endothelial cells, prompting them to release organelles known as Weibel-Palade bodies. Within these bodies are pre-formed P-selectin, allowing endothelial cells to rapidly upregulate P-selectin in response to stimulation by mediators like histamine and thrombin. In addition to P-selectin, endothelial activation also involves cytokines, which promote the upregulation of other adhesion molecules, including E-selectin. The upregulation of E- selectin is a more prolonged process since it requires transcription and translation into protein before being displayed on the cell surface. L-selectins, found on the surface of leukocytes, also play a role in this process. 6 Selectins have a variety of ligands; for example, P-selectin binds to carbohydrates such as Sialyl Lewis X and other glycoproteins on leukocytes. This interaction is characterized by weak-aDinity binding, resulting in slower adhesion. In contrast, integrins represent a stable adhesion mechanism. They are composed of alpha and beta subunits, with 18 alpha subunits and 8 beta subunits that combine in numerous ways to form various integrins. Integrins are crucial during diapedesis, the process by which immune cells exit blood vessels to migrate to sites of infection. The primary integrins involved in this process are those containing the beta-2 and sometimes beta-7 subunits. A key integrin in neutrophil diapedesis is LFA-1 (alpha-L beta-2), which is specifically utilized by neutrophils to adhere to endothelial cells. Another important integrin is VLA-4, which facilitates diapedesis and contributes to various cell-to-cell interactions beyond leukocytes. Another important integrin is VLA-4 (Very Late Antigen-4), which is not only involved in diapedesis but also plays a role in various cell-to-cell contacts beyond leukocytes. While LFA- 1 is highly specific for neutrophils exiting the bloodstream, integrins like VLA-4, MAK-1, and LPMN participate in broader interactions among diDerent immune cells. VLA-4 is critical for the adhesion of leukocytes to the endothelium during inflammation and for their migration into tissues where they can exert their functions. LFA-1 and Mac-1 bind to ICAM-1 on the endothelial surface, while VLA-4 and LPAM-1 bind to another integrin ligand called VCAM-1. This interaction helps stabilize the leukocyte's position on the endothelium, leading to a process known as firm adhesion. Once firmly adhered, the leukocyte begins transendothelial migration (or diapedesis) to move through the endothelial barrier toward the site of infection or inflammation. This migration is further facilitated by integrins and an additional protein called PECAM-1 (Platelet Endothelial Cell Adhesion Molecule-1), which is expressed on both leukocytes and between endothelial cells. PECAM-1 guides leukocytes through the endothelial junctions, allowing them to exit the bloodstream and reach inflamed tissues. 7 Chemotaxis Chemotaxis is the directed movement of immune cells, such as neutrophils and macrophages, toward sites of infection or inflammation, guided by a gradient of signaling molecules called chemo attractants. This mechanism is essential for immune response, as it helps immune cells reach and neutralize pathogens while supporting tissue repair. Key chemo attractants include: Chemokines like IL-8 (CXCL8), which is crucial for directing neutrophils. Complement component C5a, generated as part of the body’s response to pathogens. Leukotriene B4 (LTB4), a lipid mediator produced by immune cells to amplify inflammation. Bacterial debris, which can attract immune cells by signaling a site of infection. The process begins with immune cells detecting the gradient of these chemoattractants. Their receptors sense the highest concentration of signals in a specific direction, which orients the cell and establishes a sort of “compass” pointing toward the infection site. Once the cell senses a strong signal, it reorients its cytoskeleton, extending a pseudopod—a temporary projection driven by actin polymerization—at its leading edge to propel itself forward. Myosin II helps retract the cell's trailing edge, allowing it to move smoothly in the direction of the signal. The cell continuously adjusts its movement, forming new pseudopods as it senses the gradient and maintains its orientation toward the strongest signals. As it moves along the gradient, the cell adheres to its environment via integrins, which form strong connections with the surrounding extracellular matrix or endothelial cells. When the immune cell reaches the blood vessel wall near the infection, it undergoes transendothelial migration (diapedesis) to cross into the infected tissue. At this stage, the cell adheres tightly to the endothelial cells lining the blood vessel, guided by both integrins and PECAM-1 (Platelet Endothelial Cell Adhesion Molecule-1). PECAM-1, present on both leukocytes and endothelial cells, facilitates the cell's movement through endothelial junctions and into the tissue. 8 Neutrophil EDector Functions Neutrophils play a crucial role in the immune response through their eWector functions, primarily by performing phagocytosis. When a neutrophil encounters a pathogen, it initiates the process of engulfing the microorganism, encapsulating it within a vesicle formed from the cell membrane. This vesicle, known as a phagosome, traps the pathogen inside. Following this, the phagosome merges with a lysosome, an organelle filled with enzymes and proteins capable of degrading pathogens. The combined vesicle, called a phagolysosome, exposes the pathogen to reactive oxygen species and antimicrobial proteins, eDectively breaking it down. In addition to phagocytosis, neutrophils can release structures known as neutrophil extracellular traps (NETs). These NETs are composed of chromatin and antimicrobial molecules expelled from the neutrophil, creating a web-like structure that traps and neutralizes pathogens outside the cell. This mechanism, known as NETosis, acts as a physical barrier to prevent the spread of bacteria, enhancing pathogen clearance. Neutrophils are also sources of inflammatory mediators. These mediators are divided into several groups: Arachidonic acid derivatives, including prostaglandins and leukotrienes, play a significant role in promoting inflammation and recruiting additional immune cells. Cytokines and chemokines, such as those listed in the table, coordinate further immune responses by stimulating immune cell activation and guiding cells to sites of infection. Additionally, neutrophils interact with the complement system, a group of proteins that facilitate inflammation, enhance phagocytosis, and directly target pathogens through processes like opsonization and membrane attack complex formation. Lastly, histamine, which is released by neutrophils and other immune cells, primarily drives vascular responses. This includes increasing blood flow and vascular permeability, allowing immune cells easier access to the infection site. The complement system operates through three main pathways: the alternative, classical, and lectin pathways. These pathways converge in the formation of C3 convertase, leading to the production of C3b, which tags pathogens for phagocytosis. Additionally, complement components such as C5a act as chemoattractants and activators for leukocytes, further coordinating the inflammatory response and supporting phagocytosis. 9 When acute inflammation occurs, it typically resolves as the body successfully eradicates the pathogen. However, if the pathogen cannot be eliminated, the inflammation may transition into a chronic phase. In cases where the body is unable to regenerate the aDected tissue, resolution is unlikely, leading instead to fibrosis. Thus, the progression from acute to chronic inflammation is marked by the persistence of the inflammatory response, often resulting in tissue damage and scarring rather than healing. 10 Dying neutrophils CHRONIC INFLAMMATION The two main cells of the chronic inflammation are the T lymphocytes and the macrophages. The chronic inflammation is a response of prolonged duration (weeks or months) in which inflammation, tissue injury and attempts at repair co-exist in varying combinations. We can consider 3 causes of chronic inflammation: Persistent infections – Bacteria, viruses, fungi and parasites Hypersensitivity – When we have an inappropriate (autoimmune diseases) or excessive response (allergic diseases) Prolonged exposure to toxic agents (endogenous/exogenous) There are many diseases caused by or aggravated by chronic inflammation and all of them are non-infectious Autoimmune disorders (IBD) – Crohn’s Disease o Colits o Type 1 Diabates Cancers – chronic inflammation help the progress of the cancer Cardiovascular Disease Neurological disease Diabetic complications Pulmonary disease Metabolic disorders 15 The key morphological features: Acute inflammation o Major cells type is the neutrophils, they come in and fill in the alveoli o Vascular response – the small vessels dilated, and all the blood stream works to get the neutrophils to the tissue with the patoghen o Edema After the infection is gone, the neutrophils there clear by macrophages and we can talk of chronic inflammation, if the pathogens is still present Chronic inflammation o Major cells involved are macrophages, T-lymphocytes, and plasma cells. Macrophages play a central role by presenting pathogen-derived antigens to T- cell receptors. When a T-cell receptor recognizes and matches an antigen presented by a macrophage, it leads to clonal expansion of the T-cells, amplifying the immune response. Plasma cells, which are indeed activated forms of B-cells, produce antibodies to target and neutralize pathogens or infected cells. o We can see the area of the alveoli that is destruct, in fact we have the tissue destruction. The destruction exceed the regenerative capacity of the tissue, and we will have this area fill by fibrosis (collagen I) or scar tissue. The chronic inflammation can also start from the day one as chronic inflammation and it is what happen if we have an inflammation from a virus, for example. 16 Cell types – blood smear VS solid tissue In this blood smear, the macrophages appear in their circulating form as monocytes, characterized by a large, bean-shaped nucleus. We also observe a lymphocyte with a large nucleus that occupies almost the entire cell, leaving very little visible cytoplasm. When lymphocytes look like this, it’s diDicult to distinguish between B and T cells based on morphology alone. Additionally, neutrophils are present with their characteristic multi-lobed (polymorphic) nucleus. When these cells move into the tissue, they appear less organized. Monocytes entering inflamed tissue become activated and diDerentiate into macrophages, identifiable by their abundant cytoplasm and a large, lighter- staining nucleus. This lighter appearance results from the conversion of dense heterochromatin to more relaxed euchromatin, allowing for increased gene expression. It can be hard to recognize the lymphocyte but, activated B cells, or plasma cells, have a distinct appearance: their cytoplasm expands as they produce immunoglobulins, and their nucleus shifts to the side. Neutrophils in tissue are recognized by their characteristic multi-lobed nucleus, which often appears as if it contains two or more connected segments, helping to distinguish them in histological slides. In this image we can cleary see the plasma cell with their characteristic clock face. They are round-to-ovoid cells containing abundant deep blue cytoplasm with a pale perinuclear area corresponding to the Golgi apparatus. They have a round, eccentrically placed nucleus with coarse chromatin arranged in a clock face (art wheel) pattern. 17 Here there is viral myocarditis, a viral infection of the heart. In the cardiomyocyte where we cannot see the nucleus because they are dead, and we can see these typical aspect of T cells. If we have that it means that we have macrophages presenting the viral proteins and clonal expansions of T protein. Macrophages There are two sources of macrophages the fetal yolk sac and the fetal liver. They migrate in the fetal stage into various tissue tissue to create what we call the resident tissue macrophages. In the liver, we refer to them as KupDer cells; in the lungs, they are known as alveolar macrophages; and in the brain, we call them microglia. These resident macrophages remain in place, ready to respond to pathogens, and are involved in both acute and chronic responses. They can persist throughout life and are replaced by proliferation. However, to combat significant infections, additional macrophages are needed, which come from the monocytes from the blood. Monocytes are derived from hematopoietic stem cells in the bone marrow, which produce blood monocytes. These cells have a very short lifespan, only about 24 hours, and circulate in the bloodstream. However, if they pass through inflamed tissue, they are recruited into it. Morphology – Monocytes have a bean-shaped nucleus with dense chromatin. When they become activated, the nucleus appears open and lightly stained, and large lysosomes become visible. 18 Functions of macrophages There are two types of macrophages with completely opposite functions: M1 and M2. M1 macrophages, also known as classically activated macrophages, are pro-inflammatory and microbicidal. When monocytes enter inflamed tissues, microbial molecules (PAMPs) can bind to Toll- like receptors on the monocyte, activating it into an active macrophage. Another activating signal can come from IFN-gamma produced by T helper cells. Once activated, M1 macrophages upregulate many cytokines, including IL-1, TNF, IL-12, and chemokines, leading to an increase in chronic inflammation. Their second major function is the killing of microbes; activated macrophages upregulate the production of toxic molecules like ROS, NO, and lysosomal enzymes. Phagocytosis, a key function of macrophages, is central to this mechanism, allowing them to kill microbes in chronic inflammation. We observe the macrophage cell membrane interacting with a pathogen. Phagocytic receptors on the macrophage bind to molecules on the pathogen's surface, enabling internalization. A lysosome, containing a cocktail of destructive enzymes, fuses with the phagosome to form a phagolysosome. This process occurs in activated macrophages and triggers a signaling pathway diDerent from that of Toll-like receptors. This pathway activates enzymes such as iNOS and phagocyte oxidase, which produce nitric oxide—a highly toxic molecule that kills bacteria. In some cases, a portion of these toxic molecules is released back onto the cell membrane, contributing to tissue destruction. This release is a key mechanism by which chronic inflammation damages tissues, as these toxic molecules can harm surrounding cells. 19 All this tissue destruction requires repair, and this is the role of M2 macrophages, also called alternatively activated macrophages. They are anti-inflammatory. M2 macrophages are activated by a diDerent set of cytokines, including IL-13 and IL-4, which promote their anti- inflammatory functions. Once activated, M2 macrophages produce anti-inflammatory cytokines, such as IL-10 and TGF-beta, which inhibit immune cell proliferation, resulting in an anti-inflammatory eDect. They also produce growth factors; however, if tissue destruction exceeds the repair capacity, fibrosis can begin. TGF-beta and growth factors activate fibroblasts, causing them to transform into myofibroblasts, which then produce collagen. It is important to note that both M1 and M2 macrophages are not terminally diDerentiated and can switch between forms depending on the predominant signals in their environment. MACROPHAGE AND T- CELL INTERACTIONS T-helper cells are another major cell type involved in chronic inflammation, and it is essential to discuss them alongside macrophages. T-helper cells exhibit many of their key functions through positive feedback and interaction with macrophages, amplifying and propagating chronic inflammation. Monocytes enter inflamed tissue and diDerentiate into classically activated macrophages (M1). These activated macrophages produce pro-inflammatory cytokines such as TNF and IL- 1, which further recruit and activate more immune cells, including T-lymphocytes, amplifying the inflammatory response. 20 Activated macrophages phagocytose microbes and present microbial proteins on MHC class II (MHC- II) molecules. If T-helper cells with matching T-cell receptors (TCRs) encounter these antigen- presenting macrophages, they become activated and proliferate, producing additional cytokines. For T-cell activation, co-stimulatory signals from antigen-presenting cells, such as macrophages, are also necessary, along with cytokines. This cocktail of signals activates T-helper cells to produce IL-2, a growth factor that promotes T-cell proliferation, leading to clonal expansion of T cells in the chronically inflamed tissue. If the predominant cytokine is IL-12, T cells will diDerentiate into TH1 cells, whose primary function is to produce IFN-gamma, a potent activator of macrophages, thus reinforcing the positive feedback loop. Conversely, if the cytokines present are IL-6 or IL-23, T cells will diDerentiate into TH17 cells, which primarily recruit neutrophils. While neutrophils are present in chronic inflammation, they do not dominate the inflammatory response. We have diDerent subtypes of T-helper cells depending on which cytokines are produced by the antigen-presenting cells. If the cytokines are IL-12 or IFN-gamma, T-helper cells diDerentiate into TH1 cells. The defining cytokine they produce is IFN-gamma, which activates macrophages to help combat intracellular pathogens. However, this response can also be involved in disease reactions that occur in chronic inflammatory diseases or autoimmunity. If the cytokines are IL-6 and IL-23, we get TH17 cells. The defining cytokines are IL-17 and IL-22, which recruit neutrophils to assist in fighting extracellular pathogens. This pathway can also be implicated in autoimmune diseases. If IL-4 is present, T-helper cells diDerentiate into TH2 cells. They can produce IL-4, which acts on B cells to activate them and promote the production of IgE. TH2 cells also produce IL-5, which activates eosinophils. This response typically occurs during infections caused by helminths. The antibodies (immunoglobulins) will coat the helminths, allowing eosinophils to recognize them and secrete granules filled with neurotoxic proteins that can eDectively kill the helminths. However, this reaction can also contribute to allergic hypersensitivity diseases, as these molecules can harm surrounding tissues. 21 Eosinophils (in blue) contain granules filled with toxic proteins and are classified as polymorphonuclear leukocytes. Activated B cells (in green) diDerentiate into plasma cells, characterized by a nucleus positioned in the corner and an extensive Golgi apparatus, reflecting their role in producing antibodies. Macrophages (in red) can have a nucleus typically eccentric, often appearing oval or kidney- shaped, they may have a foamy cytoplasm due to the presence of phagocytized material and lipid droplets. 22 Plasma Cells Plasma cells are specialized B lymphocytes that produce large quantities of immunoglobulins (antibodies). When a B lymphocyte encounters an antigen that is presented on MHC molecules on the surface of antigen-presenting cells, it binds to the antigen through its surface antibodies. This interaction, along with additional signals from T- helper cells (particularly cytokines like IL-4 and IL-5), activates the B cell. Once activated, the B cell undergoes clonal expansion, proliferating to generate a population of identical B cells. Some of these cells diDerentiate into plasma cells, which have an extensive endoplasmic reticulum for synthesizing antibodies. Although plasma cells secrete a significant amount of immunoglobulins, their cytoplasm may appear relatively sparse because the antibodies are secreted immediately into the extracellular space. This rapid secretion is crucial for the eDective neutralization of pathogens, playing a key role in the humoral immune response. An important point to note is that T-helper cells do not directly kill pathogens; instead, they produce mediators and signaling proteins that recruit or activate other immune cells responsible for microbial killing. However, some T cells can directly target and kill pathogens, specifically CD8+ T cells. These cells are capable of killing cells infected with cytoplasmic pathogens, such as in cases of chronic viral hepatitis in the liver. In the liver, hepatocytes can become infected by viruses, leading to chronic inflammation. CD8+ T cells recognize and attack these infected hepatocytes, inducing cell death through apoptosis. The infected hepatocytes contain viral proteins that are recognized by the activated CD8+ T cells, which then secrete granules containing perforin and granzyme B. 23 Perforin is a protein that forms pores in the plasma membrane of the target hepatocyte, allowing granzyme B to enter the cell. Granzyme B is a serine protease that cleaves key proteins and activates caspases, leading to the apoptotic pathway and ultimately resulting in cell death. Additionally, CD8+ T cells can upregulate Fas ligand (FasL), which binds to the Fas receptor on the surface of the infected hepatocyte. This interaction also activates the caspase cascade, further promoting apoptosis. The red area indicates scar tissue, highlighted by Sirius Red staining, which specifically marks fibrosis. The brown region represents islands of hepatocytes that are surrounded by this scar tissue (fibrosis). Granulomatous Inflammation Granuloma is a subtype of chronic inflammation characterized by aggregates of activated macrophages. In this distinctive morphology, macrophages cluster together to form a large mass, diDerentiating it from T-lymphocyte responses. Granulomas often arise in response to infections or irritants that are diDicult for the body to eliminate. There are two main types of granulomas: foreign body granulomas and immune granulomas. Foreign body granulomas develop when non- degradable materials enter the body, such as talc, sutures, or certain types of implants. These materials are recognized by macrophages, which surround them in an attempt to degrade them. This results in the formation of a prominent clump of macrophages (red), often including multinucleated giant cells that form from the fusion of macrophages around the foreign material. Giant cells are a characteristic feature of foreign body granulomas. Immune granulomas occur in response to persistent pathogens, such as mycobacteria (e.g., tuberculosis), fungi, or parasites. While giant cells may also be present in immune granulomas, they primarily consist of activated macrophages that are attempting to contain the infection. 24 Importantly, granulomas typically exhibit a predominance of macrophages, with T-cells often being absent or present in minimal amounts. The localized aggregation of macrophages reflects the body’s response to contain a persistent irritant or pathogen that cannot be easily eliminated. Immune granulomas are characterized by a dense aggregation of activated macrophages and are commonly associated with infections like tuberculosis, which is caused by Mycobacterium tuberculosis—one of the most significant infectious disease killers globally. Granulomatous inflammation can be induced by various diseases, with tuberculosis being a prime example. In the lungs aDected by tuberculosis, the granulomas appear as purple structures on histological slides, indicating areas of significant tissue disruption that may ultimately require repair through fibrosis. Other conditions that can lead to granuloma formation include leprosy and syphilis, although these diseases are not as dangerous today due to advancements in treatment. Additionally, granulomas can be observed in non-infectious conditions such as sarcoidosis and Crohn's disease. When a granuloma is identified, it often provides a clear and rapid diagnostic clue to underlying pathology, enabling clinicians to initiate appropriate management. Inside the granuloma, there is a prominent necrotic core (blue) due to the lack of blood supply, resulting in a chaotic environment filled with activated macrophages. The activation of these macrophages is upregulated by toxic molecules released by the pathogens, contributing to the formation of this necrotic center. Surrounding the necrotic core, we find a dense aggregation of macrophages known as epithelioid cells. Some of these epithelioid cells may fuse to form Langhans giant cells; their presence is a strong indicator of a granuloma, although giant cells are not always observed. 25 Surrounding this core are T cells, which play a crucial role in the formation of granulomas through a positive feedback mechanism. Macrophages recognize and phagocytize Mycobacterium tuberculosis, but these bacteria express proteins that inhibit the fusion of the phagosome with the lysosome, thereby evading destruction. Some of the proteins secreted by the bacteria are presented on MHC molecules, which interact with T cells, leading to their activation. In particular, the activation of TH1 cells occurs, which produce TNF and IFN-gamma. These cytokines help recruit monocytes and activate more macrophages, perpetuating the cycle of inflammation and ultimately resulting in the formation of the granuloma. 26 TISSUE REPAIR AND HEALING Repair and healing refer to the processes involved in restoring damaged tissue, which can occur through two primary mechanisms: regeneration and scar formation. Regeneration involves the complete restoration of the tissue to its original state, meaning the tissue is identical to how it was before the injury. This process is often observed in tissues with a high capacity for cell division. Scar Formation, on the other hand, results in partial replacement of the damaged tissue with connective tissue, leading to an imperfect restoration. This occurs when the tissue cannot regenerate fully. The ability of a tissue to restore itself can vary between these two mechanisms, with some tissues exhibiting a greater capacity for regeneration, while others are more likely to form scars. Labile Tissues: These are tissues with a high degree of cell division, such as most surface epithelial tissues. They predominantly undergo regeneration, allowing for eDective healing and restoration of function. Permanent Tissues: In contrast, tissues such as cardiac muscle and brain tissue contain non-proliferative cells in adults. These tissues generally do not regenerate and instead rely on scar formation for repair, which can lead to functional deficits. Stable Tissues: Some tissues, like the liver and kidneys, fall in between the two extremes. These are known as stable tissues, characterized by quiescent cells that possess a limited capacity for proliferation. In these tissues, both regeneration and scar formation can occur, depending on the extent of the damage. 33 Liver Regeneration The liver is composed of various cell types, with the major cell type being hepatocytes. Under normal circumstances, hepatocytes are typically quiescent and do not divide frequently. However, in response to liver injury or wounding, hepatocytes transition from the G0 phase to the G1 and S phases of the cell cycle. This transition signifies that hepatocytes can potentially re-enter the cell cycle and behave like stem cells, which is a key factor in the liver's remarkable regenerative capabilities. In addition to hepatocytes, the liver contains other important cell types that contribute to its regenerative process: Stellate Cells: These cells, also known as hepatic stellate cells or Ito cells, play a crucial role in producing extracellular matrix components. They are involved in the regulation of liver fibrosis and participate in the liver's response to injury by producing collagen and other matrix proteins that support tissue repair. KupWer Cells: These specialized macrophages reside in the liver and are vital for immune responses. They help to clear pathogens and dead cells from the liver, thereby playing a significant role in maintaining the liver's health and facilitating regeneration after injury. The interplay between these diDerent cell types enables the liver not only to recover from damage but also to restore its structure and function eDectively. This regenerative capacity is unique among organs, allowing the liver to withstand substantial damage and still return to a functional state. How it is regulated? Liver regeneration is intricately regulated by a mechanism known as the gut-liver axis. This connection highlights how the gut influences liver function and proliferation. The intestines harbor a vast array of bacteria that are beneficial for our health; however, it is crucial that these bacteria remain confined to the intestinal lumen and do not enter the bloodstream. The intestinal epithelium plays a critical role in maintaining this barrier, supported by antibodies and immune cells that ensure tight junctions. In disease conditions, the integrity of this barrier may be compromised, leading to increased permeability. As a result, bacterial products can enter the bloodstream and reach the liver. The liver, in response, produces factors that help fortify the intestinal barrier. If liver size is reduced—such as in cases of liver disease—this barrier function may decline, allowing even more bacterial products to enter the liver. Once these bacterial products reach the liver, they activate KupWer cells, which are specialized macrophages. Activated KupWer cells secrete inflammatory cytokines, including IL-8 and TNF-alpha. These cytokines are crucial for signaling hepatocytes to initiate the regenerative process. 34 However, liver regeneration is not solely influenced by gut-derived signals. Other organs, such as the thyroid, pancreas, adrenal glands, and duodenum, also send signals that aDect liver regeneration. This creates a complex network of regulatory pathways, and if any component within this system is dysfunctional, the regenerative response may be impaired. Once activation occurs and the liver starts its regenerative process, the barrier function improves, reducing the influx of bacterial products into the bloodstream. This feedback loop helps to restore normal liver function. However, excessive alcohol consumption can trigger this activation pathway, but over time, the liver's regenerative capacity may become diminished. Hepatocytes may reach a point where they can no longer proliferate, even after many years of adaptation and regeneration. Stem Cells and Liver Regeneration The liver contains a population of stem cells that reside in the canals of Hering, located between cholangiocytes (cells lining bile ducts) and hepatocytes (on the liver side). These stem cells share the same progenitor, known as liver progenitor cells (LPCs). When liver function is compromised, these LPCs become activated, diDerentiating into both hepatocytes and cholangiocytes. However, their regenerative potential is limited, and they cannot fully restore liver function on their own. The presence of stem cells oDers an advantage, especially in chronic diseases, as they provide a basis for regeneration. Regenerative medicine leverages this capacity, using techniques to reprogram fibroblasts (connective tissue cells) into stem cells for various tissues. This process has the potential to generate cells that resemble the original cells needed for tissue repair. Despite its promise, regenerative medicine faces challenges. While the reprogrammed cells may look like the target cells, their function may diDer significantly, limiting the eDectiveness of the regeneration. Another major concern is that pluripotent stem cells, which can diDerentiate into any cell type, also have the potential to form tumors if not carefully regulated. 35 Repair involving scar formation One standard example of tissue repair is skin wound healing. The upper part of the skin is the epidermis, which consists primarily of keratinocytes. Below this layer lies the connective tissue, rich in extracellular matrix, containing fibroblasts, macrophages, and lymphocytes. In the dermis, we have blood vessels, and in the subcutis, there is fat. When a wound occurs, the first response is blood coagulation, resulting in a provisional matrix made primarily of fibrin and fibronectin. This temporary matrix helps prevent blood loss, but it is relatively soft compared to normal matrix tissue, making it mechanically weak and not an eDective barrier against bacteria. Therefore, it only serves as a temporary fix that must be replaced quickly. The healing process begins with an inflammatory reaction, where neutrophils migrate to the wound site to fight oD any pathogens that have entered. After about two days, macrophages replace the neutrophils. These macrophages not only remove the temporary matrix but also induce fibroblasts to produce a stronger extracellular matrix. This process is further supported by the formation of new blood vessels, which increases vascularization and promotes the transport of nutrients and cells to the area. This stage, characterized by inflammation and the presence of new vessels and fibroblasts, is referred to as granulation tissue. As healing progresses, the immune cells gradually disappear, leaving behind primarily the extracellular matrix. The activated fibroblasts become inactive, and excess blood vessels regress. Upon careful examination, the extracellular matrix molecules may not completely resemble normal tissue, and it can take many weeks for changes in the matrix to stabilize. In the epidermis, the wound stimulates the proliferation of keratinocytes from both sides, prompting them to migrate toward the wound site. Once the wound is closed, what remains is a scar, which will be formed as keratinocytes continue to migrate and re-epithelialize the area. 36 Macrophages Macrophages are a type of immune cell that play a crucial role in tissue homeostasis, immune response, and repair processes. These cells are typically identified by specific cell surface markers, though not all markers are present on every macrophage. There are two primary types of macrophages: M1 and M2. M1 Macrophages: These macrophages are associated with the inflammatory response. They produce pro-inflammatory cytokines such as TNF-alpha and reactive oxygen species (ROS). M1 macrophages are typically involved in fighting infections and initiating the immune response against pathogens. M2 Macrophages: In contrast, M2 macrophages are involved in the healing and repair process. They secrete anti-inflammatory cytokines and growth factors that promote the activation of fibroblasts and support fibrosis. M2 macrophages help to resolve inflammation and facilitate tissue repair and remodeling. Between the M1 and M2 types, there is likely a continuum of macrophage states, reflecting their plasticity and ability to adapt to diDerent microenvironments. Various factors, including the type of injury and the surrounding conditions, can influence the polarization of macrophages, leading to the diDerentiation into either M1 or M2. Injuries induce macrophages to produce diDerent signaling molecules depending on the type of tissue damage and the specific macrophage subtype activated. This variability allows for a tailored response to injury, ensuring that the appropriate type of macrophage is present to facilitate healing or combat infection. 37 Wound healing occurs in several distinct phases. Initially, neutrophils migrate to the wound site within the first two days, playing a crucial role in combating pathogens and clearing debris. Following this, neutrophils are replaced by macrophages, which continue the cleanup process and initiate the next stages of healing. As healing progresses, we observe the proliferation and activation of fibroblasts. These fibroblasts are essential for synthesizing the extracellular matrix and promoting tissue repair. During this phase, fibroblasts migrate to the wound area, where they contribute to forming a more stable matrix. An important factor that regulates the timing and coordination of these cellular waves is the oxygen level in the tissue. When a wound occurs and bleeding is present, blood supply to the area may be compromised, leading to a dramatic reduction in oxygenation. This hypoxic condition serves as a critical trigger for the restoration of new blood vessels, promoting angiogenesis and increasing oxygen availability in the wound area. Once oxygen levels rise again, this shift plays a key role in modulating the wound healing process. The balance of oxygen is crucial; too little can delay healing, while optimal levels support the necessary cellular functions involved in tissue repair. In summary, the interplay between neutrophils, macrophages, and fibroblasts, along with the regulation of oxygen levels, is vital for eDective wound healing. Granulation Tissue Granulation tissue is a transitory type of connective tissue that forms during the healing process of a wound. When you observe a wound, it may appear red due to the increased number of blood vessels in the area. The whitish regions surrounding the wound are typically made up of keratinocytes. Granulation tissue is characterized as a loose connective tissue, which provides a scaDold for the repair process. The formation of new blood vessels, a process known as angiogenesis, 38 is primarily driven by key growth factors such as VEGF-A (Vascular Endothelial Growth Factor A) and FGF-2 (Fibroblast Growth Factor 2). These factors stimulate endothelial cells to proliferate and form new vascular structures. Additionally, the activation of fibroblasts is crucial for the development of granulation tissue. This activation is mediated by TGF-beta (Transforming Growth Factor-beta), which is essential for fibroblast activation, and PDGF (Platelet-Derived Growth Factor), which promotes fibroblast proliferation. Within this environment, you can also find M2 macrophages, which play a significant role in modulating inflammation and supporting tissue repair. M2 macrophages release anti- inflammatory cytokines and growth factors that further facilitate the healing process. Myofibroblast produce ECM and are contractile Myofibroblasts are specialized cells that play a crucial role in the production of the extracellular matrix (ECM) and are also contractile. They can synthesize various ECM components, including collagen, and are primarily found in connective tissue. DiDerent tissues can have distinct types of fibroblasts, reflecting the specific needs and functions of those tissues. A fibroblast can diDerentiate into a myofibroblast under certain conditions. This transformation is often induced by cytokines such as TGF-beta (Transforming Growth Factor- beta) and mechanical stress. When fibroblasts experience stretching or mechanical tension, they initiate the production of ECM, which is a normal and essential part of tissue repair. Myofibroblasts derive their name from their muscle-like characteristics, particularly the presence of actin filaments, which grant them a contractile ability. This contractility is important for wound healing, as it helps to pull the edges of a wound together, facilitating closure. However, once the tissue has healed and is no longer under stress, the presence of myofibroblasts may not be necessary. In such cases, these cells typically undergo apoptosis (programmed cell death) or revert to a more quiescent fibroblast state to maintain tissue homeostasis. 39 Remodeling of Connective Tissue The remodeling of connective tissue is a critical phase in the wound healing process and involves several key factors: Activated Fibroblasts: These cells play a central role in remodeling by synthesizing and modifying the extracellular matrix (ECM). ECM Synthesis: During healing, diDerent types of collagen are produced. Collagen Type III is typically synthesized in the early stages of wound healing, especially in wounds with high vascularity due to the presence of blood. As healing progresses, there is a shift to Collagen Type I, which provides greater tensile strength to the tissue. The composition of collagen in the ECM is crucial for maintaining tissue integrity and strength. ECM Degradation: The degradation of the ECM is primarily mediated by matrix metalloproteinases (MMPs), a group of enzymes that have specificity for diDerent ECM components. The activity of MMPs can be regulated, as they control the breakdown of collagen and other ECM proteins, which is essential for tissue remodeling. Inhibition of ECM Degradation: The activity of MMPs is counterbalanced by tissue inhibitors of metalloproteinases (TIMPs), which prevent excessive degradation of the ECM, ensuring a proper balance between synthesis and breakdown during remodeling. 40 Reduction of Excess Blood Vessels: Apoptosis, or programmed cell death, helps to reduce the number of newly formed blood vessels, restoring normal vascularity as the wound heals. In cases of larger wounds, the healing process is referred to as healing by secondary intention. This occurs when a wound is too large for primary closure, resulting in a more complex healing process. In such situations, the focus is on managing the wound environment to promote healing rather than closing it directly. There are several factors that can impair tissue repair, including: Infection: Can complicate healing and increase the risk of further tissue damage. Diabetes: Often leads to delayed healing due to poor circulation and impaired immune function. Poor Nutritional Status: Essential nutrients are required for optimal healing. Glucocorticoids: While used to treat inflammatory diseases, these can negatively aDect wound healing. Mechanical Factors: Movement or tension on the wound can disrupt healing. Poor Perfusion: InsuDicient blood supply to the area can impair nutrient delivery and waste removal. Foreign Bodies: The presence of foreign materials can trigger an inflammatory response and delay healing. Fibrosis (Pathological scarring) Fibrosis is a form of pathological scarring marked by excessive ECM (extracellular matrix) production, usually due to chronic injury or persistent inflammation, which often reduces organ function. In fibrosis, the ECM is primarily composed of collagen types I, III, and IV as well as elastin; initially, collagen III is abundant in blood-rich wound areas but gradually gets replaced by collagen I, adding stiDness to the tissue. Fibrosis progresses through distinct stages, each characterized by specific ECM components, with varying types and quantities of proteins based on the fibrosis stage. The primary cells involved in fibrosis are macrophages, which release cytokines such as TGF-beta that activate myofibroblasts. These cells, derived from fibroblasts, produce a 41 significant amount of collagen and have contractile properties due to actin fibers. This contraction is essential in normal wound healing but problematic in fibrosis, where excessive ECM production leads to rigid, non-functional tissue. To regulate ECM levels, the body uses matrix metalloproteinases (MMPs), enzymes that degrade the matrix, and TIMPs, which inhibit MMPs. Excess TIMPs, however, prevent ECM degradation, causing matrix accumulation and worsening fibrosis. In alcoholic liver cirrhosis, chronic alcohol-induced injury progressively damages liver function to the point where the only eDective treatment is often a liver transplant. Microscopically, cirrhotic liver tissue shows a buildup of collagen matrix (in blue), surrounding the hepatocytes (shown in pink/red), which are attempting regeneration but ultimately undergoing cell death due to constant inflammation. This regenerative process is impaired by the surrounding collagen, which forms fibrotic scar tissue around the hepatocytes, leading to fibrosis. One major consequence of fibrosis in cirrhosis is portal hypertension. Normally, the liver's fenestrated endothelium allows blood to flow through and come into indirect contact with hepatocytes, facilitating eDicient filtration and nutrient exchange. However, in cirrhosis, this fenestration closes up due to excessive scarring, restricting blood flow and preventing hepatocytes from properly accessing the blood. As a result, blood cannot flow freely through the liver, leading to high blood pressure within the portal vein system. In cirrhotic patients, the architecture of the liver is so severely distorted by fibrosis that it can no longer repair itself. While acute liver damage can often heal over time, the irreversible architectural changes in cirrhosis mean that liver transplantation may be the only solution to restore function, as regeneration alone is not enough to recover from such chronic, widespread damage. Liver fibrosis significantly increases the risk of developing liver cancer, particularly in the context of any chronic liver damage that can progress to fibrosis. The rising incidence of liver cancer is closely associated with factors such as excessive alcohol consumption and diets high in fat. Both fibrosis and chronic inflammation are key contributors to the development of liver cancer, as they create an environment conducive to cellular changes and mutations. Over time, the combination of these factors can lead to the transformation of normal liver cells into cancerous cells, exacerbating the health risks associated with liver disease. 42 SCLERODERMA Scleroderma, also known as systemic sclerosis, is an autoimmune disease characterized by an abnormal autoimmune reaction that leads to excessive production of extracellular matrix (ECM) throughout the connective tissue, resulting in significant fibrosis and stiDness. One common manifestation of scleroderma is Raynaud’s syndrome, where the contraction of certain arteries reduces blood flow to the fingertips, leading to tissue ischemia and necrosis. Microscopically, the skin of individuals with scleroderma shows a marked increase in collagen, which stains red, indicating a much denser and stiDer tissue composition. This excessive collagen production is accompanied by a diDerentiation of many stem cells into fibroblasts, contributing further to the ECM buildup. As a result of this fibrotic process, there is a loss of fat and sweat glands in the skin, leading to profound changes in skin texture and function, ultimately aDecting the overall health and quality of life of aDected individuals. Aging significantly contributes to the development of fibrosis in the heart, aDecting its overall function. The heart is normally surrounded by collagen, which is essential for its contraction. However, as individuals age, collagen production increases, leading to a stiDer heart muscle. While the heart may still contract, its ability to expand elastically becomes compromised due to the excessive collagen buildup. 43 Fibroblasts, which make up about 20% of the normal heart tissue, become activated with age and begin producing more collagen. This process is further aggravated in individuals with heart disease. As a result, the heart becomes increasingly stiD, and although cardiac muscle cells (cardiomyocytes) may hypertrophy—growing larger and stronger in an attempt to compensate for the stiDness—the overall heart function declines. This hypertrophy is characterized by swelling of the cardiomyocytes, which initially enhances the heart's contractility but ultimately leads to reduced eDiciency and potential heart failure. In addition to these changes, the extracellular matrix (ECM) within the heart is also altered, which can further impair cardiac function. Collectively, these factors contribute to the increased risk of heart failure in aging populations and those with pre- existing heart conditions. Myocardial infarction can lead to rapid cell death of cardiomyocytes, which are then replaced by fibrotic tissue in a process known as replacement fibrosis. This acute reaction occurs as the heart attempts to heal itself after the loss of functional muscle cells. During this process, the normal cardiomyocytes, which typically have a prominent central nucleus, are replaced by dense connective tissue, leading to reduced heart function and contractility due to the absence of functional muscle cells. In addition to myocardial infarction, fibrosis also plays a significant role in cancer, a phenomenon known as desmoplasia. In this context, cancer cells stimulate the production of extracellular matrix components, resulting in an increase in collagen and activated fibroblasts surrounding the tumor. The presence of large nuclei, characteristic of cancer cells, is often observed alongside the red-stained collagen in the fibrotic tissue. Desmoplasia contributes to the aggressiveness and invasiveness of malignant tumors, as the dense connective tissue not only alters the tumor microenvironment but also facilitates the cancer's ability to invade surrounding tissues. This interaction between cancer cells and the extracellular matrix can ultimately impact treatment outcomes and disease progression. 44 Fibrosis can occur in response to persistent tissue damage Fibrosis can develop as a consequence of various factors, including persistent tissue damage from autoimmune diseases like scleroderma, chronic intoxication such as alcoholic liver disease, healing through connective tissue replacement following myocardial infarction, idiopathic causes, and cancer. Unlike normal wound healing, where the healing process resolves once the injury has been repaired, fibrosis often persists due to ongoing damage and dysregulated repair mechanisms. This failure to halt the fibrotic process can lead to progressive scarring and eventual organ dysfunction. Myofibroblasts play a crucial role in fibrosis, originating from various precursor cells, with resident fibroblasts being the primary source. Other sources include smooth muscle cells and hematopoietic cells. These myofibroblasts are characterized by their ability to produce extracellular matrix components, such as collagen, which contribute to tissue stiDness and scarring. In pathological conditions, the activation and proliferation of myofibroblasts can become excessive, leading to an imbalance between ECM synthesis and degradation. This dysregulation prevents the restoration of normal tissue architecture and function, reinforcing the fibrotic state. Additionally, chronic inflammation and microenvironmental changes can further promote myofibroblast activation and persistence, perpetuating the cycle of fibrosis. In conditions like scleroderma, the autoimmune response leads to continuous ECM production and tissue stiDness, while in the case of chronic liver damage, the ongoing insult from toxins fuels the fibrotic process. The interplay between myofibroblasts, ECM components, and inflammatory signals is critical in understanding the mechanisms driving fibrosis and its long-term consequences on organ function. 45 TGF-beta Signaling is Crucial for Fibrosis Transforming growth factor-beta (TGF-β) is a pivotal cytokine in the fibrotic process, promoting the activation of myofibroblasts and the excessive production of extracellular matrix (ECM) components. TGF-β signaling initiates a cascade of events leading to fibrosis by stimulating fibroblast proliferation, collagen synthesis, and the inhibition of ECM degradation. Consequently, targeting TGF-β signaling pathways has emerged as a promising therapeutic strategy to inhibit fibrosis progression. Agents that block TGF-β signaling can potentially reverse or halt the fibrotic process, restoring normal tissue architecture and function. Can We Eliminate Myofibroblasts? Myofibroblasts play a significant role in fibrosis due to their ability to produce collagen and other ECM components. Strategies to eliminate myofibroblasts include: Induction of Apoptosis: Inducing programmed cell death can reduce the number of activated myofibroblasts in fibrotic tissues. Induction of Senescence: Promoting a senescent state in myofibroblasts leads to decreased ECM production and altered cellular functions. Killing by Immune Cells: Utilizing the immune system to target and destroy myofibroblasts can help mitigate fibrosis. DediWerentiation: Encouraging myofibroblasts to revert to a less activated fibroblast state can help alleviate the fibrotic response and restore normal tissue function. Fibrosis is Counteracted by ECM Degradation Extracellular matrix degradation is a crucial mechanism to counteract fibrosis. Matrix metalloproteinases (MMPs) are key enzymes that degrade various components of the ECM, facilitating tissue remodeling and repair. The balance between MMPs and their inhibitors, tissue inhibitors of metalloproteinases (TIMPs), regulates ECM turnover. In fibrotic diseases, this balance is often disrupted, leading to excessive ECM accumulation. Therapeutic strategies that enhance MMP activity or inhibit TIMPs are being explored to promote ECM degradation and reduce fibrosis, ultimately restoring tissue homeostasis. Fibrotic Diseases Fibrotic diseases encompass a range of pathological conditions characterized by excessive ECM deposition and tissue scarring. Examples include liver cirrhosis, pulmonary fibrosis, and scleroderma. In these diseases, persistent injury, inflammation, and dysregulation of fibroblast activity lead to extensive fibrous tissue formation, significantly impairing organ 46 function. Fibrotic diseases often have a poor prognosis and can result in organ failure. Current research focuses on understanding the underlying mechanisms of fibrosis to develop targeted therapies aimed at preventing or reversing the fibrotic process. Inflammatory Exudate Might Organize Inflammatory exudate refers to the fluid and cellular debris that accumulate during inflammation. This exudate can undergo several processes: Digestion of Exudate: The resolution phase involves the breakdown of exudate components, allowing for tissue repair and restoration of homeostasis. Granulation Tissue Formation: In the process of organizing, granulation tissue develops from the exudate, characterized by new connective tissue and blood vessels. This tissue provides a scaDold for further healing. Connective Tissue Formation and Organization: The granulation tissue matures into organized scar tissue, which can replace lost tissue. In the case of organized pneumonia, this process results in the formation of fibrotic nodules within the lung, altering pulmonary function and potentially leading to respiratory complications. 47