Solar Thermal Systems PDF
Document Details
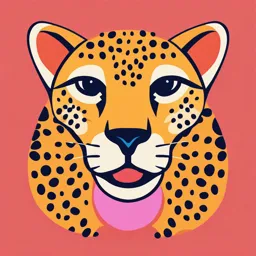
Uploaded by LuxuriousLagoon
Università di Padova
D. Del Col
Tags
Summary
These lecture notes cover Solar Thermal Systems, discussing renewable energy technologies, sources, and applications. Information on solar collectors, types of systems, and performance is included.
Full Transcript
Renewable energy technologies Solar Thermal Systems D. Del Col Dipartimento di Ingegneria Industriale Università di Padova Sources: - Lecture notes and slides - Duffie and Beckman, Solar Engineering of Thermal Process...
Renewable energy technologies Solar Thermal Systems D. Del Col Dipartimento di Ingegneria Industriale Università di Padova Sources: - Lecture notes and slides - Duffie and Beckman, Solar Engineering of Thermal Processes, Ed. WILEY https://onlinelibrary.wiley.com/doi/epub/10.1002/9781119540328 Consultation: AAVV, Planning and Installing Solar Thermal Systems. Ed. EARTHSCAN INTRODUCTION Solar radiation can be collected and converted into thermal energy through solar collectors. A solar thermal system allows us to reduce the energy consumption of the building from fossil sources and therefore the amount of carbon dioxide introduced into the atmosphere for combustion processes. Solar energy has characteristics of discontinuity in space and time. A system that uses solar energy requires integration with an auxiliary system. ✓ Environmental benefit: avoided kg of CO2 ✓ Economic benefit: favourable pay-back time SOLAR THERMAL SYSTEM AUXILIARY SYSTEM STORAGE COLLECTOR USER APPLICATIONS Heating of outdoor swimming pools Required temperature: 25 ÷ 27 °C Energy demand coinciding with the most favorable period for solar radiation Sanitary water heating Required temperature: 45 ÷ 60 °C Energy demand constant all year round Space heating Required temperature: depends on the type of terminals Energy demand coinciding with the most unfavorable period for solar radiation Solar cooling Required temperature: higher than 80 °C Energy demand coinciding with the most favorable period for solar radiation SOLAR THERMAL MARKET SOLAR THERMAL MARKET Shares for newly installed capacity SOLAR THERMAL MARKET New installations for 1000 capita SOLAR THERMAL MARKET Capacity in operation for 1000 capita SOLAR COLLECTOR It collects the solar radiation incident on its surface to heat a working fluid ▪ Glazed flat collectors (covered) ▪ Vacuum collectors ▪ Flat unglazed collectors (uncovered) Efficiency [%] PERFORMANCE Evacuated tube collector Unglazed collector Glazed collector T* = reduced temperature TYPE OF SYSTEMS: classifications ▪ Natural circulation systems: gravity driven circulation ▪ Forced circulation systems: pump driven circulation ▪ Open circuit (direct): the water intended for users passes directly into the collectors. Solution suitable only for areas not subject to freezing in winter. ▪ Closed circuit (indirect): the system can be divided into a solar circuit (primary) and a secondary circuit where the water intended for users flows. FORCED CIRCULATION SYSTEM Circulation pump in the collectors circuit NATURAL CIRCULATION SYSTEM The collectors circuit is not equipped with a pump: the heat transfer liquid heating up in the collectors decreases in density and rises towards the storage, triggering a spontaneous motion. GLAZED FLAT COLLECTOR Containment frame or box. Transparent glass cover. Absorber plate. Channels or tubes in which the heat transfer liquid flows. Insulation. There is air in the gap between the absorbent plate and the glass plate THE ABSORBER PLATE It generally consists of a single metal sheet in copper, aluminum or stainless steel. Its surface is treated with a selective coating. Absorber plate with serpentine or parallel tubes. Plates with parallel tubes Serpentine plate THE SELECTIVE COATING Non-selective plate Selective plate Transparent Transparent cover cover Low High wavelenght emission Low wavelenght High absorption ABSORBER PLATE With simple hypotheses, proceeding iteratively, it is possible to calculate - knowing the geometry Relative intensity and the materials of the collector - what is the 1000 K 500 K thermal dispersion coefficient, which is then normally evaluated by the efficiency test. In defining the dispersion coefficient, the most important variables are the number of transparent covers and the possible selectivity of plate and covers. Reflectance To understand the importance of selectivity, it must curve be remembered that the wavelenghts of the spectrum of the incoming solar radiation and the infrared radiation dispersed by the collector are very different. It is therefore advantageous that the plate displays a strong absorption in the solar spectrum and a strong reflection in the infrared. Remember: ++= Characteristics of selective coatings on the market TRASPARENT COVER Coefficient of transparency (in the normal direction) as a function of the wavelength of a 1 mm thick sheet of optical glass. Glass (with a low iron content, to enhance transparency at low wavelengths) is the ideal material for the cover of flat solar collectors, as it is substantially transparent to solar radiation, and substantially opaque to infrared radiation emitted by the plate. ABSORBER PLATE When heated, the plate emits heat by radiation in the infrared field: the selective coating allows to maximize the heat absorbed and at the same time minimize the heat emissions due to surface heating. These characteristics are indicated with the terms absorption and emission. Currently a solar collector has an absorption of 0.95 (95%) and an emission of 0.05 (5%). Serpentine plate (meander) It has higher pressure drop: it is not suitable for natural circulation systems. Operation even with low liquid flow rate because it ensures a turbulent flow. Parallel tube plate (harp) Limited pressure drop: they are also suitable for natural circulation systems. THE HEAT TRANSFER FLUID It must have good thermal properties. It must guarantee frost protection. It must not be corrosive. It must be resistant to the high temperatures that can be reached in the collector in particular conditions. The fluid that represents a good compromise among the listed properties is a mixture of water and glycol (generally propylene) containing corrosion inhibitors ▪ Glycol is incompatible with some materials, such as zinc. ▪ A 40% glycol mixture guarantees operation down to a temperature of approximately - 24°C. ▪ Better not exceed the percentage of glycol: possibly avoid a glycol content higher than 50% in order not to increase the viscosity too much. Freezing points Fluids: viscosity Kinematic viscosity as a function of temperature. The concentration is chosen to have a freezing point at -20°C Example of FLAT COLLECTORS ENERGY LOSSES IN THE COLLECTOR THERMAL EFFICIENCY OF THE COLLECTOR Not all solar energy that reaches the surface of the collector can be converted into heat and made available to users: Efficiency = Q3 / E0 ( 2,5 User Water main THERMAL STRATIFICATION Poor thermal stratification of the storage has these consequences: The maximum achievable water temperature is lower. The mixed area, characterized by an average water temperature, is more extended. The thermal energy obtained is of lower quality: the exergy of the water is lower Storage is less efficient: the auxiliary heating system intervenes more frequently and at partial load. FORCED FLOW SYSTEM: expansion vessel The function of the expansion vessel is: - compensate for changes in liquid volume that occur with increasing temperature. - avoid the leakage of heat transfer fluid through the safety valves: intrinsically safe system. The expansion vessel must be installed in an uninsulated point of the solar circuit return line to reduce the thermal load on the membrane. It may be useful to install the vessel with the liquid side up and the gas side down to limit the thermal stress to which the membrane can be subjected. FORCED FLOW SYSTEM: control system T 1 T2 T3 (T1-T3) > 6 ÷ 10° C: the solar circuit pump starts T2 < 45 °C: the boiler turns on T2 > 90 °C: the system is blocked FORCED FLOW SYSTEM: control system The pump starts when the temperature difference between the lower part of the storage tank and the hottest part of the collectors exceeds a certain value (6 ÷ 10°C) and switches off when this temperature difference falls below a minimum value (3 ÷ 4°C). SANITARY WATER HEATING SPACE HEATING SYSTEMS They are convenient in locations with a long heating season (October to April), in order to take advantage of the good spring and autumn radiation. It is essential to limit the dispersion of the building envelope and use low temperature system terminals. The collectors must have a high inclination. DESIGN To size a solar system it is necessary to know the coverage factor of the system: this implies the knowledge of an average yield of the collectors. It is necessary to establish the monthly fraction f of the thermal energy requirement supplied by the solar system. The annual coverage fraction F is subsequently determined starting from the monthly fraction values obtained with the chosen calculation method. A procedure of this type is adopted by the method of the f-chart where the monthly fraction f is expressed through an empirical correlation. EVALUATION OF THERMAL LOAD Production of domestic hot water The evaluation of the daily heat load per capita for the production of domestic hot water is linked to the consumption of water per person: L = 40 ÷ 60 liters / day The daily heat load per person is equal to: Q = L c p ( tm – tr ) [J/day] tm : water supply temperature [°C] tr : water temperature from the supply network [°C] cp : specific heat of water [ 4186 J/(kg K) ≈ J/(L K) ] Sizing principles: domestic hot water Input data: location, number of people, orientation and inclination of the collectors Historical data of solar radiation + calculation model to take into account the inclination of the surface to calculate the average monthly daily solar radiation on the inclined plane [kWh/m2] H Monthly energy requirement [kWh] QTOT Evaluation of the average efficiency of the solar collector system Choice of the surface to be installed [m2] A (first attempt) Calculation of useful energy [kWh] Qu H A Qu Solar fraction: FS QTOT If the value of FS is not considered "satisfactory", another value must be chosen for A. Exercise: system for hot water production Calculation of the heat demand for domestic hot water for a family of five persons. Input data Twater,cold = 15 °C, Twater,hot = 45 °C, Lwater= 50 L/(day person) Np = 5 cp,water = 4185 J/(kg K), rwater = 995 kg/m3 Results Qwater ,day Lwater / 1000 N p r water c p ,water Twater ,hot Twater ,cold / 3600000 Qwater , year Qwater ,day N days the daily and yearly heat demands of domestic water are: Qwater,day = 8.67 kWh Qwater,year = 3165 kWh Yearly solar irradiation in the plane of the collectors Location: Padova (45.4°N, 11.9°E) Orientation: South Tilt = 35° (respect to the horizontal plane) Yearly global solar irradiation on the horizontal plane: Hh,year = 1300 kWh/m2 Yearly global solar irradiation on the tilted plane: Htilt,year = 1510 kWh/m2 The tilt angle of 35° maximizes the yearly collection of solar radiation for the site of Padova. Exercise Calculation of the aperture area of solar collectors Input data Location: Padova (45.4°N, 11.9°E). Solar collectors installed due South, 35° tilted to the horizontal plane. Heat demand: heating of domestic water for 5 persons. Yearly efficiency of the solar thermal system: 0.40 Yearly solar fraction: 0.70 Results the yearly heat demand and the available solar irradiation on the plane of the collectors are: Qwater,year = 3165 kWh Htilt,year = 1510 kWh/m2 In the present example, for the site of Padova, the following values of the yearly efficiency of the solar system and the yearly solar fraction can be considered: system,year = 0.40 , SFyear = 0.70 SFyear Qwater , year From the equation: system, year H tilt , year Acoll it is possible to calculate the area of the solar collectors needed to cover 70% of the yearly heat demand of domestic hot water: SFyearQwater ,year Acoll 3.7 m 2 H tilt ,year system,year f - CHART METHOD Monthly fraction: f = Qsol / QL = 1 - Qaux / QL Qsol : useful solar energy [J] Qaux : heat from auxiliary system [J] QL : monthly thermal energy requirement [J] water daily consumption DT of water during heating Simulations performed on a wide range of values for the design parameters led to the following correlation for water systems: f = 1,029Y – 0,065X – 0,245Y2 + 0,0018X2 + 0,0215Y3 Y can be interpreted as the ratio between the total useful energy absorbed and the thermal load: 0 < Y < 3 X can be interpreted as the ratio between the thermal losses of the collectors at the reference temperature and the load: 0 < X < 18 f - CHART METHOD F ' R AC ( ) FR' ( ) H N Y H N FR ( ) n AC QL FR ( ) n QL F 'R AC FR' A X U L (Trif Td )Dt FRU L (Trif Td )Dt C QL FR QL FR: collector heat dissipation factor AC: collector area [ m2 ] F’R/FR: factor to account for the presence of the heat exchanger H: monthly average daily solar energy on the inclined surface [J/(m2 d)] N: number of days in the month Tdd: : average daytime temperature [°C ] T Trif: reference temperature [°C ] UL: overall dispersion coefficient of the collector [ W/(m2K) ] : describes the transmission through the glazed surface and the absorption of the plate Dt: duration of the month [ s ] QL: monthly energy requirement [ J ] f - CHART METHOD Historical data of solar radiation + Calculation models to take into H account the surface inclination QL Monthly energy needs of the building FR ( ) n Efficiency value at null reduced temperature FRU L Slope of the efficiency line FR' f ( FRU L , sc ) Efficiency of the heat exchanger sc FR Once the total area of the collectors to be installed has been chosen, it is possible to calculate the X and Y parameters and therefore the monthly and annual solar fractions. SOLAR CONTRIBUTION Solar Fraction: FS = Qsol / QL The monthly solar fraction must not "exceed" 100% during the summer period to avoid a production of thermal energy higher than the demand. Yearly value EN 15316-4-3:2017: Energy performance of buildings - Method for calculation of system energy requirements and system efficiencies – Part 4-3: Heat generation systems, thermal solar and photovoltaic systems This standard reports methods for calculating the solar contribution for systems in buildings: combined systems for winter heating and domestic hot water production, systems for winter heating only and systems for only production of domestic hot water. For the different types of system, it considers two distinct calculation methodologies, both based on the monthly time period: Method 1 – using system test data; Method 2 – monthly, using component specifications. In particular, method 2 adapts and extends the calculation procedure of f- Chart, directly using the characterization parameters of the solar collectors required by the tests according to EN ISO 9806. Method 2 according to EN 15316-4-3 is shown in detail for the calculation of the solar contribution for a system dedicated only to the production of domestic hot water. SOLAR SYSTEM FOR DHW PRODUCTION ONLY Calculation according to EN 15316-4-3 The method directly uses the value of the parameters of the efficiency curve of the collector, referred to the opening area: t t t t 0 a1 m a a2 m a 2 G G η0: efficiency at zero losses [-]; a1: collector heat loss coefficient [W/(m2K)]; a2: temperature dependent heat loss coefficient [W/(m2K2)]; G: hemispherical solar irradiance [W/m2]; A: total aperture area of the collectors [m2]; IAM: incidence angle modifier at 50° [-]. As for the classic method of f-Chart, the fraction of solar coverage f of the monthly thermal energy requirements for the supply of DHW is obtained as a function of two dimensionless characteristic parameters X and Y: X is the similarity ratio between losses and heat requirement; Y is the similarity ratio between solar energy recovery and heat requirement. SOLAR SYSTEM FOR DHW PRODUCTION ONLY Calculation according to EN 15316-4-3 X A Uloop loop DT f st tm /(Qsol ,us ,m 1000) A m2 : area total di apertura aperture areadella totalità dei collettori. of collectors U loop W/ m 2 K : coefficiente thermal dispersion coefficienttermica di dispersione (including tubes) of the circuit of collectors del circuito collettori (anche tubi ) : U loop a1 a2 40 U loop , p / A U loop , p W/K : coefficiente di dispersione global dispersion coefficient globale of all thedi tutte pipes le in tubazioni delcollectors; the circuit of circuito collettori ; può venire it can be calculated directly or : direttamente calcolato, oppure : U loop , p 5 0,5 A. loop : fattore difactor efficiency efficienza in the del circuito circuit collettori of collectors, , inclusa including thel effect ' influenza of heat exchanger; dello scambiatoretypical. Valore tipico loop 0,9. value SOLAR SYSTEM FOR DHW PRODUCTION ONLY Calculation according to EN 15316-4-3 loop può can be analitically essere calcolatocalculated from dalla analiticamente loop 1 D , D 0 A a1 U st hx U st hx : trasmittanza global transmittance globale of the scambiatore dello heat exchanger. DT °C, K : differenza reference temperature difference di temperatura di riferimento DT ref e,avg ref °C : temperatura di riferimento reference temperature; ; per sistemi for DHW : : systemsacs ref 11,6 1,18 w 3,86cw 1,32 e,avg w °C : temperatura desiderata desired temperature ; ; w 40°C per l ' acs for DHW c w °C : temperatura temperature cold water acqua fromda fredda mains rete e,avg °C : temperatura average temperature of external media aria esternaair delinperiodo the period. SOLAR SYSTEM FOR DHW PRODUCTION ONLY Calculation according to EN 15316-4-3 f st : fattore correttivo correction factor del volume Vsol L del for volume tankdi; accumulo; serbatoio of storage ilthe standard valore value fordel standard thevolume è Vref 75 A L volume is 0,25 Vref f st . sol V tm h : durata durationdel periodo of the (mese considered ) in(month) period considerazione. Qsol ,us , m kWh : global fabbisogno termico monthly globale heating mensile requirement for per la fornitura the supply of DHW. di acs. It must also include the heat dispersions of the DHW distribution Deve comprendere anche le dispersioni della rete di network. distribuzione acs. Heat dispersions are usually estimated as an increase by 5-10% Le dispersioni vengono di solito considerate con un fattore of the DHW heating energy. di aumento (5 10%) dell ' energia di riscaldamento dell ' acs. SOLAR SYSTEM FOR DHW PRODUCTION ONLY Calculation according to EN 15316-4-3 Y A IAM 0 loop I m tm Qsol ,us,m 1000 0 : efficiency fattore di efficienza factor del collettore of the collector a referred at zero losses perditetonulle the aperture area according to EN ISO 9806 riferito all ' area di apertura secondo EN 12975 2 IAM : modificatore incidence angle dell ' angolo modifier of the di incidenza collector at 50°del collettore a 50 [ K , 50 ] I m W/m 2 : irradiazione solare media average solar irradiance on thesul pianoindel collector the collettore considered period (month) nel periodo (mese) considerato SOLAR SYSTEM FOR DHW PRODUCTION ONLY Calculation according to EN 15316-4-3 Qsol ,out ,m kWh :energy energia prodotta production dall by the ' impianto solar solare system in the nel considered period (month) periodo (mese) f sol , m : fraction frazione of di copertura solar coverage insolare nel periodo the considered period ((month) mese) Qsol ,out ,m f sol ,m 1,029 Y 0,065 X 0, 245 Y 2 0,0018 X 2 0,0215 Y 3 Qsol ,us ,m f sol , a : fraction frazione of di copertura solar solare coverage in nellyear the whole ' intero anno f sol , a Qsol ,out ,m Qsol ,us,m Exercise: SOLAR SYSTEM FOR DHW PRODUCTION Calculation according to EN 15316-4-3 Location: Padova House of 4 persons. Individual requirement of DHW 50 L/d Mains water temperature 12 °C Desired DHW temperature 40 °C Distribution network losses 5% of DHW heating Forced circulation DHW production solar system with integration boiler, 320 L storage tank, two Immergas flat glazed collectors with selective surface (technical data shown in the following sheet), arranged integrated on the roof with inclination angle on the horizontal plane β = 60° and azimuth γ = 25° Exercise: SOLAR SYSTEM FOR DHW PRODUCTION Calculation according to EN 15316-4-3 Exercise: SOLAR SYSTEM FOR DHW PRODUCTION Calculation according to EN 15316-4-3 Calculation for month of February Total needs of DHW Temp water from mains Desired temp of DHW Average outdoor temp in February at Padova Total area of collectors Data of collectors Storage tank capacity Reference value from standard: 75 L / m2 of collectors Correction factor for tank volume Exercise: SOLAR SYSTEM FOR DHW PRODUCTION Calculation according to EN 15316-4-3 - Calculation for month of February Reference temperature Reference temperature difference Total monthly heating requirement for February (28 days): includes losses in the distribution network, not the solar circuit Qsol,us,m = 200284,186(40-12)FP/3600 = 191,44 kWh FP loss factor = 1,05 Coefficient of losses of solar circuit (collectors and tubes) Coefficient of losses of all the tubes W/K Efficiency factor of the solar circuit Let us assume (it should be later verified) Exercise: SOLAR SYSTEM FOR DHW PRODUCTION Calculation according to EN 15316-4-3 - Calculation for month of February Duration of the month Average irradiance in the period Im = 10,33 MJ/(m2 d) = 10,33 106 / (24 3600) = 119,56 W/m2 Calculation of fraction of solar coverage f= GEN FEB MAR APR MAG GIU LUG Fabbisogno DHW demandacs [L][L] 6200 5600 6200 6000 6200 6000 6200 Temp. Monthly media mese θe,avg [°C] avg. temp. 1,9 4,0 8,4 13,0 17,1 21,3 23,6 Temperatuura riferimento θref [°C] Reference temperature 102,6 99,8 94,0 88,0 82,5 77,0 74,0 Ref. temp. Diff. difference temperatura riferim. ΔT [°C] 100,7 95,8 85,6 75,0 65,4 55,7 50,4 Fattore correttivo Storage vol. vol.factor correction accum. fst [-] 0,9653 Coeff. Losses dispers. tubi Uloop,p [W/(m2K)] coeff tubes 6,852 Coeff. Losses dispers. circ. Uloop [W/(m2K)] coeff circuit 6,108 Fattore efficienza Efficiency circuito ηloop [-] of the circuit 0,900 Lunghezza mese tm [h] Duration month 744 672 744 720 744 720 744 Irradiazione Average solarmed. solare Im [W/m2] irradiance 81,1 119,6 149,4 161,8 178,0 181,6 195,4 Fabbisogno Energy demand energia Qsol,us,m [kWh] 212,0 191,4 212,0 205,1 212,0 205,1 212,0 Parametro X [-] 6,948 6,612 5,908 5,172 4,515 3,843 3,475 Parametro Y [-] 0,6618 0,9753 1,2189 1,3199 1,4521 1,4814 1,5937 Energia dal solar Energy from solare Qsol,out,m [kWh] 45,6 84,1 128,9 142,1 166,7 170,9 190,9 Fattore di copertura Solar coverage factor solare f [-] 0,215 0,439 0,608 0,693 0,787 0,833 0,901 AGO SET OTT NOV DIC ANNO Fabbisogno DHW demandacs [L][L] 6200 6000 6200 6000 6200 73000 Temp. media Monthly mese θe,avg [°C] avg. temp. 23,1 19,7 13,8 8,2 3,6 Temperatuura Reference riferimento θref [°C] temperature 74,6 79,1 86,9 94,3 100,4 Diff.temp. Ref. differenceriferim. ΔT [°C] temperatura 51,5 59,4 73,1 86,1 96,8 Fattore vol. Storage correttivo vol.factor correction accum. fst [-] 0,9653 Coeff. dispers. Losses tubi Uloop,p [W/(m2K)] coeff tubes 6,852 Coeff. dispers. Losses circ. Uloop [W/(m2K)] coeff circuit 6,108 Fattore efficienza Efficiency circuito ηloop [-] of the circuit 0,900 Lunghezza Duration mese tm [h] month 744 720 744 720 744 Irradiazione Average solar med. solare Im [W/m2] irradiance 195,5 186,1 154,9 97,2 96,5 Energy demand Fabbisogno energia Qsol,us,m [kWh] 212,0 205,1 212,0 205,1 212,0 2495,6 Parametro X [-] 3,555 4,099 5,044 5,940 6,676 Parametro Y [-] 1,5946 1,5182 1,2633 0,7931 0,7874 Energiafrom Energy dal solar solare Qsol,out,m [kWh] 190,1 171,6 142,1 71,8 66,8 1571,5 Fattore Solar di copertura coverage factor solare f [-] 0,897 0,837 0,670 0,350 0,315 0,630 Calculation of DHW production with solar panels according to EN 15316-4-3 Solar fraction Months Year SIZING PRINCIPLES: REFERENCE VALUES DOMESTIC HOT WATER Collector area: Flat collector: 0,7 ÷ 1 m2 per person Vacuum collector: 0,5 ÷ 0,8 m2 per person Storage: 70 ÷ 100 liters per m2 of installed collector DOMESTIC HOT WATER AND SPACE HEATING Systems with coverage of up to 35% of space heating needs Collector area: Flat collector: 0,7 ÷ 1 m2 of absorbing surface per 10 m2 of building surface area Vacuum collector: 0,5 ÷ 0,8 m2 of absorbing surface per 10 m2 of building surface area Storage: 50 liters per m2 of installed collector 100 ÷ 200 liters per kW of thermal capacity SIZING THE EXPANSION VESSEL Closed expansion vessel, sizing for traditional heating systems (i.e. when stagnation conditions are excluded): eC V p 1 1 i p f 1 C : overall system capacity [ liters ] e : volume expansion coefficient corresponding to the difference between the fluid temperature in the switched-off cold system and the maximum operating temperature pi : pressure at which the vessel is preloaded [ barg ] pf : safety valve calibration pressure – 0,5 bar [ barg ] V : total volume of the vessel to be installed [ liters ] We can take the following value for e e = 0,000654 [1/K] ·ΔT [K] (water + 40 % propylene glycol) STAGNATION IN SOLAR COLLECTORS If during the summer season the user is unable to withdraw heat from the solar circuit for a certain period, the phenomenon of stagnation may occur inside the collector, characterized by the formation of steam and the achievement of very high temperatures. Stagnation temperature is the temperature of a solar system in periods of time under no flow conditions. It is therefore the fluid temperature in a solar collector in periods of time where no useful energy is taken from solar collector and results from the given irradiance and ambient temperature. Causes: - The sanitary side has reached the maximum allowable temperature because there is no withdrawal by the user. - The pump has been blocked due to a fault. A protection system must be adopted. STAGNATION IN SOLAR COLLECTORS The heat transfer fluid (water and glycol) is subjected to thermal stress which, if too frequent and lasting, can accelerate the aging of the fluid and produce its chemical alteration with the following consequences: - the antifreeze properties deteriorate; - the fluid becomes more aggressive; - some components of the mixture (additives against corrosion or impurities present) can deposit and adhere to the absorber channels. EVAPORATION IN THE SOLAR CIRCUIT The amount of vapor that is formed depends on the construction characteristics of the panels and on the configuration of the collector field. The collectors with inlet located at the bottom during evaporation have a tendency to expel the liquid still present inside them. The collectors with high inlet and outlet have poor emptying capacity during evaporation. Large systems mounted on façades or on very sloping roofs can generate a large amount of steam. Handling STAGNATION Handling STAGNATION Handling STAGNATION Handling STAGNATION Handling STAGNATION Handling STAGNATION Sometimes it may be advisable to connect the expansion tank to a line cooled by means of a so- called dissipator. THE SOLAR CIRCUIT EXPANSION VESSELS FUNCTIONS Compensate for fluctuations in the volume of the heat transfer fluid of the solar circuit, due to thermal expansion and any evaporation of the liquid contained in the collectors. Prevent heat transfer fluid from escaping through the safety valves, acting as a storage from which the liquid, once cooled down, can return to the circuit. SIZING When sizing the vessel, it must be taken into account that the liquid contained in the solar collectors can evaporate. This leads to consider a useful volume equal to the expansion volume of the heat transfer fluid in the circuit increased by the evaporation volume of the collector field. Vu = (ΔVFL + VC) x 1,1 (1,1 = safety index), with Vu = useful volume of the expansion vessel, in liters ΔVFL = (E x VFL); E = thermal expansion of the fluid, for water-glycol with ΔT≈100°C E = 0,07 VFL = fluid content in the solar circuit, in liters VC = fluid content in the solar collectors, in liters. The nominal volume of the expansion vessel can now be determined, as a function of the operating pressures: Vn = Vu x (pF + 1)/( pF – pI), with pF = final pressure in barg. Recommended: safety valve opening pressure - 0,5 bar pI = initial system filling pressure, in barg. Recommended : pstatica + 0,5 bar. SOLAR COOLING Absorption cycle