Aircraft Cleaning and Corrosion Control PDF
Document Details
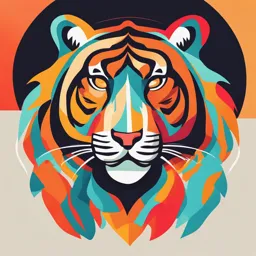
Uploaded by ValuableBowenite2530
Tags
Related
- Chemical Fundamentals of Corrosion PDF
- CASA B-06a Aircraft Materials and Corrosion PDF 2022
- Aircraft Defects, Corrosion Inspection, Assessment and Reprotection PDF
- EASA Module 06 Materials and Hardware PDF
- 8-STP Airframe Maintenance Procedures PDF
- GTEquiz4mod15.22 Engine Storage & Preservation PDF (CASA Part 66)
Summary
This document provides an overview of aircraft cleaning and corrosion control, focusing on the various metal alloys used in aircraft construction and their susceptibility to corrosion. It details the different forms of corrosion, corrosive agents, and preventive maintenance measures. It also includes a table summarizing the types of attack various alloys are susceptible to.
Full Transcript
Home Cleaning and Corrosion Control SEARCH Aircraft Cleaning and Corrosion Control Search...
Home Cleaning and Corrosion Control SEARCH Aircraft Cleaning and Corrosion Control Search FLYING TRAINING Airplane Flying Helicopter Flying Instrument Flying Many aircraft structures are made of metal, and the most insidious form of damage to those structures is Aeronautical Knowledge corrosion. From the moment the metal is manufactured, it must be protected from the deleterious effects Aviation Instructor of the environment that surrounds it. This protection can be the introduction of certain elements into the base metal, creating a corrosion-resistant alloy, or the addition of a surface coating of a chemical Insrtument Procedures conversion coating, metal, or paint. While in use, additional moisture barriers, such as viscous lubricants and protectants, may be added to the surface. The introduction of airframes built primarily of composite components has not eliminated the need for careful monitoring of aircraft with regard to corrosion. The airframe itself may not be subject to corrosion; however, the use of metal components and accessories within the airframe means the aircraft maintenance technician (AMT) must be on the alert for the evidence of corrosion when inspecting any aircraft. This section provides an overview to the problems associated with aircraft corrosion. For more in-depth information on the subject, refer to the latest edition of the Federal Aviation Administration (FAA) Advisory Circular (AC) 43-4, Corrosion Control for Aircraft. The AC is an extensive handbook that deals with the sources of corrosion particular to aircraft structures, as well as steps the AMT can take in the course of maintaining aircraft that have been attacked by corrosion. Metal corrosion is the deterioration of the metal by chemical or electrochemical attack. This type of damage can take place internally, as well as on the surface. As in the rotting of wood, this deterioration may change the smooth surface, weaken the interior, or damage or loosen adjacent parts. Water or water vapor containing salt combines with oxygen in the atmosphere to produce the main source of corrosion in aircraft. Aircraft operating in a marine environment, or in areas where the atmosphere contains industrial fumes that are corrosive, are particularly susceptible to corrosive attacks. [Figure 1] Figure 1. Seaplane operations If left unchecked, corrosion can cause eventual structural failure. The appearance of corrosion varies with the metal. [Figure 2] ··· Alloy Type of attack to which alloy is susceptible Appearance of corrosion product Magnesium Highly susceptible to pitting White, powdery, snow-like mounds and white spots on surface Low alloy Surface oxidation and pitting, surface, and Reddish–brown oxide (rust) steel(4,000– intergranular 8,000 series) Aluminum Surface pitting, intergranular, exfoliation White–to–grey powder stress– corrosion and fatigue cracking, and fretting Titanium Highly corrosion resistant; extended or No visible corrosion products at repeated contact with chlorinated solvents low temperature. Colored may result in degradation of the metal’s surface oxides develop above structural properties at high temperature 700 °F (370 °C) Cadmium Uniform surface corrosion; used as sacrificial From white powdery deposit to plating to protect steel brown or black mottling of the surface Stainless steels Crevice corrosion; some pitting in marine Rough surface; sometimes a (300–400 series) environments; corrosion cracking; uniform red, brown, stain intergranular corrosion (300 series); surface corrosion (400 series) Nickel–base Generally has good corrosion resistant Green powdery deposit (Inconel, Monel) qualities; susceptible to pitting in sea water Copper–base Surface and intergranular corrosion Blue or blue–green powdery Brass, Bronze deposit Chromium Pitting (promotes rusting of steel where pits No visible corrosion products; (Plate) occur in plating) blistering of plating due to rusting and lifting Silver Will tarnish in the presence of sulfur Brown–to–black film Gold Highly corrosion resistant Deposits cause darkening of reflective surfaces Tin Subject to whisker growth Whisker–like deposit Figure 2. Corrosion of metals On the surface of aluminum alloys and magnesium, it appears as pitting and etching and is often combined with a gray or white powdery deposit. On copper and copper alloys, the corrosion forms a greenish film; on steel, a reddish corrosion byproduct commonly referred to as rust. When the gray, white, green, or reddish deposits are removed, each of the surfaces may appear etched and pitted, depending upon the length of exposure and severity of attack. If these surface pits are not too deep, they may not significantly alter the strength of the metal; however, the pits may become sites for crack development, particularly if the part is highly stressed. Some types of corrosion burrow between the inside of surface coatings and the metal surface, spreading until the part fails. CONTENTS Factors Affecting Corrosion Types of Corrosion Forms of Corrosion Corrosive Agents Preventive Maintenance and Inspection Corrosion Prone Areas Aircraft Corrosion Removal Corrosion of Ferrous Metals, Aluminum, Magnesium Alloys and Treatment Protection of Dissimilar Metal Contacts and Corrosion Limits Chemical Treatments and Protective Paint Finishes Aircraft and Powerplant Cleaning Aircraft Cleaners ··· Tags Cleaning and Corrosion Control General Maintenance Sh Previous Post Next Post Sponsored Content Recommended by Upgrade to Office 2024 (Get Now) ps.sbs Get More Out of Your Browsing Experience With our Custom Content DiscoveryFeed Contact | Privacy | Terms | Advertise Copyright @ 2024 Aeronautics-Guide --> HOME AIRFRAME POWERPLANT GENERAL MAINTENANCE QUESTION BANK AVIATION GLOSSARY HANDBOOKS SPONSORSHIP Home Aircraft Materials SEARCH Precipitation Heat Treating and Annealing of Aluminum Search Alloys FLYING TRAINING Airplane Flying Helicopter Flying Instrument Flying Aeronautical Knowledge Precipitation Heat Treating Aviation Instructor Insrtument Procedures The aluminum alloys are in a comparatively soft state immediately after quenching from a solution heat- treating temperature. To obtain their maximum strengths, they must be either naturally aged or precipitation hardened. During this hardening and strengthening operation, precipitation of the soluble constituents from the supersaturated solid solution takes place. The strength of the material increases (often by a series of peaks) until a maximum is reached, as precipitation progresses. Further aging, or over-aging, causes the strength to steadily decline until a somewhat stable condition is obtained. The submicroscopic particles that are precipitated provide the keys or locks within the grain structure and between the grains to resist internal slippage and distortion when a load of any type is applied. In this manner, the strength and hardness of the alloy are increased. Precipitation hardening produces a great increase in the strength and hardness of the material with corresponding decreases in the ductile properties. The process used to obtain the desired increase in strength is therefore known as aging or precipitation hardening. The strengthening of the heat-treatable alloys by aging is not due merely to the presence of a precipitate. The strength is due to both the uniform distribution of a finely dispersed submicroscopic precipitate and its effects upon the crystal structure of the alloy. The aging practices used depend upon many properties other than strength. As a rule, the artificially- aged alloys are slightly over-aged to increase their resistance to corrosion. This is especially true with the artificially-aged, high-copper content alloys that are susceptible to intergranular corrosion when inadequately aged. ··· The heat-treatable aluminum alloys are subdivided into two classes: those that obtain their full strength at room temperature and those that require artificial aging. The alloys that obtain their full strength after 4 or 5 days at room temperature are known as natural- aging alloys. Precipitation from the supersaturated solid solution starts soon after quenching, with 90 percent of the maximum strength generally being obtained in 24 hours. Alloys 2017 and 2024 are natural- aging alloys. The alloys that require precipitation thermal treatment to develop their full strength are artificially-aged alloys. However, these alloys also age a limited amount at room temperature, the rate and extent of the strengthening depending upon the alloys. Many of the artificially-aged alloys reach their maximum natural or room temperature aging strengths after a few days. These can be stocked for fabrication in the –T4 or –T3 tempers. High zinc content alloys, such as 7075, continue to age appreciably over a long period of time. Their mechanical property changes being sufficient to reduce their formability. The advantage of –W temper formability can be utilized; however, in the same manner as with natural- aging alloys; that is, by fabricating shortly after solution heat treatment or retaining formability by using refrigeration. Refrigeration retards the rate of natural aging. At 32 °F, the beginning of the aging process is delayed for several hours, while dry ice (−50 °F to −100 °F) retards aging for an extended period of time. Precipitation Practices The temperatures used for precipitation hardening depend upon the alloy and the properties desired, ranging from 250 °F to 375 °F. They should be controlled within a very narrow range (±5 °F) to obtain best results. [Figure] Conditions for heat treatment of aluminum alloys The time at temperature is dependent upon the temperature used, the properties desired, and the alloy. It ranges from 8 to 96 hours. Increasing the aging temperature decreases the soaking period necessary for proper aging. However, a closer control of both time and temperature is necessary when using the higher temperatures. After receiving the thermal precipitation treatment, the material should be air cooled to room temperature. Water quenching, while not necessary, produces no ill effects. Furnace cooling tends to produce over-aging. ··· Annealing of Aluminum Alloys The annealing procedure for aluminum alloys consists of heating the alloys to an elevated temperature, holding or soaking them at this temperature for a length of time depending upon the mass of the metal, and then cooling in still air. Annealing leaves the metal in the best condition for cold working. However, when prolonged forming operations are involved, the metal takes on a condition known as “mechanical hardness” and resists further working. It may be necessary to anneal a part several times during the forming process to avoid cracking. Aluminum alloys should not be used in the annealed state for parts or fittings. Clad parts should be heated as quickly and carefully as possible, since long exposure to heat tends to cause some of the constituents of the core to diffuse into the cladding. This reduces the corrosion resistance of the cladding. RELATED POSTS Properties of Metals Ferrous Aircraft Metals Nonferrous Aircraft Metals Metalworking Processes Heat Treatment of Ferrous Metals Heat Treatment of Nonferrous Metals Heat Treatment of Aluminum Alloy Rivets, Magnesium Alloys and Titanium ··· Tags Aircraft Materials Share Previous Post Next Post Sponsored Content Recommended by Upgrade to Office 2024 (Get Now) Discover the Cutest Animal in the World One-time purchase. No subscription. Now! ps.sbs Cutest Animal Sarkari Media - Status & Latest Govt Exam Results Contact | Privacy | Terms | Advertise Copyright @ 2024 Aeronautics-Guide --> Home Aircraft Materials SEARCH Heat Treatment of Nonferrous Metals Search × FLYING TRAINING Airplane Flying Helicopter Flying Instrument Flying Aeronautical Knowledge Aviation Instructor Insrtument Procedures Aluminum Alloys In the wrought form, commercially-pure aluminum is known as 1100. It has a high degree of resistance to corrosion and is easily formed into intricate shapes. It is relatively low in strength and does not have the properties required for structural aircraft parts. The process of alloying generally obtains high strengths. The resulting alloys are less easily formed and, with some exceptions, have lower resistance to corrosion ··· than 1100 aluminum. Alloying is not the only method of increasing the strength of aluminum. Like other materials, aluminum becomes stronger and harder as it is rolled, formed, or otherwise cold worked. Since the hardness depends on the amount of cold working done, 1100 and some wrought aluminum alloys are available in several strain-hardened tempers. The soft or annealed condition is designated O. If the material is strain hardened, it is said to be in the H condition. The most widely used alloys in aircraft construction are hardened by heat treatment rather than by cold work. These alloys are designated by a somewhat different set of symbols: T4 and W indicate solution heat treated and quenched but not aged, and T6 indicates an alloy in the heat-treated, hardened condition. W—solution heat treated, unstable temper T—treated to produce stable tempers other than F, O, or H T2—annealed (cast products only) T3—solution heat treated and then cold worked T4—solution heat treated T5—artificially aged only T6—solution heat treated and then artificially aged T7—solution heat treated and then stabilized T8—solution heat treated, cold worked, and then artificially aged T9—solution heat treated, artificially aged, and then cold worked T10—artificially aged and then cold worked Additional digits may be added to T1 through T10 to indicate a variation in treatment, which significantly alters the characteristics of the product. Aluminum-alloy sheets are marked with the specification number on approximately every square foot of material. If for any reason this identification is not on the material, it is possible to separate the heat- treatable alloys from the non-heat-treatable alloys by immersing a sample of the material in a 10 percent solution of caustic soda (sodium hydroxide). The heat-treatable alloys turn black due to the copper content, whereas the others remain bright. In the case of clad material, the surface remains bright, but there is a dark area in the middle when viewed from the edge. ··· Alclad Aluminum The terms “Alclad and Pureclad” are used to designate sheets that consist of an aluminum-alloy core coated with a layer of pure aluminum to a depth of approximately 51⁄2 percent on each side. The pure aluminum coating affords a dual protection for the core, preventing contact with any corrosive agents, and protecting the core electrolytically by preventing any attack caused by scratching or from other abrasions. There are two types of heat treatments applicable to aluminum alloys: solution heat treatment and precipitation heat treatment. Some alloys, such as 2017 and 2024, develop their full properties as a result of solution heat treatment followed by about 4 days of aging at room temperature. Other alloys, such as 2014 and 7075, require both heat treatments. The alloys that require precipitation heat treatment (artificial aging) to develop their full strength also age to a limited extent at room temperature; the rate and amount of strengthening depends upon the alloy. Some reach their maximum natural or room temperature aging strength in a few days, and are designated as –T4 or –T3 temper. Others continue to age appreciably over a long period of time. Because of this natural aging, the –W designation is specified only when the period of aging is indicated, for example, 7075–W (1⁄2 hour). Thus, there is considerable difference in the mechanical and physical properties of freshly quenched (–W) material and material that is in the –T3 or –T4 temper. The hardening of an aluminum alloy by heat treatment consists of four distinct steps: 1. Heating to a predetermined temperature. 2. Soaking at temperature for a specified length of time. 3. Rapidly quenching to a relatively low temperature. 4. Aging or precipitation hardening either spontaneously at room temperature, or because of a low temperature thermal treatment. The first three steps above are known as solution heat treatment, although it has become common practice to use the shorter term, “heat treatment.” Room temperature hardening is known as natural aging, while hardening done at moderate temperatures is called artificial aging, or precipitation heat treatment. ··· Solution Heat Treatment Temperature The temperatures used for solution heat treating vary with different alloys and range from 825 °F to 980 °F. As a rule, they must be controlled within a very narrow range (±10 °F) to obtain specified properties. If the temperature is too low, maximum strength is not obtained. When excessive temperatures are used, there is danger of melting the low melting constituents of some alloys with consequent lowering of the physical properties of the alloy. Even if melting does not occur, the use of higher than recommended temperatures promotes discoloration and increases quenching strains. Time at Temperature The time at temperature, referred to as soaking time, is measured from the time the coldest metal reaches the minimum limit of the desired temperature range. The soaking time varies, depending upon the alloy and thickness, from 10 minutes for thin sheets to approximately 12 hours for heavy forgings. For the heavy sections, the nominal soaking time is approximately 1 hour for each inch of cross-sectional thickness. [Figure] Typical soaking times for heat treatment Choose the minimum soaking time necessary to develop the required physical properties. The effect of an abbreviated soaking time is obvious. An excessive soaking period aggravates high-temperature oxidation. With clad material, prolonged heating results in excessive diffusion of copper and other soluble constituents into the protective cladding and may defeat the purpose of cladding. Quenching After the soluble constituents are in solid solution, the material is quenched to prevent or retard immediate re-precipitation. Three distinct quenching methods are employed. The one to be used in any instance depends upon the part, the alloy, and the properties desired. ··· Cold Water Quenching Parts produced from sheet, extrusions, tubing, small forgings, and similar type material are generally quenched in a cold-water bath. The temperature of the water before quenching should not exceed 85 °F. Using a sufficient quantity of water keeps the temperature rise under 20 °F. Such a drastic quench ensures maximum resistance to corrosion. This is particularly important when working with alloys, such as 2017, 2024, and 7075. This is the reason a drastic quench is preferred, even though a slower quench may produce the required mechanical properties. Hot Water Quenching Large forgings and heavy sections can be quenched in hot or boiling water. This type of quench minimizes distortion and alleviates cracking, which may be produced by the unequal temperatures obtained during the quench. The use of a hot water quench is permitted with these parts, because the temperature of the quench water does not critically affect the resistance to corrosion of the forging alloys. In addition, the resistance to corrosion of heavy sections is not as critical a factor as for thin sections. Spray Quenching High-velocity water sprays are useful for parts formed from clad sheet and for large sections of almost all alloys. This type of quench also minimizes distortion and alleviates quench cracking. However, many specifications forbid the use of spray quenching for bare 2017 and 2024 sheet materials because of the effect on their resistance to corrosion. ··· Lag Between Soaking and Quenching The time interval between the removal of the material from the furnace and quenching is critical for some alloys and should be held to a minimum. The elapsed time must not exceed 10 seconds when solution heat treating 2017 or 2024 sheet material. The allowable time for heavy sections may be slightly greater. Allowing the metal to cool slightly before quenching promotes re-precipitation from the solid solution. The precipitation occurs along grain boundaries and in certain slip planes causing poorer formability. In the case of 2017, 2024, and 7075 alloys, their resistance to intergranular corrosion is adversely affected. Reheat Treatment The treatment of material, which has been previously heat-treated, is considered a re-heat treatment. The unclad heat-treatable alloys can be solution heat treated repeatedly without harmful effects. The number of solution heat treatments allowed for clad sheet is limited due to increased diffusion of core and cladding with each reheating. Existing specifications allow one to three re-heat treatments of clad sheet depending upon cladding thickness. RELATED POSTS Properties of Metals Ferrous Aircraft Metals Nonferrous Aircraft Metals Metalworking Processes Heat Treatment of Ferrous Metals Precipitation Heat Treating and Annealing of Aluminum Alloys Heat Treatment of Aluminum Alloy Rivets, Magnesium Alloys and Titanium ··· Tags Aircraft Materials Share Previous Post Next Post Sponsored ContentRecommended by Upgrade to Office 2024 (Get Now) ps.sbs Explore Discoveryfeed Now DiscoveryFeed Contact | Privacy | Terms | Advertise Copyright @ 2024 Aeronautics-Guide --> Home Aircraft Materials SEARCH Nonmetallic Aircraft Materials Search FLYING TRAINING Airplane Flying Helicopter Flying Instrument Flying Aeronautical Knowledge Aviation Instructor Insrtument Procedures The use of magnesium, plastic, fabric, and wood in aircraft construction has nearly disappeared since the mid-1950s. Aluminum has also greatly diminished in use, from 80 percent of airframes in 1950 to about 15 ··· percent aluminum and aluminum alloys today for airframe construction. Replacing those materials are nonmetallic aircraft materials, such as reinforced plastics and advanced composites. Wood The earliest aircraft were constructed of wood and cloth. Today, except for restorations and some home- built aircraft, very little wood is used in aircraft construction. Plastics Plastics are used in many applications throughout modern aircraft. These applications range from structural components of thermosetting plastics reinforced with fiberglass to decorative trim of thermoplastic materials to windows. ··· Transparent Plastics Transparent plastic materials used in aircraft canopies, such as windshields, windows and other similar transparent enclosures, may be divided into two major classes or groups: thermoplastic and thermosetting. These plastics are classified according to their reaction to heat. Thermoplastic materials soften when heated and harden when cooled. These materials can be heated until soft and then formed into the desired shape. When cooled, they retain this shape. The same piece of plastic can be reheated and reshaped any number of times without changing the chemical composition of the materials. Thermosetting plastics harden upon heating, and reheating has no softening effect. These plastics cannot be reshaped once being fully cured by the application of heat. In addition to the above classes, transparent plastics are manufactured in two forms: monolithic (solid) and laminated. Laminated transparent plastics are made from transparent plastic face sheets bonded by an inner layer material, usually polyvinyl butyryl. Because of its shatter resistant qualities, laminated plastic is superior to solid plastics and is used in many pressurized aircraft. Most of the transparent sheet used in aviation is manufactured in accordance with various military specifications. A new development in transparent plastics is stretched acrylic. Stretched acrylic is a type of plastic, which before being shaped, is pulled in both directions to rearrange its molecular structure. Stretched acrylic panels have a greater resistance to impact and are less subject to shatter; its chemical resistance is greater, edging is simpler, and crazing and scratches are less detrimental. Individual sheets of plastic are covered with a heavy masking paper to which a pressure sensitive adhesive has been added. This paper helps to prevent accidental scratching during storage and handling. Be careful to avoid scratches and gouges which may be caused by sliding sheets against one another or across rough or dirty tables. If possible, store sheets in bins that are tilted at approximately 10° from vertical. If they must be stored horizontally, piles should not be over 18 inches high, and small sheets should be stacked on the larger ones to avoid unsupported overhang. Store in a cool, dry place away from solvent fumes, heating coils, radiators, and steam pipes. The temperature in the storage room should not exceed 120 °F. While direct sunlight does not harm acrylic plastic, it causes drying and hardening of the masking adhesive, making removal of the paper difficult. If the paper does not roll off easily, place the sheet in an oven at 250 °F for 1 minute, maximum. The heat softens the masking adhesive for easy removal of the paper. If an oven is not available, remove hardened masking paper by softening the adhesive with aliphatic naphtha. Rub the masking paper with a cloth saturated with naphtha. This softens the adhesive and frees the paper from the plastic. Sheets so treated must be washed immediately with clean water, taking care not to scratch the surfaces. Note: Aliphatic naphtha is not to be confused with aromatic naphtha and other dry cleaning solvents, which have harmful effects on plastic. However, aliphatic naphtha is flammable and all precautions regarding the use of flammable liquids must be observed. × Composite Materials In the 1940s, the aircraft industry began to develop synthetic fibers to enhance aircraft design. Since that time, composite materials have been used more and more. When composites are mentioned, most people think of only fiberglass, or maybe graphite or aramids (Kevlar). Composites began in aviation, but now are being embraced by many other industries, including auto racing, sporting goods, and boating, as well as defense industry uses. A “composite” material is defined as a mixture of different materials or things. This definition is so general that it could refer to metal alloys made from several different metals to enhance the strength, ductility, conductivity, or whatever characteristics are desired. Likewise, the composition of composite materials is a combination of reinforcement, such as a fiber, whisker, or particle, surrounded and held in place by a resin forming a structure. Separately, the reinforcement and the resin are very different from their combined state. Even in their combined state, they can still be individually identified and mechanically separated. One composite, concrete, is composed of cement (resin) and gravel or reinforcement rods for the reinforcement to create the concrete. Advantages/Disadvantages of Composites Some of the many advantages for using composite materials are: High strength-to-weight ratio Fiber-to-fiber transfer of stress allowed by chemical bonding Modulus (stiffness-to-density ratio) 3.5 to 5 times that of steel or aluminum Longer life than metals Higher corrosion resistance Tensile strength 4 to 6 times that of steel or aluminum Greater design flexibility Bonded construction eliminates joints and fasteners Easily repairable The disadvantages of composites include: Inspection methods difficult to conduct, especially delamination detection (Advancements in technology will eventually correct this problem.) Lack of long-term design database, relatively new technology methods Cost Very expensive processing equipment Lack of standardized system of methodology Great variety of materials, processes, and techniques General lack of repair knowledge and expertise Products often toxic and hazardous Lack of standardized methodology for construction and repairs The increased strength and the ability to design for the performance needs of the product makes composites much superior to the traditional materials used in today’s aircraft. As more and more composites are used, the costs, design, inspection ease, and information about strength-to-weight advantages help composites become the material of choice for aircraft construction. × × Composite Safety Composite products can be very harmful to the skin, eyes, and lungs. In the long or short term, people can become sensitized to the materials with serious irritation and health issues. Personal protection is often uncomfortable, hot, and difficult to wear; however, a little discomfort while working with the composite materials can prevent serious health issues or even death. Respirator particle protection is very important to protecting the lungs from permanent damage from tiny glass bubbles and fiber pieces. At a minimum, a dust mask approved for fiberglass is a necessity. The best protection is a respirator with dust filters. The proper fit of a respirator or dust mask is very important, because if the air around the seal is breathed, the mask cannot protect the wearer’s lungs. When working with resins, it is important to use vapor protection. Charcoal filters in a respirator remove the vapors for a period of time. When removing the respirator for breaks, and upon placing the mask back on, if you can smell the resin vapors, replace the filters immediately. Sometimes, charcoal filters last less than 4 hours. Store the respirator in a sealed bag when not in use. If working with toxic materials for an extended period, a supplied air mask and hood are recommended. Avoid skin contact with the fibers and other particles by wearing long pants and long sleeves along with gloves or barrier creams. The eyes must be protected using leak-proof goggles (no vent holes) when working with resins or solvents, because chemical damage to the eyes is usually irreversible. Fiber Reinforced Materials The purpose of reinforcement in reinforced plastics is to provide most of the strength. The three main forms of fiber reinforcements are particles, whiskers, and fibers. A particle is a square piece of material. Glass bubbles (Q-cell) are hollow glass spheres, and since their dimensions are equal on all axes, they are called a particle. A whisker is a piece of material that is longer than it is wide. Whiskers are usually single crystals. They are very strong and used to reinforce ceramics and metals. Fibers are single filaments that are much longer than they are wide. Fibers can be made of almost any material and are not crystalline like whiskers. Fibers are the base for most composites. Fibers are smaller than the finest human hair and are normally woven into cloth-like materials. ··· Laminated Structures Composites can be made with or without an inner core of material. Laminated structure with a core center is called a sandwich structure. Laminate construction is strong and stiff, but heavy. The sandwich laminate is equal in strength, and its weight is much less; less weight is very important to aerospace products. The core of a laminate can be made from nearly anything. The decision is normally based on use, strength, and fabricating methods to be used. Various types of cores for laminated structures include rigid foam, wood, metal, or the aerospace preference of honeycomb made from paper, Nomex, carbon, fiberglass, or metal. Figure 1 shows a typical sandwich structure. Figure 1. Sandwich structure It is very important to follow proper techniques to construct or repair laminated structures to ensure the strength is not compromised. Taking a high-density laminate or solid face and back plate and sandwiching a core in the middle make a sandwich assembly. The design engineer, depending on the intended application of the part, decides the selection of materials for the face and the back plate. It is important to follow manufacturers’ maintenance manual specific instructions regarding testing and repair procedures as they apply to a particular aircraft. ··· Reinforced Plastic Reinforced plastic is a thermosetting material used in the manufacture of radomes, antenna covers, and wingtips, and as insulation for various pieces of electrical equipment and fuel cells. It has excellent dielectric characteristics that make it ideal for radomes; however, its high strength-to-weight ratio, resistance to mildew, rust, and rot, and ease of fabrication make it equally suited for other parts of the aircraft. Reinforced plastic components of aircraft are formed of either solid laminates or sandwich-type laminates. Resins used to impregnate glass cloths are of the contact pressure type (requiring little or no pressure during cure). These resins are supplied as a liquid, which can vary in viscosity from water like consistency to thick syrup. Cure or polymerization is affected by the use of a catalyst, usually benzoyl peroxide. Solid laminates are constructed of three or more layers of resin impregnated cloths “wet laminated” together to form a solid sheet facing or molded shape. Sandwich-type laminates are constructed of two or more solid sheet facings or a molded shape enclosing a fiberglass honeycomb or foam-type core. Honeycomb cores are made of glass cloths impregnated with polyester or a combination of nylon and phenolic resins. The specific density and cell size of honeycomb cores varies over considerable latitude. Honeycomb cores are normally fabricated in blocks that are later cut to the desired thickness on a band saw. Foam-type cores are formulated from combinations of alkyd resins and metatoluene di-isocyanate. Sandwich-type fiberglass components filled with foam-type cores are manufactured to exceedingly close tolerances on overall thickness of the molded facing and core material. To achieve this accuracy, the resin is poured into a close tolerance, molded shape. The resin formulation immediately foams up to fill the void in the molded shape and forms a bond between the facing and the core. Rubber Rubber is used to prevent the entrance of dirt, water, or air, and to prevent the loss of fluids, gases, or air. It is also used to absorb vibration, reduce noise, and cushion impact loads. The term “rubber” is as all- inclusive as the term “metal.” It is used to include not only natural rubber, but also all synthetic and silicone rubbers. ··· Natural Rubber Natural rubber has better processing and physical properties than synthetic or silicone rubber. These properties include flexibility, elasticity, tensile strength, tear strength, and low heat buildup due to flexing (hysteresis). Natural rubber is a general-purpose product; however, its suitability for aircraft use is somewhat limited because of its inferior resistance to most influences that cause deterioration. Although it provides an excellent seal for many applications, it swells and often softens in all aircraft fuels and in many solvents (naphthas and so forth). Natural rubber deteriorates more rapidly than synthetic rubber. It is used as a sealing material for water/methanol systems. Synthetic Rubber Synthetic rubber is available in several types, each of which is compounded of different materials to give the desired properties. The most widely used are the butyls, Bunas, and neoprene. Butyl is a hydrocarbon rubber with superior resistance to gas permeation. It is also resistant to deterioration; however, its comparative physical properties are significantly less than those of natural rubber. Butyl resists oxygen, vegetable oils, animal fats, alkalies, ozone, and weathering. Like natural rubber, butyl swells in petroleum or coal tar solvents. It has a low water absorption rate and good resistance to heat and low temperature. Depending on the grade, it is suitable for use in temperatures ranging from −65 °F to 300 °F. Butyl is used with phosphate ester hydraulic fluids (Skydrol™), silicone fluids, gases, ketones, and acetones. Buna-S rubber resembles natural rubber both in processing and performance characteristics. Buna-S is as water resistant as natural rubber, but has somewhat better aging characteristics. It has good resistance to heat, but only in the absence of severe flexing. Generally, Buna-S has poor resistance to gasoline, oil, concentrated acids, and solvents. Buna-S is normally used for tires and tubes as a substitute for natural rubber. Buna-N is outstanding in its resistance to hydrocarbons and other solvents; however, it has poor resilience in solvents at low temperature. Buna-N compounds have good resistance to temperatures up to 300 °F and may be procured for low temperature applications down to −75 °F. Buna-N has fair tear, sunlight, and ozone resistance. It has good abrasion resistance and good breakaway properties when used in contact with metal. When used as a seal on a hydraulic piston, it does not stick to the cylinder wall. Buna-N is used for oil and gasoline hoses, tank linings, gaskets, and seals. Neoprene can take more punishment than natural rubber and has better low-temperature characteristics. It possesses exceptional resistance to ozone, sunlight, heat, and aging. Neoprene looks and feels like rubber. Neoprene, however, is less like rubber in some of its characteristics than butyl or Buna. The physical characteristics of neoprene, such as tensile strength and elongation, are not equal to natural rubber but do have a definite similarity. Its tear resistance, as well as its abrasion resistance, is slightly less than that of natural rubber. Although its distortion recovery is complete, it is not as rapid as natural rubber. Neoprene has superior resistance to oil. Although it is good material for use in nonaromatic gasoline systems, it has poor resistance to aromatic gasoline. Neoprene is used primarily for weather seals, window channels, bumper pads, oil resistant hose, and carburetor diaphragms. It is also recommended for use with Freon™ and silicate ester lubricants. Thiokol, known also as polysulfide rubber, has the highest resistance to deterioration but ranks the lowest in physical properties. Petroleum, hydrocarbons, esters, alcohols, gasoline, or water, in general, does not seriously affect Thiokols. Thiokols are ranked low in such physical properties as compression set, tensile strength, elasticity, and tear abrasion resistance. Thiokol is used for oil hoses, tank linings for aromatic aviation gasoline, gaskets, and seals. Silicone rubbers are a group of plastic rubber materials made from silicon, oxygen, hydrogen, and carbon. The silicones have excellent heat stability and very low temperature flexibility. They are suitable for gaskets, seals, or other applications where elevated temperatures up to 600 °F are prevalent. Silicone rubbers are also resistant to temperatures down to −150 °F. Throughout this temperature range, silicone rubber remains extremely flexible and useful with no hardness or gumminess. Although this material has good resistance to oils, it reacts unfavorably to both aromatic and nonaromatic gasoline. Silastic, one of the best-known silicones, is used to insulate electrical and electronic equipment. Because of its dielectric properties over a wide range of temperatures, it remains flexible and free from crazing and cracking. Silastic is also used for gaskets and seals in certain oil systems. ··· Shock Absorber Cord Shock absorber cord is made from natural rubber strands encased in a braided cover of woven cotton cords treated to resist oxidation and wear. Great tension and elongation are obtained by weaving the jacket upon the bundle of rubber strands while they are stretched about three times their original length. There are two types of elastic shock absorbing cord. Type I is a straight cord, and type II is a continuous ring known as a “bungee.” The advantages of the type II cord are that it is easily and quickly replaced and does not need to be secured by stretching and whipping. Shock cord is available in standard diameters from 1⁄4 inch to 13⁄16 inch. Three colored threads are braided into the outer cover for the entire length of the cord. Two of these threads are of the same color and represent the year of manufacture; the third thread, a different color, represents the quarter of the year in which the cord was made. The code covers a 5-year period and then repeats itself. This makes it easy to figure forward or backward from the years shown in Figure 2. Figure 2. Shock absorber cord color coding RELATED POSTS Aircraft Materials, Hardware, and Processes Properties of Metals Ferrous Aircraft Metals Nonferrous Aircraft Metals Heat Treatment of Nonferrous Metals Precipitation Heat Treating and Annealing of Aluminum Alloys Heat Treatment of Aluminum Alloy Rivets, Magnesium Alloys and Titanium Hardness Testing ··· Tags Aircraft Materials Share Previous Post Next Post Sponsored Content Recommended by Upgrade to Office 2024 (Get Now) Looking for More Content? We May Have One-time purchase. No subscription. What You Want ps.sbs DiscoveryFeed Contact | Privacy | Terms | Advertise Copyright @ 2024 Aeronautics-Guide --> Home Aircraft Materials SEARCH Search FLYING TRAINING Airplane Flying Heat Treatment of Aluminum Alloy Rivets, Magnesium Helicopter Flying Instrument Flying Alloys and Titanium Aeronautical Knowledge × Aviation Instructor Insrtument Procedures Heat Treatment of Aluminum Alloy Rivets Aluminum alloy rivets are furnished in the following compositions: alloys 1100, 5056, 2117, 2017, and 2024. Alloy 1100 rivets are used in the “as fabricated” condition for riveting aluminum alloy sheets where a low- ··· strength rivet is suitable. Alloy 5056 rivets are used in the “as fabricated” condition for riveting magnesium alloy sheets. Alloy 2117 rivets have moderately high strength and are suitable for riveting aluminum alloy sheets. These rivets receive only one heat treatment, which is performed by the manufacturer, and are anodized after being heat treated. They require no further heat treatment before they are used. Alloy 2117 rivets retain their characteristics indefinitely after heat treatment and can be driven anytime. Rivets made of this alloy are the most widely used in aircraft construction. Alloy 2017 and 2024 rivets are high-strength rivets suitable for use with aluminum alloy structures. They are purchased from the manufacturer in the heat-treated condition. Since the aging characteristics of these alloys at room temperatures are such that the rivets are unfit for driving, they must be reheat- treated just before they are to be used. Alloy 2017 rivets become too hard for driving in approximately 1 hour after quenching. Alloy 2024 rivets become hardened in 10 minutes after quenching. Both alloys may be reheat-treated as often as required; however, they must be anodized before the first reheat treatment to prevent intergranular oxidation of the material. If these rivets are stored in a refrigerator at a temperature lower than 32 °F immediately after quenching, they remain soft enough to be usable for several days. × Rivets requiring heat treatment are heated either in tubular containers in a salt bath or in small screen wire baskets in an air furnace. The heat treatment of alloy 2017 rivets consists of subjecting the rivets to a temperature between 930 °F to 950 °F for approximately 30 minutes and immediately quenching in cold water. These rivets reach maximum strength in about 9 days after being driven. Alloy 2024 rivets should be heated to a temperature of 910 °F to 930 °F and immediately quenched in cold water. These rivets develop greater shear strength than 2017 rivets and are used in locations where extra strength is required. Alloy 2024 rivets develop their maximum shear strength in 1 day after being driven. The 2017 rivet should be driven within approximately 1 hour and the 2024 rivet within 10 to 20 minutes after heat treating or removal from refrigeration. If not used within these times, the rivets should be reheat treated before being refrigerated. Heat Treatment of Magnesium Alloys Magnesium alloy castings respond readily to heat treatment, and about 95 percent of the magnesium used in aircraft construction is in the cast form. The heat treatment of magnesium alloy castings is like the heat treatment of aluminum alloys in that there are two types of heat treatment: solution heat treatment and precipitation (aging) heat treatment. Magnesium, however, develops a negligible change in its properties when allowed to age naturally at room temperatures. Solution Heat Treatment Magnesium alloy castings are solution heat treated to improve tensile strength, ductility, and shock resistance. This heat-treatment condition is indicated by using the symbol –T4 following the alloy designation. Solution heat treatment plus artificial aging is designated –T6. Artificial aging is necessary to develop the full properties of the metal. Solution heat-treatment temperatures for magnesium alloy castings range from 730 °F to 780 °F, the exact range depending upon the type of alloy. The temperature range for each type of alloy is listed in Specification MIL-H-6857. The upper limit of each range listed in the specification is the maximum temperature to which the alloy may be heated without danger of melting the metal. The soaking time ranges from 10 to 18 hours, the exact time depending upon the type of alloy as well as the thickness of the part. Soaking periods longer than 18 hours may be necessary for castings over 2 inches in thickness. NEVER heat magnesium alloys in a salt bath as this may result in an explosion. A serious potential fire hazard exists in the heat treatment of magnesium alloys. If through oversight or malfunctioning of equipment the maximum temperatures are exceeded, the casting may ignite and burn freely. For this reason, the furnace used should be equipped with a safety cutoff that turns off the power to the heating elements and blowers if the regular control equipment malfunctions or fails. Some magnesium alloys require a protective atmosphere of sulfur dioxide gas during solution heat treatment. This aids in preventing the start of a fire even if the temperature limits are slightly exceeded. Air quenching is used after solution heat treatment of magnesium alloys since there appears to be no advantage in liquid cooling. ··· Precipitation Heat Treatment After solution treatment, magnesium alloys may be given an aging treatment to increase hardness and yield strength. Generally, the aging treatments are used merely to relieve stress and stabilize the alloys to prevent dimensional changes later, especially during or after machining. Both yield strength and hardness are improved somewhat by this treatment at the expense of a slight amount of ductility. The corrosion resistance is also improved, making it closer to the “as cast” alloy. Precipitation heat-treatment temperatures are considerably lower than solution heat-treatment temperatures and range from 325 °F to 500 °F. Soaking time ranges from 4 to 18 hours. Heat Treatment of Titanium Titanium is heat treated for the following purposes: Relief of stresses set up during cold forming or machining. Annealing after hot working or cold working, or to provide maximum ductility for subsequent cold working. Thermal hardening to improve strength. Stress Relieving Stress relieving is generally used to remove stress concentrations resulting from forming of titanium sheet. It is performed at temperatures ranging from 650 °F to 1,000 °F. The time at temperature varies from a few minutes for a very thin sheet to an hour or more for heavier sections. A typical stress relieving treatment is 900 °F for 30 minutes, followed by an air cool. The discoloration or scale that forms on the surface of the metal during stress relieving is easily removed by pickling in acid solutions. The recommended solution contains 10 to 20 percent nitric acid and 1 to 3 percent hydrofluoric acid. The solution should be at room temperature or slightly above. Full Annealing The annealing of titanium and titanium alloys provides toughness, ductility at room temperature, dimensional and structural stability at elevated temperatures, and improved machinability. The full anneal is usually called for as preparation for further working. It is performed at 1,200 to 1,650 °F. The time at temperature varies from 16 minutes to several hours, depending on the thickness of the material and the amount of cold work to be performed. The usual treatment for the commonly used alloys is 1,300 °F for 1 hour, followed by an air cool. A full anneal generally results in sufficient scale formation to require the use of caustic descaling, such as sodium hydride salt bath. ··· Thermal Hardening Unalloyed titanium cannot be heat treated, but the alloys commonly used in aircraft construction can be strengthened by thermal treatment, usually at some sacrifice in ductility. For best results, a water quench from 1,450 °F, followed by reheating to 900 °F for 8 hours is recommended. Casehardening The chemical activity of titanium and its rapid absorption of oxygen, nitrogen, and carbon at relatively low temperatures make casehardening advantageous for special applications. Nitriding, carburizing, or carbonitriding can be used to produce a wear-resistant case of 0.0001 to 0.0002 inch in depth. RELATED POSTS Properties of Metals Ferrous Aircraft Metals Nonferrous Aircraft Metals Metalworking Processes Heat Treatment of Ferrous Metals Heat Treatment of Nonferrous Metals Precipitation Heat Treating and Annealing of Aluminum Alloys ··· Tags Aircraft Materials Share Previous Post Next Post Sponsored Content Recommended by Upgrade to Office 2024 (Get Now) ps.sbs Check Out Our Recommendations Curated Just for You DiscoveryFeed Contact | Privacy | Terms | Advertise Copyright @ 2024 Aeronautics-Guide --> HOME AIRFRAME POWERPLANT GENERAL MAINTENANCE QUESTION BANK AVIATION GLOSSARY HANDBOOKS SPONSORSHIP Home Aircraft Materials SEARCH Heat Treatment of Ferrous Metals Search FLYING TRAINING Airplane Flying Helicopter Flying Instrument Flying The first important consideration in the heat treatment of a steel part is to know its chemical composition. Aeronautical Knowledge This, in turn, determines its upper critical point. When the upper critical point is known, the next Aviation Instructor consideration is the rate of heating and cooling to be used. Carrying out these operations involves the use of uniform heating furnaces, proper temperature controls, and suitable quenching mediums. Insrtument Procedures Behavior of Steel During Heating and Cooling Changing the internal structure of a ferrous metal is accomplished by heating to a temperature above its upper critical point, holding it at that temperature for a time sufficient to permit certain internal changes ··· to occur, and then cooling to atmospheric temperature under predetermined, controlled conditions. At ordinary temperatures, the carbon in steel exists in the form of particles of iron carbide scattered throughout an iron matrix known as “ferrite.” The number, size, and distribution of these particles determine the hardness of the steel. At elevated temperatures, the carbon is dissolved in the iron matrix in the form of a solid solution called “austenite,” and the carbide particles appear only after the steel has been cooled. If the cooling is slow, the carbide particles are relatively coarse and few. In this condition, the steel is soft. If the cooling is rapid, as by quenching in oil or water, the carbon precipitates as a cloud of very fine carbide particles, and the steel is hard. The fact that the carbide particles can be dissolved in austenite is the basis of the heat treatment of steel. The temperatures at which this transformation takes place are called the critical points and vary with the composition of the steel. The percent of carbon in the steel has the greatest influence on the critical points of heat treatment. ··· Hardening Pure iron, wrought iron, and extremely low carbon steels cannot be appreciably hardened by heat treatment, since they contain no hardening element. Cast iron can be hardened, but its heat treatment is limited. When cast iron is cooled rapidly, it forms white iron, which is hard and brittle. When cooled slowly, it forms gray iron, which is soft but brittle under impact. In plain carbon steel, the maximum hardness depends almost entirely on the carbon content of the steel. As carbon content increases, the ability of steel to harden also increases. However, this increase in the ability to harden with an increase in carbon content continues only to a certain point. In practice, that point is 0.85 percent carbon content. When the carbon content is increased beyond 0.85 percent, there is no increase in wear resistance. ··· For most steels, the hardening treatment consists of heating the steel to a temperature just above the upper critical point, soaking or holding for the required length of time, and then cooling it rapidly by plunging the hot steel into oil, water, or brine. Although most steels must be cooled rapidly for hardening, a few may be cooled in still air. Hardening increases the hardness and strength of the steel but makes it less ductile. Carbon steel must be cooled to below 1,000 °F in less than 1 second when hardening. Should the time required for the temperature to drop to 1,000 °F exceed 1 second, the austenite begins to transform into fine pearlite. This pearlite varies in hardness, but is much harder than the pearlite formed by annealing and much softer than the martensite desired. After the 1,000 °F temperature is reached, the rapid cooling must continue if the final structure is to be all martensite. The time limit for the temperature drop to 1,000 °F increases above the 1 second limit for carbon steels when alloys are added to steel. Therefore, a slower quenching medium produces hardness in alloy steels. Because of the high internal stresses in the “as quenched” condition, steel must be tempered just before it becomes cold. The part should be removed from the quenching bath at a temperature of approximately 200 °F, since the temperature range from 200 °F down to room temperature is the cracking range. ··· Hardening temperatures and quenching mediums for the various types of steel are listed in Figure. Heat treatment procedures for steels Hardening Precautions A variety of different shapes and sizes of tongs for handling hot steels is necessary. It should be remembered that cooling of the area contacted by the tongs is retarded and that such areas may not harden, particularly if the steel being treated is very shallow hardening. Small parts may be wired together or quenched in baskets made of wire mesh. Special quenching jigs and fixtures are frequently used to hold steels during quenching in a manner to restrain distortion. When selective hardening is desired, covering with alundum cement or some other insulating material may protect portions of the steel. Selective hardening may be accomplished by using water or oil jets designed to direct quenching medium on the areas to be hardened. This also is accomplished by the induction and flame hardening procedures previously described, particularly on large production jobs. Shallow hardening steels, such as plain carbon and certain varieties of alloy steels, have such a high critical cooling rate that they must be quenched in brine or water to effect hardening. In general, intricately-shaped sections should not be made of shallow hardening steels because of the tendency of these steels to warp and crack during hardening. Such items should be made of deeper hardening steels capable of being hardened by quenching in oil or air. ··· Tempering Tempering reduces the brittleness imparted by hardening and produces definite physical properties within the steel. Tempering always follows, never precedes, the hardening operation. In addition to reducing brittleness, tempering softens the steel. Tempering is always conducted at temperatures below the low critical point of the steel. In this respect, tempering differs from annealing, normalizing, or hardening, all of which require temperatures above the upper critical point. When hardened steel is reheated, tempering begins at 212 °F and continues as the temperature increases toward the low critical point. By selecting a definite tempering temperature, the resulting hardness and strength can be predetermined. Approximate temperatures for various tensile strengths are listed in Figure. The minimum time at the tempering temperature should be 1 hour. If the part is over one inch in thickness, increase the time by 1 hour for each additional inch of thickness. Tempered steels used in aircraft work have from 125,000 to 200,000 psi ultimate tensile strength. Generally, the rate of cooling from the tempering temperature has no effect on the resulting structure; therefore, the steel is usually cooled in still air after being removed from the furnace. Annealing Annealing of steel produces a fine-grained, soft, ductile metal without internal stresses or strains. In the annealed state, steel has its lowest strength. In general, annealing is the opposite of hardening. Heating the metal to just above the upper critical point, soaking at that temperature, and cooling very slowly in the furnace accomplishes annealing of steel. (Refer to Figure for recommended temperatures.) Soaking time is approximately 1 hour per inch of thickness of the material. To produce maximum softness in steel, the metal must be cooled very slowly. Slow cooling is obtained by shutting off the heat and allowing the furnace and metal to cool together to 900 °F or lower, then removing the metal from the furnace and cooling in still air. Another method is to bury the heated steel in ashes, sand, or other substance that does not conduct heat readily. Normalizing The normalizing of steel removes the internal stresses set up by heat treating, welding, casting, forming, or machining. Stress, if not controlled, leads to failure. Because of the better physical properties, aircraft steels are often used in the normalized state, but seldom, if ever, in the annealed state. One of the most important uses of normalizing in aircraft work is in welded parts. Welding causes strains to be set up in the adjacent material. In addition, the weld itself is a cast structure as opposed to the wrought structure of the rest of the material. These two types of structures have different grain sizes, and to refine the grain as well as to relieve the internal stresses, all welded parts should be normalized after fabrication. Heating the steel above the upper critical point and cooling in still air accomplish normalizing. The more rapid quenching obtained by air-cooling, as compared to furnace cooling, results in a harder and stronger material than that obtained by annealing. Recommended normalizing temperatures for the various types of aircraft steels are listed in Figure. ··· Casehardening Casehardening produces a hard, wear-resistant surface or case over a strong, tough core. Casehardening is ideal for parts that require a wear-resistant surface and, at the same time, must be tough enough internally to withstand the applied loads. The steels best suited to casehardening are the low carbon and low-alloy steels. If high-carbon steel is casehardened, the hardness penetrates the core and causes brittleness. In casehardening, the surface of the metal is changed chemically by introducing a high carbide or nitride content. The core is unaffected chemically. When heat-treated, the surface responds to hardening while the core toughens. The common forms of casehardening are carburizing, cyaniding, and nitriding. Since cyaniding is not used in aircraft work, only carburizing and nitriding are discussed in this section. Carburizing Carburizing is a casehardening process in which carbon is added to the surface of low-carbon steel. Thus, carburized steel has a high-carbon surface and a low-carbon interior. When the carburized steel is heat-treated, the case is hardened while the core remains soft and tough. A common method of carburizing is called “pack carburizing.” When carburizing is to be done by this method, the steel parts are packed in a container with charcoal or some other material rich in carbon. The container is then sealed with fire clay, placed in a furnace, heated to approximately 1,700 °F, and soaked at that temperature for several hours. As the temperature increases, carbon monoxide gas forms inside the container and, being unable to escape, combines with the gamma iron in the surface of the steel. The depth to which the carbon penetrates depends on the length of the soaking period. For example, when carbon steel is soaked for 8 hours, the carbon penetrates to a depth of about 0.062 inch. In another method of carburizing, called “gas carburizing,” a material rich in carbon is introduced into the furnace atmosphere. The carburizing atmosphere is produced by using various gases or by the burning of oil, wood, or other materials. When the steel parts are heated in this atmosphere, carbon monoxide combines with the gamma iron to produce practically the same results as those described under the pack carburizing process. A third method of carburizing is that of “liquid carburizing.” In this method, the steel is placed in a molten salt bath that contains the chemicals required to produce a case comparable with one resulting from pack or gas carburizing. Alloy steels with low-carbon content, as well as low-carbon steels, may be carburized by any of the three processes. However, some alloys, such as nickel, tend to retard the absorption of carbon. Thus, the time required to produce a given thickness of case varies with the composition of the metal. ··· Nitriding Nitriding is unlike other casehardening processes in that, before nitriding, the part is heat-treated to produce definite physical properties. Thus, parts are hardened and tempered before being nitrided. Most steels can be nitrided, but special alloys are required for best results. These special alloys contain aluminum as one of the alloying elements and are called “nitralloys.” In nitriding, the part is placed in a special nitriding furnace and heated to a temperature of approximately 1,000 °F. With the part at this temperature, ammonia gas is circulated within the specially constructed furnace chamber. The high temperature cracks the ammonia gas into nitrogen and hydrogen. The ammonia, which does not break down, is caught in a water trap below the regions of the other two gases. The nitrogen reacts with the iron to form nitride. The iron nitride is dispersed in minute particles at the surface and works inward. The depth of penetration depends on the length of the treatment. Soaking periods (as long as 72 hours) are frequently required to produce the desired thickness during nitriding. Nitriding can be accomplished with a minimum of distortion, because of the low temperature at which parts are casehardened and because no quenching is required after exposure to the ammonia gas. RELATED POSTS Heat Treatment of Nonferrous Metals Properties of Metals Ferrous Aircraft Metals Nonferrous Aircraft Metals Metalworking Processes Precipitation Heat Treating and Annealing of Aluminum Alloys Heat Treatment of Aluminum Alloy Rivets, Magnesium Alloys and Titanium ··· Tags Aircraft Materials Share Previous Post Next Post Sponsored Content Recommended by Upgrade to Office 2024 (Get Now) ps.sbs Get More Out of Your Browsing Experience With our Custom Content DiscoveryFeed Contact | Privacy | Terms | Advertise Copyright @ 2024 Aeronautics-Guide --> Home Aircraft Materials SEARCH Metalworking Processes Search FLYING TRAINING Airplane Flying Helicopter Flying Instrument Flying There are three methods of metalworking: hot working, cold working, and extruding. The method used Aeronautical Knowledge depends on the metal involved and the part required, although in some instances both hot and cold Aviation Instructor working methods may be used to make a single part. Insrtument Procedures Hot Working Almost all steel is hot worked from the ingot into some form from which it is either hot or cold worked to the finished shape. When an ingot is stripped from its mold, its surface is solid, but the interior is still molten. The ingot is then placed in a soaking pit, which retards loss of heat, and the molten interior ··· gradually solidifies. After soaking, the temperature is equalized throughout the ingot, then it is reduced to intermediate size by rolling, making it more readily handled. The rolled shape is called a bloom when its section dimensions are 6 inches × 6 inches or larger and square. The section is called a billet when it is square and less than 6 inches × 6 inches. Rectangular sections, which have a width greater than twice their thickness, are called slabs. The slab is the intermediate shape from which sheets are rolled. Blooms, billets, or slabs are heated above the critical range and rolled into a variety of shapes of uniform cross section. Common rolled shapes are sheet, bar, channel, angle, and I-beam. Hot-rolled material is frequently finished by cold rolling or drawing to obtain accurate finish dimensions and a bright, smooth ··· surface. Complicated sections, which cannot be rolled, or sections of which only a small quantity is required, are usually forged. Forging of steel is a mechanical working at temperatures above the critical range to shape the metal as desired. Forging is done either by pressing or hammering the heated steel until the desired shape is obtained. ··· Pressing is used when the parts to be forged are large and heavy; this process also replaces hammering where high-grade steel is required. Since a press is slow acting, its force is uniformly transmitted to the center of the section, thus affecting the interior grain structure, as well as the exterior to give the best possible structure throughout. Hammering can be used only on relatively small pieces. Since hammering transmits its force almost instantly, its effect is limited to a small depth. Thus, it is necessary to use a very heavy hammer or to subject the part to repeated blows to ensure complete working of the section. If the force applied is too weak to reach the center, the finished forged surface is concave. If the center was properly worked, the surface is convex or bulged. The advantage of hammering is that the operator has control over both the amount of pressure applied and the finishing temperature and can produce small parts of the highest grade. This type of forging is usually referred to as smith forging. It is used extensively where only a small number of parts are needed. Considerable machining time and material are saved when a part is smith forged to approximately the finished shape. Steel is often harder than necessary and too brittle for most practical uses when put under severe internal strain. To relieve such strain and reduce brittleness, it is tempered after being hardened. This consists of heating the steel in a furnace to a specified temperature and then cooling it in air, oil, water, or a special solution. Temper condition refers to the condition of metal or metal alloys with respect to hardness or toughness. Rolling, hammering, or bending these alloys, or heat treating and aging them, causes them to become tougher and harder. At times, these alloys become too hard for forming and must be re-heat treated or annealed. Metals are annealed to relieve internal stresses, soften the metal, make it more ductile, and refine the grain structure. Annealing consists of heating the metal to a prescribed temperature, holding it there for a specified length of time, and then cooling the metal back to room temperature. To produce maximum softness, the metal must be cooled very slowly. Some metals must be furnace cooled; others may be cooled in air. Normalizing applies to iron base metals only. Normalizing consists of heating the part to the proper temperature, holding it at that temperature until it is uniformly heated, and then cooling it in still air. Normalizing is used to relieve stresses in metals. Strength, weight, and reliability are three factors that determine the requirements to be met by any material used in airframe construction and repair. Airframes must be strong and yet as lightweight as possible. There are very definite limits to which increases in strength can be accompanied by increases in weight. An airframe so heavy that it could not support a few hundred pounds of additional weight would be of little use. ··· All metals, in addition to having a good strength-toweight ratio, must be thoroughly reliable, thus minimizing the possibility of dangerous and unexpected failures. In addition to these general properties, the material selected for a definite application must possess specific qualities suitable for the purpose. The material must possess the strength required by the dimensions, weight, and use. The five basic stresses that metals may be required to withstand are tension, compression, shear, bending, and torsion. The tensile strength of a material is its resistance to a force, which tends to pull it apart. Tensile strength is measured in pounds per square inch (psi) and is calculated by dividing the load in pounds required to pull the material apart by its cross-sectional area in square inches. The compression strength of a material is its resistance to a crushing force, which is the opposite of tensile strength. Compression strength is also measured in psi. When a piece of metal is cut, the material is subjected, as it comes in contact with the cutting edge, to a force known as shear. Shear is the tendency on the part of parallel members to slide in opposite directions. It is like placing a cord or thread between the blades of a pair of scissors (shears). The shear strength is the shear force in psi at which a material fails. It is the load divided by the shear area. Bending can be described as the deflection or curving of a member due to forces acting upon it. The bending strength of material is the resistance it offers to deflecting forces. Torsion is a twisting force. Such action would occur in a member fixed at one end and twisted at the other. The torsional strength of material is its resistance to twisting. The relationship between the strength of a material and its weight per cubic inch, expressed as a ratio, is known as the strength-to-weight ratio. This ratio forms the basis for comparing the desirability of various materials for use in airframe construction and repair. Neither strength nor weight alone can be used as a means of true comparison. In some applications, such as the skin of monocoque structures, thickness is more important than strength. In this instance, the material with the lightest weight for a given thickness or gauge is best. Thickness or bulk is necessary to prevent bucking or damage caused by careless handling. Corrosion is the eating away or pitting of the surface or the internal structure of metals. Because of the thin sections and the safety factors used in aircraft design and construction, it would be dangerous to select a material possessing poor corrosion-resistant characteristics. Another significant factor to consider in maintenance and repair is the ability of a material to be formed, bent, or machined to required shapes. The hardening of metals by cold working or forming is termed work hardening. If a piece of metal is formed (shaped or bent) while cold, it is said to be cold worked. Practically all the work an aviation mechanic does on metal is cold work. While this is convenient, it causes the metal to become harder and more brittle. If the metal is cold worked too much, that is, if it is bent back and forth or hammered at the same place too often, it will crack or break. Usually, the more malleable and ductile a metal is, the more cold working it can stand. Any process that involves controlled heating and cooling of metals to develop certain desirable characteristics (such as hardness, softness, ductility, tensile strength, or refined grain structure) is called heat treatment or heat-treating. With steels, the term “heat-treating” has a broad meaning and includes processes such as annealing, normalizing, hardening, and tempering. ··· In the heat treatment of aluminum alloys, only two processes are included: the hardening and toughening process and the softening process. The hardening and toughening process is called heat- treating, and the softening process is called annealing. Aircraft metals are subjected to both shock and fatigue (vibrational) stresses. Fatigue occurs in materials that are exposed to frequent reversals of loading or repeatedly applied loads, if the fatigue limit is reached or exceeded. Repeated vibration or bending ultimately causes a minute crack to occur at the weakest point. As vibration or bending continues, the crack lengthens until the part completely fails. This is termed “shock and fatigue failure.” Resistance to this condition is known as shock and fatigue resistance. It is essential that materials used for critical parts be resistant to these stresses. Heat treatment is a series of operations involving the heating and cooling of metals in the solid state. Its purpose is to change a mechanical property, or combination of mechanical properties, so that the metal is more useful, serviceable, and safe for a definite purpose. By heat-treating, a metal can be made harder, stronger, and more resistant to impact. Heat-treating can also make a metal softer and more ductile. No one heat-treating operation can produce all these characteristics. In fact, some properties are often improved at the expense of others. In being hardened, for example, a metal may become brittle. The various heat-treating processes are similar in that they all involve the heating and cooling of metals. They differ, however, in the temperatures to which the metal is heated, the rate at which it is cooled, and, of course, in the result. The most common forms of heat treatment for ferrous metals are hardening, tempering, normalizing, annealing, and casehardening. Most nonferrous metals can be annealed and many of them can be hardened by heat treatment. However, there is only one nonferrous metal, titanium, that can be casehardened, and none can be tempered or normalized. Internal Structure of Metals The results obtained by heat treatment depend on the structure of the metal and on the way the structure changes when the metal is heated and cooled. A pure metal cannot be hardened by heat treatment, because there is little change in its structure when heated. On the other hand, most alloys respond to heat treatment since their structures change with heating and cooling. An alloy may be in the form of a solid solution, a mechanical mixture, or a combination of a solid solution and a mechanical mixture. When an alloy is in the form of a solid solution, the elements and compounds that form the alloy are absorbed, one into the other, in much the same way that salt is dissolved in a glass of water, and the constituents cannot be identified even under a microscope. When two or more elements or compounds are mixed but can be identified by microscopic examination, a mechanical mixture is formed. A mechanical mixture can be compared to the mixture of sand and gravel in concrete. The sand and gravel are both visible. Just as the sand and gravel are held together and kept in place by the matrix of cement, the other constituents of an alloy are embedded in the matrix formed by the base metal. An alloy in the form of a mechanical mixture at ordinary temperatures may change to a solid solution when heated. When cooled back to normal temperature, the alloy may return to its original structure. On the other hand, it may remain a solid solution or form a combination of a solid solution and mechanical mixture. An alloy, which consists of a combination of solid solution and mechanical mixture at normal temperatures, may change to a solid solution when heated. When cooled, the alloy may remain a solid solution, return to its original structure, or form a complex solution. ··· Heat-Treating Equipment Successful heat treating requires close control over all factors affecting the heating and cooling of metals. Such control is possible only when the proper equipment is available and the equipment is selected to fit the job. Thus, the furnace must be of the proper size and type and must be controlled so that temperatures are kept within the limits prescribed for each operation. Even the atmosphere within the furnace affects the condition of the part being heat-treated. Further, the quenching equipment and the quenching medium must be selected to fit the metal and the heat-treating operation. Finally, there must be equipment for handling parts and materials, for cleaning metals, and for straightening parts. Furnaces and Salt Baths There are many different types and sizes of furnaces used in heat treatment. As a rule, furnaces are designed to operate in certain specific temperature ranges and attempted use in other ranges frequently results in work of inferior quality. In addition, using a furnace beyond its rated maximum temperature shortens its life and may necessitate costly and time-consuming repairs. Fuel-fired furnaces (gas or oil) require air for proper combustion, and an air compressor or blower is therefore necessary. These furnaces are usually of the muffler type; that is, the combustion of the fuel takes place outside of and around the chamber in which the work is placed. If an open muffler is used, the furnace should be designed to prevent the direct impingement of flame on the work. In furnaces heated by electricity, the heating elements are generally in the form of wire or ribbon. Good design requires incorporation of additional heating elements at locations where maximum heat loss may be expected. Such furnaces commonly operate at up to a maximum temperature of about 2,000 °F. Furnaces operating at temperatures up to about 2,500 °F usually employ resistor bars of sintered carbides. ··· Temperature Measurement and Control A thermoelectric instrument, known as a pyrometer, measures temperature in the heat-treating furnace. This instrument measures the electrical effect of a thermocouple and, hence, the temperature of the metal being treated. A complete pyrometer consists of three parts: a thermocouple, extension leads, and meter. Furnaces intended primarily for tempering may be heated by gas or electricity and are frequently equipped with a fan for circulating the hot air. Salt baths are available for operating at either tempering or hardening temperatures. Depending on the composition of the salt bath, heating can be conducted at temperatures as low as 325 °F to as high as 2,450 °F. Lead baths can be used in the temperature range of 650 °F to 1,700 °F. The rate of heating in lead or salt baths is much faster in furnaces. Heat-treating furnaces differ in size, shape, capacity, construction, operation, and control. They may be circular or rectangular and may rest on pedestals or directly on the floor. There are also pit-type furnaces, which are below the surface of the floor. When metal is to be heated in a bath of molten salt or lead, the furnace must contain a pot or crucible for the molten bath. The size and capacity of a heat-treating furnace depends on the intended use. A furnace must be capable of heating rapidly and uniformly, regardless of the desired maximum temperature or the mass of the charge. An oven-type furnace should have a working space (hearth) about twice as long and three times as wide as any part that is heated in the furnace. Accurate temperature measurement is essential to good heat-treating. The usual method is by means of thermocouples: the most common base metal couples are copper-constantan (up to about 700 °F), iron-constantan (up to about 1,400 °F), and chromel-alumel (up to about 2,200 °F). The most common noble metal couples (which can be used up to about 2,800 °F) are platinum coupled with either the alloy 87 percent platinum (13 percent rhodium) or the alloy 90 percent platinum (10 percent rhodium). The temperatures quoted are for continuous operation. The life of thermocouples is affected by the maximum temperature (which may frequently exceed those given above) and by the furnace atmosphere. Iron-constantan is more suited for use in reducing and chromel-alumel in oxidizing atmospheres. Thermocouples are usually encased in metallic or ceramic tubes closed at the hot end to protect them from the furnace gases. A necessary attachment is an instrument, such as a millivoltmeter or potentiometer, for measuring the electromotive force generated by the thermocouple. In the interest of accurate control, place the hot junction of the thermocouple as close to the work as possible. The use of an automatic controller is valuable in controlling the temperature at the desired value. Pyrometers may have meters either of the indicating type or recording type. Indicating pyrometers give direct reading of the furnace temperature. The recording type produces a permanent record of the temperature range throughout the heating operation by means of an inked stylus attached to an arm, which traces a line on a sheet of calibrated paper or temperature chart. Pyrometer installations on all modern furnaces provide automatic regulation of the temperature at any desired setting. Instruments of this type are called controlling potentiometer pyrometers. They include a current regulator and an operating mechanism, such as a relay. ··· Heating The object in heating is to transform pearlite (a mixture of alternate strips of ferrite and iron carbide in a single grain) to austenite as the steel is heated through the critical range. Since this transition takes time, a relatively slow rate of heating must be used. Ordinarily, the cold steel is inserted when the temperature in the furnace is from 300 °F to 500 °F below the hardening temperature. In this way, too rapid heating through the critical range is prevented. If temperature-measuring equipment is not available, it becomes necessary to estimate temperatures by some other means. An inexpensive, yet accurate method involves the use of commercial crayons, pellets, or paints that melt at various temperatures within the range of 125 °F to 1,600 °F. The least accurate method of temperature estimation is by observation of the color of the hot hearth of the furnace or of the work. The heat colors observed are affected by many factors, such as the conditions of artificial or natural light, the character of the scale on the work, and so forth. Steel begins to appear dull red at about 1,000 °F, and as the temperature increases, the color changes gradually through various shades of red to orange, to yellow, and finally to white. A rough approximation of the correspondence between color and temperature is indicated in Figure 1. Figure 1. Temperature chart indicating conversion of Centigrade to Fahrenheit or vice versa, color temperature scale for hardening temperature range, and tempering temperature range It is also possible to secure some idea of the temperature of a piece of carbon or low alloy steel, in the low temperature range used for tempering, from the color of the thin oxide film that forms on the cleaned surface of the steel when heated in this range. The approximate temperature/color relationship is indicated on the lower portion of the scale in Figure 1. It is often necessary or desirable to protect steel or cast iron from surface oxidation (scaling) and loss of carbon from the surface layers (decarburization). Commercial furnaces, therefore, are generally equipped with some means of atmosphere control. This usually is in the form of a burner for burning controlled amounts of gas and air and directing the products of combustion into the furnace muffle. Water vapor, a product of this combustion, is detrimental and many furnaces are equipped with a means for eliminating it. For furnaces not equipped with atmosphere control, a variety of external atmosphere generators are available. The gas so generated is piped into the furnace and one generator may supply several furnaces. If no method of atmosphere control is available, some degree of protection may be secured by covering the work with cast iron borings or chips. Since the liquid heating medium surrounds the work in salt or lead baths, the problem of preventing scaling or decarburization is simplified. Vacuum furnaces also are used for annealing steels, especially when a bright non-oxidized surface is a prime consideration. ··· Soaking The temperature of the furnace must be held constant during the soaking period, since it is during this period that rearrangement of the internal structure of the steel takes place. Soaking temperatures for various types of steel are specified in ranges varying as much as 100 °F. [Figure 2] Small parts are soaked in the lower part of the specified range and heavy parts in the upper part of the specified range. The length