Physiology of Somatosensory System PDF
Document Details
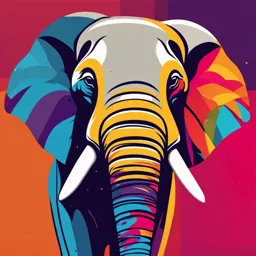
Uploaded by HealthfulTuring
Okan University
Prof. Dr. Lamia Pınar
Tags
Summary
This document discusses the physiology of the somatosensory system, covering somatic and autonomic nervous systems, special senses, receptors, and pain pathways. It details the types of receptors, their functions, and the processes that transmit sensory information to the central nervous system. The text also discusses pain physiology, including the differences between acute and chronic pain, and the modulation of pain signals.
Full Transcript
Physiology of Somatosensory System Faculty of Dentistry and Faculty of Health Science Prof. Dr. Lamia Pınar Okan University Faculty of Medicine Department of Physiology Somatic nervous system, controls movements of our somatic organs like the musculoskeletal system and provides movements such as ref...
Physiology of Somatosensory System Faculty of Dentistry and Faculty of Health Science Prof. Dr. Lamia Pınar Okan University Faculty of Medicine Department of Physiology Somatic nervous system, controls movements of our somatic organs like the musculoskeletal system and provides movements such as reflexes, walking, running, or carrying out an activity with our arms, legs, or any part of our body. It is activated by the needs of the body. The Somatic nervous system mostly works voluntarily. 9/9/12 Autonomic nervous system, controls the functions of our internal organs like the heart, lungs, stomach, liver, intestines and urogenital organs, etc. It is activated by incoming senses from the internal organs and the needs of the body system. The Autonomic nervous system produces reflex activations and works involuntarily. That means it works automatically. Introduction to the Sensory System Special senses are concerned with the perception of stimuli from the external environment. These are the eye for vision, ear for hearing (audition) and equilibrium, nose for smell (olfaction) and oral mucosa for taste. These are all located in the head. Remaining senses such as touch, pressure, proprioception, and pain are sensed by receptors throughout the skin and underlying tissue (subcutaneous area). These receptors cause the body to be aware of activity in the surrounding area and allow appropriate or protective actions to be taken. Unimportant senses are eliminated before entering the CNS The central nervous system receives millions of bits of information from the different sensory nerves and organs each minute. If the incoming senses are not relevant or not important for the body at a given moment, they are suppressed before reaching the somatic sensory cortex. This means that around 99% of sensory inputs that enter the central nervous system, such as the sensations of clothes touching our body or sounds from the environment, are eliminated at some point in the ascending system. RECEPTORS are Cells or parts of the cells in the surface or interior environment of the body that receive sensory information such as light, sound, touch, pain, heat, cold, etc. They may be proteins or glycoproteins. All of them are connected to a sensory axon When a receptor potential reaches a threshold voltage, an action potential is produced in the sensory axon. Receptors can be classified based on their Stimulus Localization Teleceptors: detect distant senses such as light and sound Exteroceptors: perceive sensations from the outer surface of the body such as touch, pressure, heat, and pain… Interoceptors: perceive stimuli from the body's internal environment, such as blood pressure, blood oxygen level, stretching internal organs *The modality of each receptor is unique. For example; Light cannot activate the auditory system, and sound cannot activate the pressure receptors. This is ‘the law of specific energies.’ Classification of Receptors According to Sensory Types Chemoreceptor: stimulated by taste, smell, and chemicals in the blood. Photoreceptor: stimulated by light. Thermoreceptor: stimulated by cold and heat. Mechanoreceptor: stimulated by mechanical deformation of the cell membrane Nociceptor: stimulated by chemicals that occur in tissue damage (and creates pain) Mechanoreceptors Touch and Pressure Receptors (Tactile receptors) Merkel discs can sense continuous touch (adapt slowly; tonic) They are located in both hairy and glabrous skin. Pacinian corpuscles are found in the skin and subcutaneous areas. They are stimulated by rapid movement on the skin (adapt rapidly; phasic). Detect vibration or rapid changes in the tissues. Meissner’s corpuscles are sensitive to the light touch of glabrous skin. It is found in the most sensitive areas of the body, such as the cornea of the eyes, lips, tongue, tops of fingers, nips of the breasts, face … (adapt rapidly; phasic) Types of mechanoreceptors (continue) Ruffini endings produce signals for continuous deformation, such as heavy touch or pressure (adapt very slowly or not, tonic). They are found in deeper tissues and joint capsules, activated with joint rotation. They form the sense of proprioception. Free Nerve Endings, respond to pain, touch and pressure, and thermal stimuli. The myelin sheath is lost at the endings of the axonal branches. Their adaptation depends on the type and intensity of the stimuli. Hair Follicle Receptors are phasic, free nerve endings that can innervate up to 100 hairs spread over several cm² of skin. Sensory Unit One sensory axon and its peripheral branches constitute a ‘sensory unit’ The area where a stimulus produces a sensory effect in the clusters of neighboring receptors is ‘the receptive field’ The intensity of the stimulus depends on the frequency of the impulse transmission or increasing the number of receptors involved simultaneously But the type of sensation (modality) does not change as intensity increases (Law of Specific Energies) Senses are affected by ‘central excitation’ or ‘central inhibition’ states of the nervous system, due to releasing; of; Opiates, or Calcitonin Gene-Related Peptides (CGRP) secreted from the brain Cutaneous and subcutaneous senses (Tactile senses) Beginning of Action Potential in the Pacinian Corpuscle (Two-point Discrimination) The ability to discriminate clearly between two separate stimuli applied together is highly developed in the skin, and poorly developed in deeper tissues and organs. The two points that can be recognized by the cortex must have a distance of at least 70 mm on the back of the body and 1 to 3 mm on the fingertips or lips. These difference depends on the sensitivity and the amount of the sensory receptors detect the stimuli. Types of somatic receptors (dorsal root ganglion-spinal ganglion) All the somatic afferents have their cell bodies in the spinal ganglion. All the sensory fibers are excitatory fibers and secrete glutamate. All the somatic and part of the autonomic sensory fibers enter the spinal cord via dorsal roots, and all the motor fibers going to the musculature leave the spinal cord from the anterior (ventral) column via ventral roots. This is Bell-Magendie law. Dorsal Column - Medial Lemniscus System (proprioception, fine touch, fine pressure) Receptors: muscle spindles, Golgi tendon organs, Ruffini Endings. Axons: thick, myelinated First neurons: Spinal Ganglion (Dorsal root ganglion) Afferents travel in the dorsal horn of the spinal cord and are called Fasciculus Gracilis and Fasciculus cuneatus Second neurons: Nucleus Gracilis and Nucleus Cuneatus in the Bulbus Fibers cross to the opposite side. Fibers are named Medial Lemniscus and go up. Third neurons are located in the ventral posterolateral nucleus of the Thalamus Fourth neurons are located in the postcentral gyrus (Brodman’s 3,1,2 area) Lesions in the Dorsal Column-Medial Lemniscal System causes; Disorders of Balance (unsteady during standing) Ataxia (drunken-like walking, swinging during eyes closed) Astereognosis: inability to distinguish the shape of the objects handled, when eyes are closed PAIN PHYSIOLOGY Prof. Dr. Lamia Pınar İstanbul Okan University Medical Faculty Dpartment of Physiology OBJECTIVES After studying this chapter, you should be able to: Describe the receptors that mediate the sensations of pain and temperature Explain the differences between pain and nociception, first and second pain, acute and chronic pain, hyperalgesia, and allodynia. Describe and explain visceral and referred pain Describe processes involved in the modulation of transmission in pain pathways. Relief of pain Pain or Nociception; is a protective mechanism. It provides a reflex activity to withdraw the parts of the body from a harmful object or alert the brain. Fast pain, also called acute pain or physiological pain, is transmitted to the central nervous system by thin myelinated A delta (Aδ) fibers. Fast pain, mostly occurs in the skin and underlying tissues by chemical, thermal, mechanical, or polymodal (all of them) stimuli. It is felt as burning, pinprick, knife wounded, electric shock, etc. It is sharp, and intense pain. It can be localized and recognized by its sharpness and intensity. Slow pain (pathological pain) usually occurs due to tissue damage It is transmitted to the central nervous system by thin unmyelinated C fibers. It can create an unbearable feeling when it prolonges, even if its intensity is not very high. Slow pain is also called chronic or visceral pain because it is generally produced by inflammation, infection, or stretching of the visceral organs or damage to the deep tissues such as joints. The pathway of chronic pain activates the reticular activating system (RAS) in the brainstem and causes the brain to become alert about the matter. Pain Receptors: (NOCICEPTORS) For both acute and chronic pain, the receptors are ‘Free Nerve Endings’ (or naked nerve endings) Pain receptors are called nociceptors. They are located in the skin, subcutaneous and deep tissues, and in the visceral organs Acute pain fiber’s neurotransmitter is Glutamate Chronic pain fiber’s neurotransmitter is Substance P (Lewis' P factor) After a tissue injury; inflammation occurs in the area The main symptoms of the inflammation (flare) are, - Redness, - Fever - Swelling - Pain Both fast and slow pain systems travel to the CNS by Lateral Spinothalamic pathways (in the Antero-Lateral System) Fast Pain Slow Pain Sharp, pricking Dull, burning Thin myelinated (A) fiber Group IV (C) fiber Short latency Slower onset Well localized Diffuse Short duration Long duration Less emotional Emotional (may be agonizing) Not blocked by morphine Blocked by morphine Neospinothalamic Tract Paleospinothalamic Tract Muscle Pain The contractions of an ischemic muscle cause pain even in a short time. Because contracting muscle produces metabolites such as Lewis’ P factor (substance P). The P factor may be potassium. When the blood supply is restored, the pain disappears. Pain produces muscle spasms. This mechanism resembles the flexion reflex of muscles due to pain. Acute abdomen is referred to as abdominal muscle spasms caused by the pain of inflamed organs in the abdominal or pelvic cavity (such as inflammation of the appendix). Clinically, substernal pain develops when the myocardium becomes ischemic during exertion. It is called angina pectoris. Resting, relieves angina by decreasing the heart load and O2 requirement. The 1st neurons of acute and chronic pain fibers are in the spinal ganglion. Afferents enter the spinal cord through the dorsal root and come to the dorsal horn. In the dorsal horn, they synapse with their second-order neurons. Some of the axons of 2. neuron produce reflex activity at the same side (ipsilateral). Some pass to the other side of the spinal cord and ascend to the upper brain areas. Transmission of pain fibers to the CNS Pain Induced Withdrawal Reflex When we touch our limb to a painful object, we pull it back by flexion reflex. Likewise, in the lesion of internal organs, abdominal flexors contract and produce spasms (Acute abdomen). Visceral Pain Visceral pain (internal organs’ pain) can be associated with autonomic symptoms like nausea, sweating, or changes in blood pressure. There are no proprioceptors, temperature, or touch receptors in the internal organs, so we cannot feel their location. Pain receptors in the internal organs are less and distributed sparsely. Pain and the other sensory fibers from visceral structures are carried to the CNS via sympathetic and parasympathetic afferent pathways. After a tissue damage on the skin or deeper areas, inflammatory factors like bradykinin, potassium, histamine, acids, leukotrienes, prostaglandins, nitric oxide (NO) and calcitonin gene-related peptides (CGRP) can increase sensitivity which causes pain in the inflammatory area. The acute pain fiber’s neurotransmitter is glutamate, and the chronic pain fiber’s neurotransmitter is substance P. Referred Pain Mostly the internal organs’ pain is referred the somatic structures of the body. The reason is the brain doesn’t know about the internal organs because there are no proprioceptors, touch and thermal receptors in the internal organs. There are two hypotheses to explain this mechanism; During embryonic development, some of the internal and somatic organs arise from the same segments of the neural tube and obtain their sensory nerves from these segments. 1- The visceral pain fibers enter the spinal cord and make synapses on the same second neurons (mutual) with the fibers coming from the somatic areas. Then, both visceral and somatic fibers ascend to the brain. However, because the brain does not recognize internal organs, it assumes that the pain sensation is coming from the somatic area. 2- Maybe there are separate 2. order neurons for visceral and somatic pain fibers. But the relationship between them also activates the somatic 2. order neurons. Again, the brain assumes that the pain is coming from the somatic area of the body. The most painful areas of the body Periosteum of the bones Membranes around and in the brain (meninges, falx cerebri, tentorium cerebelli) The walls of the great arteries Lung and liver capsule Skin and muscles In contrast, brain, lung, and liver parenchymal tissues are insensitive to pain. Cold and Heat-Sensitive Pain Receptors Cold sensation is mostly carried to the upper systems in the acute pain pathways by A-δ fibers. Heat is carried together with the chronic pain pathways, by the C fibers Extreme heat > 45ºC and extreme cold < 10ºC activate pain receptors Recently, it was recognized that some vanillins (vanilya), and capsaicin (found in the red pepper) also cause pain. The potentials that act on vanilloid receptors are called “transient receptor potentials, TRP”, there are TRPV1, TRPV2.…TRP8 type receptors. TRP causes to open some ion channels, such as Na+, Ca2+, Mg2+ Normal (moderate) cold receptors are sensitive to cold between 10-24oC and are called as cold-and menthol-sensitive receptors (CMR1) or (TRPM8).Temperatures below 10οC, towards minus degrees initially cause pain and then have an anesthetized effect. Analgesic Substances Opiate Peptides The most prominent analgesic substance for pain is morphine. Fortunately, the body can produce its own morphine-like substances and try to suppress the pain. Endogenous morphine-like substances are opioid peptides Opiates are enkephalins, endorphins, and dynorphins. They can be referred to as leu-enkephalin, met-enkephalin, dynorphin and endorphin. Analgesia-related Enkephalinergic neurons are found in three areas 1- Enkephalin is released from the immune cells in the inflammatory (injured) area. 2- They are released from the enkephalinergic neurons in the dorsal horn to suppress pain impulses coming from peripheral afferents (gate-control system). 3- They are found around the periventricular area and periaqueductal gray matter. The periventricular area and periaqueductal gray matter in the brainstem are the centers of emotions such as pain, grief, agony, misery and soreness, distress, heartache, and desperation (punishment center). Excessive pain or emotional shock causes the release of enkephalin from the vicinity cells to compensate for the agony. Cannabinoids in Pain Relief Cannabinoids are psychoactive compounds found in marijuana. At low (pharmacological) doses, cannabis can cause calmness and relaxation, reduction of pain, increase of appetite, but also decrease of shortterm memory. But it can be addictive. In high doses, it causes personality changes, hallucinations, and even delirium. Recently, some forms of cannabis have been approved for limited medical use primarily to treat nausea and stimulate appetite, in some cancer patients. There are also endogenous cannabinoids called anandamide (arachidonic acid derivative) in the brain. They produce stress-induced analgesia Pain Suppression in the Brain and Spinal Cord Putting one’s hand into water at 45oC will produce pain in everybody However, Reaction to this pain occurs differently, although the pain threshold is the same for all humans. Some people can use the suppression system of their brain but, others activate the central excitation system (CGRP) of their brain. To endure pain and other sensory impulses depends on the use of the brain’s suppressor effects. There are some sensory descending pathways from the brain to the spinal cord. These pathways may inhibit the pain fibers entering the spinal cord to some extent by causing presynaptic inhibition. Sensitization of the pain by the effects of CNS (CGRP) Gate-control mechanism in pain inhibition (1) Descending Analgesic Systems When there is unbearable pain or severe emotional shock, the brain’s opiate system is activated. The axons of the enkephalinergic neurons in the brainstem activate the serotonergic neurons in the Nucleus Raphe Magnus in the brainstem. The axons of the serotonergic neurons descend to the spinal cord and activate the enkephalinergic neurons in the dorsal horn (in the Substantia Gelatinosa of Rolando). The pain-carrying fibers are presynaptically inhibited by these enkephalinergic axons to some extent. Thus, the pain impulses are inhibited before they reach the upper levels of the brain where they would be perceived. This is the ‘gate-control mechanism of pain inhibition’ (Corticofugal Pathway). Enkephalin and endorphin systems can also be activated by the brain cortex*. *People who have self-control (willpower) can manage his/her pain to some extent. Presynaptic Inhibition; is the reduction of presynaptic and postsynaptic depolarization by the effect of a GABAergic neuron that makes an axoaxonal connection to the presynaptic end. In the Gate-Control Mechanism of pain; the enkephalinergic neurons in the dorsal horn (substantia gelatinosa) activate the GABAergic interneurons. These in turn, by presynaptic inhibition, decreases the pain fibers depolarization. Thus, calcium entering the presynaptic cell decreases and the neurotransmitter release also decreases. So, the postsynaptic neuron (the 2ndorder neuron of the pain) cannot be activated. Defense System Developed by the Brain in Physical and Emotional Trauma We see that although the periventricular and the periaqueductal areas in the brainstem and hypothalamus are concerned with punishment, fear, rage, and terror sensations, the stimulation of some neurons here can also initiate a compensatory mechanism to prevent the subject from dying due to pain shock. Sometimes, the pain or emotional shock is so severe that the excess release of endorphins, and enkephalins together with the release of corticosteroids can shut down the mind and lead to unconsciousness as is seen during big accidents, disasters, or war wounding. Pain inhibition by touching, pressure, rubbing, electrical stimulation, acupuncture applications GATE CONTROL SYSTEM Another physiological method of pain relief is to rub, shake, or electrically stimulate the painful part of the body. Doing so may activate the touch or proprioceptive pathways (Dorsal Column-Medial Lemniscal System). These pathways, give some collaterals and activate inhibitory interneurons, which can create lateral inhibition and cause pain relief. It is thought that pain relief by acupuncture depends on this method. Pain Pathways and Pathophysilogy Somatic Sensations are transmitted to the upper brain areas by way of ; Dorsal column-medial lemniscal system (proprioceptive, fine touch, fine pressure vibration)) Anterolateral System (pain, thermal, crude touch, and crude pressure) - pain and thermal senses by lateral spinothalamic pathway - crude touch, pressure, and unimportant senses by anterior (ventral) spinothalamic pathways Spinothalamic Pathway of Chronic Pain Chronic pain fibers coming from the deep tissues or visceral organs, follow the acute pain pathways. But; Most chronic pain fibers relay on the brainstem (reticular formation) and activate RAS. Then, project to the centrolateral nucleus of the thalamus for 3. neuron From the thalamus, they end in their 4. neuron in the postcentral gyrus of the cortex. PET and fMRI studies in humans indicate that chronic pain activates the primary and secondary somatosensory cortex, the cingulate gyrus, amygdala, frontal lob, and the insular cortex on the side opposite of the stimulus. Thus, these pathways mediate the affective component (suffering, agony) of pain Visceral sensation travels along the same pathways as the somatic sensation or with the cranial nerves and after relaying on limbic areas (especially to the hypothalamus) they intermix in the sensory cortex. Acute and Chronic Pain fibers along the lateral spinothalamic tract NOT: some pain fibers after synapsing with the 2. order neurons, produce flexion reflex at the same side (ipsilateral) This data is not shown in this figure The sensation of the face is perceived by Trigeminal Nerve (5. Cr) The trigeminal nerve (the fifth cranial nerve) is responsible for facial sensation and motor functions such as biting and chewing. It has 3 main branches: 1- Ophthalmic nerve (sensory) 2- Maxillary nerve (sensory) 3- Mandibular nerve (motor + sensory) The ophthalmic and maxillary nerves are purely sensory, whereas the mandibular nerve supplies motor as well as sensory (or "cutaneous") functions. The 7.Cr nerve : The facial nerve has motor function of the face, but some axons get senses from the tongue and salivary glands CLINIC Damage to pain pathways produces a deficit in pain and temperature discrimination and may also have abnormal, painful sensations called dysesthesia. In some inflammatory diseases of pain fibers, any stimulus that would generally cause only minor pain produces an exaggerated response called hyperalgesia,and an innocuous stimulus such as touch which causes pain, is called allodynia. Neuropathic pain Neuropathic pain is characterized by a burning, tingling, stabbing, or electric shock-like quality. Frequently it includes burning or coldness, pins and needles (tingling) numbness, and itching. It is mainly seen in diabetic patients. It does not even respond to non-steroid anti-inflammatory drugs (NSAIDs). In some neuropathic pain lesions ‘reflex sympathetic dystrophy, RSD’ can be seen. Trigeminal Neuralgia (tic douloureux) - excruciating (dayanılmaz), episodic pain in the area supplied by the trigeminal nerve, - intense pain makes the patient grimace (tic) - antiepileptic drug (phenytoin, carbamazepine) can be used, or, - surgical treatment is effective Thalamic Pain Syndrome (Dejerine–Roussy syndrome) It occurs as a result of a lesion in the thalamus (ischemia, bleeding (hemorrhage, tumor), It occurs in the relevant areas and related sensations. It starts with numbness, tingling, and dysesthesia. It progresses with a burning sensation, hyperalgesia, and allodynia Patients report that any stimulus that does not cause pain, even a sound or taste causes severe pain. Symptoms are unilateral, depending on the affected areas of the thalamus. It can even impair vision and balance. Low of Projection in Sensory System Phantom Pain Phantom Feeling (or phantom pain); is a sensation coming from the amputated organs or tissues. Its mechanism is not known very well. However, it is supposed that it might depend on the sensory coding of the previous pain sensation in the sensory cortex due to neural plasticity. That engram can be activated by any stimulus, coming from vicinity areas of the body. Pain-based learning occurs in the spinal cord posterior horns, thalamus, or sensory cortex by Neural Plasticity mechanisms. Syringomyelia is a chronic progressive disease in which longitudinal cavities (syrinx) form in the cervical region of the spinal cord. This characteristically results in the weakening of the muscles in the hands and bilateral loss of pain and temperature sensation. Syringomyelia initial symptom - bilateral loss of pain and temperature sensation and paralysis. Herpes zoster: shingles Chickenpox virus causes this infection. It is taken by the peripheral nerves and carried to the spinal ganglia (by retrograde transport). When the virus infects nerves (namely, the dorsal root ganglia), it remains for years in the body to be activated whenever the immune system of the body weakens. It can then be reactivated to cause shingles with blisters over the distribution area of the affected nerve accompanied by often intense pain and itching. SENSES Doç. Dr. Güldal INAL GULTEKIN 2 The eyes & sight The E Y E 3 Photoreceptor White light stimulates specific receptors, and creates and image on the retina by changing the molecular structure of vision pigments thus creating an nervous impulse which is transmitted via the N. Opticus to the optic centers in the center. 1. Depth receptor Estimating the distance or the size of an object, or the speed of one object, in respect to another object. 1. Functional anatomy 4 Eye muscles set 5 Refractor power Reciprocal contraction: one side relax while the other contracts Refraction * ışığın kırılması 6 The bending of light is called refraction The eye has two structures, the cornea and the lens, that bends light Their surface have curvatures , they are not staigth lke glass The lens is flexible, which provides the ability to the optics system of the eye to increase or decrease its convexity. Human Eye – cross section 7 Provides nutrition for the lens cells The eye has 2 structures that are crucial for its refractive ability : 1. Cornea 2. Lens Lens and the lens system 8 It is composed of ≅1000 layers of cells, That loose their nucleus and organelles during development And become absolutely transparent But lacks DNA, protein synthesizing machinery / thus cannot repair nor regenerate With its ability to refract light, it helps in the formation of the image on the retina The image is formed up-side down on the retina We learn to accept the upside down image as normal 9 Infrared light (invisible) 10 Humans and most mammals can’t see infrared lights, due to LACK of receptors, However, snakes have infrared receptors, and use them to pick up on the infrared lights that is released from the prey through radiation as it Photon energy is high thermal heat. It destroys receptors Photon energy is low, and can’t create the necessary chemical change in the vision pigments Lens özellikleri 11 Light coming from 6 m is accepted as parallel converge Odak noktası diverge Focal length 12 Parallel rays that strike a convex lens are bent then light rays converge at a 'focal point' The distance between the lens and the focal point is called the 'focal distance’ Odak noktası Fokal uzunluk Odak uzaklığı 13 The acuity in respect to light 14 Light : Will have parallel rays when it’s coming from a far ( ≥ 6 m – 20 feet ) distance But it will be oblique when coming from a near object, (a cell phone, a book) The object has to be reflected on the retina Fro this the eye’s OPTIC SYSTEM will have to adapt to the changes in the light ACCOMODASYON Accommodation 15 The ability to adjust the strength of the lens is known as accommodation The strenght of the lens depends on its shape The shape of the lens is controlled by the ciliary muscles Far sight Near sight Accomodation 16 Accomodasyon 17 The cilliary muscles are attached to the lens by the Lig. suspensorium Cilliary muscles are contracted (parasympathetic stimulus) – suspensory lig. Become loose the lens becomes more spherical due to its elastic properties (dışbükeyliği/convexity increase) this is accommodation for for the vision of near objects Cilliary muscle relaxed / resting state (sympathetic stimulus) – suspensory lig. Become tight the lens flattens the refractive power decreases accommodation for far objects 18 19 Sight problems 20 Normal view Myopia Hhyperopia Near sighted 21 Myopia Corrected Far sighted Presbyopia 22 With increasing age (40+) the lens looses its flexibility, near objects become blurry, the lens can’t accommodate thus can’t increase convexity, the objects appear behind the retina Refractive power is increased with convex lens Control of the papillae diameter 23 Parasympathetic Sympathetic The pupillae is stimulated The pupillae gets smaller Miosis Radial muscles of the iris get stimulated The dilatation of the pupillae Midriasis The pupillar ligth reflex 24 25 The fovea centralis on the macula 26 Visual acuity on the retina Retina – contains melanin pigments 27 Light path Melanin inhibits the reflection of light from one retinal point to the other Nerve cells and receptors of the retina 28 29 Circuitry of the Retina Retina receptors 30 ROD CON Receptor chemicals & action potential 31 Rods – rhodopsin (black & white sight – [B/W]) Cons – cone pigment or color pigment The chemical contain Vitamin A in their structure Avitaminosis A disrupts sight: severe conditions lead to night blindness Light energy Changes the chemical structure of Rhodopsin Creating an electrical stimulation in the receptor An impulse in generated from the retina N.Opticus carries the impulse to the centre 32 Receptor hyperpolarization and Vit A 33 w/ light the rod get hyperpolarized Na+ channels close in the outer segment Na+K+ ATPase is active in the inner segment Hence the rod (receptor) loses Na+ ion Light adaptation : cGMP activation decrease Channels close hyperpolarization (-70-80 mV) HYPERPOLARIZATION: receptor gets negative This creates an nerve impulse Most of the chemicals (retinal) turn into Vit A Dark adaptation: Na+ channels open Depolarization (-40 mV) Vit A amount decreases Vit A is in the structure of photochemicals Directional sensitivity and retinal receptor density 34 Adaption of the receptors 35 Adaptation to light OR dark Adaptation of rods for B / W sight takes longer and is stronger then cone adaptation Adaptation goes on for minutes to an hour Color adaptation stops at one point Adaptation process initiates very fast, Most of the adaptation will take place before the first minute has passed, But will keep increasing 36 Color view 37 Ishihara tests 38 http://fizyoloji.ege.edu.tr/index.php/lisansustu/23-sinir-pratik 39 Sinirsel ileti 40 41 Hearing İÇ KULAK 42 Çekiç Örs Üzengi Duyma organı = corti organı 43 Cochlea 44 45 46 47 48 49 50 Basilar membrane 51 52 Vibration of the basilar membrane 53 High frequency sound is a high pitched voice Low frequency sound is bass 54 Primary and secondary auditory corteces Iç kulak – vestibüler sistem 55 İşitme Doç. Dr. GÜldal İNAL GULTEKİN 56 57 Vestibular organ (denge) 58 1. 2. 3. Denge için 3 adet komponent: 3 semi-sirkülar kanal (horizontal-anteriorposterior) Utrikül Sakkule Macula + stataconia kristalleri: receptörleri Utrikül deki makula kafanın pozisyonu yatay (horizontal) düzlemde algılar Ayakta kafanın orientasyonu sağlanır Sakkulusdaki makula kafa pozisyonu dikey (vertikal) düzelme algılar Yatarken kafanın pozisyonu Üzerinlerindeki kristller ise calcium carbonate (CaCO3) tan oluşmultur Semi-circular kanallarda: crista ampullaris’leri içeri. Uazaydaki 3 boyut teşkil edilmiştir Doç. Dr. GÜldal İNAL GULTEKİN Denge resptörleri 59 Doç. Dr. GÜldal İNAL GULTEKİN R Le Parfume 60 Sense of smell Sent pathways 61 1. 2. Old pathways: primitive scent perception area is not a learned sent , it is associated with digestion Less old pathways: especially in coordination with the hippocampus (limbic system) are the routes in which the sent gives life or death decision. There are learned events This route is the only one that bypasses the thalamus Likes and disgusting odors It can even cause vomiting 3. New pathways: the conscious analysis of sent. Passes by the thalamus, sent impulses to the orbitofrontal lobe Taste bundles 62 Sweet Salty Saur Bitter Umami 63 GASTROINTESTINAL PHYSIOLOGY 1 Prof.Dr. Haluk KELEŞTİMUR Istanbul Okan University Medical School Department of Physiology For Contact Tlf: 5054120697 [email protected] 1st Floor Room Number: 139 General Features of Gastrointestinal System The digestive system consists of the digestive tract plus the accessory digestive organs Transit times of nutrients in digestive organs The Functions of the Gastrointestinal System The features of digestive canal The pH of the stomach contents falls as low as 2 as a result of gastric secretion of hydrochloric acid (HCl), yet in the body fluids the range of pH compatible with life is 6.8 to 8.0. The digestive enzymes that hydrolyze the protein in food could also destroy the body tissues that produce them. Therefore, once these enzymes are synthesized in inactive form, they are not activated until they reach the lumen, where they actually attack the food outside the body (that is, within the lumen), thereby protecting the body tissues against self-digestion. In the lower part of the intestine exist quadrillions of living microorganisms that are normally harmless and even beneficial, yet if these same microorganisms enter the body proper (as may happen with a ruptured appendix), they may be extremely harmful or even lethal. Foodstuffs are complex foreign particles that would be attacked by the immune system if they were in contact with the body proper. However, the foodstuffs are digested within the lumen into absorbable units such as glucose, amino acids, and fatty acids that are indistinguishable from these simple energy rich molecules already present in the body. Motility Types in Gastrointestinal Tract Peristalsis For the peristaltic contraction, behind the bolus (orad) circular muscle contracts and longitudinal muscle relaxes; in front of the bolus (caudad), circular muscle relaxes and longitudinal muscle contracts Peristalsis moves the chyme in the caudad direction. Segmentation contractions mix the chyme Energy-rich nutrients and hydrolysis Fluid intake and output in GI tract Gastrointestinal tract is separated by sphincters (cont’d) The upper esophageal sphincter separates the pharynx and the upper esophagus; The lower esophageal sphincter separates the esophagus and the stomach; The pyloric sphincter separates the stomach and the duodenum; The ileocecal sphincter separates the ileum and the cecum; The internal and external anal sphincters maintain fecal continence. (cont’d) At rest, the sphincters maintain a positive pressure that is higher than the pressures in the adjacent organs; thus at rest, both anterograde (forward) and retrograde (backward) flow is prevented. For example, at rest, the positive pressure of the lower esophageal sphincter prevents the gastric contents from refluxing into the esophagus. For gastrointestinal tract contents to move through the sphincter, it must relax and transiently lower its pressure. Changes in sphincter pressure are coordinated with contractions of the smooth muscle of the adjacent organs via reflexes (e.g., the swallowing reflex). Layers of the digestive tract wall Control of digestive system activities Slow waves of the gastrointestinal tract superimposed by action potentials and contraction The Phases of slow waves Phase 0: Resting membrane potential; outward potassium current. Phase 1: Rising phase (depolarization); activation of voltage-gated calcium channels Phase 2: Activation of voltage-gated potassium channels. Phase 3: Plaeau phase; balance of inward calcium current and outward potassium current. Phase 4: Falling phase (repolarization); inactivation of voltage-gated calcium channels and activation of calcium-gated potassium channels. Electrical slow waves with similar waveforms occur at different frequencies in the stomach, small intestine, and colon Electrical slow waves in the circular muscle occur at 3 waves/min in the human antrum, 11 to 12 waves/min in the duodenum, and 2 to 13 waves/min in the colon. Action potentials are not always associated with slow waves (A) No action potentials appear at the crests of the slow waves and the muscle contractions associated with each slow wave are small. (B) Muscle action potentials appear as sharp upward–downward deflections at the crests of the slow waves. Large-amplitude muscle contractions are associated with each slow wave when action potentials are present. Arrangement of Neurons of Enteric Nervous System The submucosal plexus (Meissner plexus) lies between the submucosa and the circular muscle. The myenteric plexus (Aurback plexus) lies between the circular muscle and the longitudinal muscle. The intrinsic nervous system of the gastrointestinal tract The motor neuron pool of the ENS consists of both excitatory and inhibitory neurons Neurotransmitters and Neuromodulators in the Enteric Nervous System The extrinsic nervous system of the gastrointestinal tract Parasympathetic system innervating GI tract Parasympathetic innervation is supplied by the vagus nerve (cranial nerve X) and the pelvic nerve. The vagus nerve innervates the upper gastrointestinal tract including the striated muscle of the upper third of the esophagus, the wall of the stomach, the small intestine, the ascending colon, and a portion of the transverse colon. The pelvic nerve innervates the lower gastrointestinal tract including the walls of the transverse, descending, and sigmoid colons. Sympathetic system innervating GI tract Four sympathetic ganglia serve the gastrointestinal tract: celiac, superior mesenteric, inferior mesenteric, and hypogastric. Postganglionic nerve fibers, which are adrenergic (i.e., release norepinephrine), leave these sympathetic ganglia and synapse on ganglia in the myenteric and submucosal plexuses, or they directly innervate smooth muscle, endocrine, or secretory cells. GASTROINTESTINAL REGULATORY SUBSTANCES Gastrointestinal Hormones Gastrin Protein in the stomach stimulates the release of gastrin, 2. It acts in multiple ways to increase secretion of HCl and pepsinogen, two substances of primary importance in initiating 1. 3. 4. 5. digestion of the protein that promoted their secretion. It enhances gastric motility, stimulates ileal motility, relaxes the ileocecal sphincter, and induces mass movements in the colon—all functions aimed at keeping the contents moving through the tract on arrival of a new meal. It also is trophic to both the stomach mucosa and the small-intestine mucosa, helping maintain a well-developed, functionally viable digestive tract lining. Gastrin secretion is inhibited by an accumulation of acid in the stomach (negative feedback effect) and by the presence in the duodenal lumen of acid and other constituents that necessitate a delay in gastric secretion. Cholecystokinin 1. 2. 3. 4. 5. As chyme empties from the stomach, fat and other nutrients enter the duodenum. These nutrients—especially fat and, to a lesser extent, protein products—cause the release of CCK. It inhibits gastric motility and secretion, thereby allowing adequate time for the nutrients already in the duodenum to be digested and absorbed. It stimulates the pancreatic acinar cells to increase secretion of pancreatic enzymes, which continue the digestion of these nutrients in the duodenal lumen (this action is especially important for fat digestion because pancreatic lipase is the only enzyme that digests fat). It causes contraction of the gallbladder and relaxation of the sphincter of Oddi so that bile is emptied into the duodenum. CCK is an important regulator of food intake. It plays a key role in satiety, the sensation of having had enough to eat. Secretin 1. 2. 3. 4. 5. As the stomach empties into the duodenum, the presence of acid in the duodenum stimulates the release of secretin. It inhibits gastric emptying to prevent further acid from entering the duodenum until the acid already present is neutralized. It inhibits gastric secretion to reduce the amount of acid being produced. It stimulates the pancreatic duct cells to produce a large volume of aqueous NaHCO3 secretion, which is emptied into the duodenum to neutralize the acid. Neutralization of the acidic chyme in the duodenum helps prevent damage to the duodenal walls and provides a suitable environment for optimal functioning of the pancreatic digestive enzymes, which are inhibited by acid. Secretin and CCK are both trophic to the exocrine pancreas. GIP (gastric inhibitory peptide, glucose-dependent insulinotropic peptide) 1. 2. 3. This hormone was originally named gastric inhibitory peptide (GIP) for its presumed role as an enterogastrone. It was believed to inhibit gastric motility and secretion, similar to secretin and CCK. Its contribution in this regard is now considered minimal. Instead, this hormone stimulates insulin release by the pancreas, so it is now called glucose-dependent insulinotropic peptide. Stimulated by the presence of a meal, especially glucose, in the digestive tract, GIP initiates the release of insulin in anticipation of absorption of the meal, in a feedforward fashion. Insulin is especially important in promoting the uptake and storage of glucose. Candidate Hormones Motilin is secreted from the upper duodenum during fasting states. Motilin is believed to increase gastrointestinal motility and, specifically, to initiate the interdigestive myoelectric complexes that occur at 90minute intervals. Pancreatic polypeptide is secreted by the pancreas in response to ingestion of carbohydrates, proteins, or lipids. Pancreatic polypeptide inhibits pancreatic secretion of HCO3 − and enzymes, although its physiologic role is uncertain. Enteroglucagon is released from intestinal cells in response to a decrease in blood glucose concentration. It then directs the liver to increase glycogenolysis and gluconeogenesis. (cont.) Glucagon-like peptide-1 (GLP-1) is produced from the selective cleavage of proglucagon. It is synthesized and secreted by the L cells of the small intestine. Like GIP, GLP-1 is classified as an incretin, because it binds to receptors on the pancreatic β cells and stimulates insulin secretion. In complementary actions, it also inhibits glucagon secretion, increases the sensitivity of pancreatic β cells to secretagogues such as glucose, decreases gastric emptying, and inhibits appetite. For these reasons, analogues of GLP-1 have been considered as possible treatments for type II diabetes mellitus. Paracrines Somatostatin is secreted by D cells (both endocrine and paracrine) of the gastrointestinal mucosa in response to decreased luminal pH. In turn, somatostatin inhibits secretion of the other gastrointestinal hormones and inhibits gastric H+ secretion. In addition to these paracrine functions in the gastrointestinal tract, somatostatin is secreted by the hypothalamus and by the delta (δ) cells of the endocrine pancreas. Histamine is secreted by endocrine-type cells of the gastrointestinal mucosa, particularly in the H+ -secreting region of the stomach. Histamine, along with gastrin and ACh, stimulates H+ secretion by the gastric parietal cells. Neurocrines Neurocrines are synthesized in cell bodies of gastrointestinal neurons. An action potential in the neuron causes release of the neurocrine, which diffuses across the synapse and interacts with receptors on the postsynaptic cell. The neurocrines include nonpeptides such as ACh and norepinephrine and peptides such as VIP, GRP, the enkephalins, neuropeptide Y, and substance P. The best-known neurocrines are ACh (released from cholinergic neurons) and norepinephrine (released from adrenergic neurons). The other neurocrines are released from postganglionic noncholinergic parasympathetic neurons (also called peptidergic neurons). GASTROINTESTINAL MOTILITY Chewing and Swallowing Oral Cavity The Functions of Chewing 1. 2. 3. 4. 5. The primary role of chewing is to break down foodstuffs for subsequent digestion. It mixes food with saliva, lubricating it to facilitate swallowing. It reduces the size of food particles, which facilitates swallowing (although the size of the swallowed particles has no effect on the digestive process). It mixes ingested carbohydrates with salivary amylase to begin carbohydrate digestion. It exposes food to the taste buds. Taste bud stimulation not only gives rise to the pleasurable sensation of taste, but also, in feedforward fashion, reflexly increases salivary, gastric, pancreatic and bile secretion to prepare for the arrival of food. The main motor and sensory brainstem nuclei associated with cranial nerves The cranial V motor nucleus contains alfa-motoneurons supplying jawclosing (e.g., masseter) and jaw-opening (e.g., anterior digastric) muscles. The cranial nerve VII nucleus has alfa-motoneurons supplying the muscles of facial expression (e.g., orbicularis oris). The main motor and sensory brainstem nuclei associated with cranial nerves The nucleus ambiguus (IX, X, XI) contains alfa- motoneurons supplying muscles of the palate, pharynx and larynx. The crainal nerve XII nucleus has alfa-motoneurons supplying intrinsic and extrinsic muscles (e.g., genioglossus) of the tongue. Diagram showing the main connections of the orofacial sensorymotor system Mastication or chewing depends on a brainstem center comprising a central neural pattern generator, the chewing center. Chewing has both voluntary and involuntary components. The involuntary component involves reflexes initiated by food in the mouth. Sensory information is relayed from mechanoreceptors in the mouth to the brain stem, which orchestrates a reflex oscillatory pattern of activity to the muscles involved in chewing. Voluntary chewing can override involuntary or reflex chewing at any time. The sensory and motor components of chewing reflex Comparison of orofacial motor system and spinal motor system The trigeminal mesencephalic nucleus is the only site in the body where primary cell bodies are located within the CNS. Many orofacial muscles lack muscle spindles and also Golgi tendon organs. A gamma efferent activation is thus absent due to the lack of muscle spindles in many muscles. The weakness of reciprocal innervation due to the lack of proprioceptors is compensated for by the powerful regulatory influences provided by afferent impulses from facial skin, mucosa, TMJ and teeth. Integrative pathways and processes exist to allow the bilateral activity of orofacial muscles. Requirements of Alimentary Swallow The Neural Control of Phryngeal Phase of Swallowing The Muscles Related to Swallowing and Their Innervations Different Swallow Patterns Some of the various muscles involved in swallowing are “obligate” swallow muscles, meaning they are always active in swallowing; examples are some of the tongue muscles as well as the palatal, pharyngeal, laryngeal, and esophageal muscles. Others are “facultative” swallow muscles in that they show a variable participation in swallowing. The facultative muscles are the facial and jaw muscles, and they may be especially sensitive to alterations in the oral environment and to maturational changes. Therefore, their participation can vary depending, for example, on the consistency or volume of a foodstuff being swallowed, or whether the subject is an infant or adult since there can be age-related differences in the involvement during swallowing of facial muscles (particularly noticeable in the infant) versus jaw muscles (noticeable in the adult). The infantile (visceral or “tooth-apart”) pattern of swallowing, as opposed to the adult (somatic or “tooth-together”) pattern, is often characterized by a pronounced tongue thrust, and retention of this pattern is thought by some clinicians to be of etiologic significance in certain malocclusions such as anterior open bite. However, toothapart swallows are quite normal in adults when a liquid or soft food bolus is being ingested, viz., the teeth may or may not come into occlusion during a normal adult swallow; a major determining factor is the consistency of the bolus. Esophagus Structures of the Upper Gastrointestinal Tract The pharynx, upper esophageal sphincter, and upper third of the esophagus are composed of striated muscle. The middle third of the esophagus contains striated and smooth muscles. The lower third of the esophagus and lower esophageal sphincter are composed of smooth muscle. The propulsive and protective effects of oesophagus and associated sphincters Schematic drawing showing the three major divisions of the stomach The functions of the stomach The stomach’s most important function is to store ingested food until it can be emptied into the small intestine at a rate appropriate for optimal digestion and absorption. The stomach secretes hydrochloric acid (HCl) and enzymes that begin protein digestion. Through the stomach’s mixing movements, the ingested food is pulverized and mixed with gastric secretions to produce a thick liquid mixture known as chyme. Gastric motility has the four aspects Filling Storage Mixing Emptying Gastric filling involves receptive relaxation. The interior of the stomach is thrown into deep folds. During a meal, the folds get smaller and nearly flatten out as the stomach relaxes slightly with each mouthful. This vagally mediated response, called receptive relaxation, allows the stomach to accommodate the meal with little change in intragastric pressure. When empty, the stomach has a volume of about 50 mL, but it can expand up to 20-fold to a capacity of about 1 liter (1000 mL) during a meal. If more than a liter of food is consumed, however, the stomach becomes overdistended, intragastric pressure rises, and the person experiences discomfort. Receptive Relaxation Mechanism of Receptive Relaxation Gastric storage takes place in the body of the stomach A group of pacemaker cells (interstitial cells of Cajal) located in the upper fundus region of the stomach generate slow-wave potentials (Basic electrical rhythm, BER) that sweep down the length of the stomach toward the pyloric sphincter at a rate of three per minute. Once initiated, a peristaltic wave spreads over the fundus and body to the antrum and pyloric sphincter. Because the muscle layers are thin in the fundus and body, the peristaltic contractions in this region are weak. When the waves reach the antrum, they become stronger and more vigorous because the muscle there is thicker. Gastric emptying and mixing Gastric emptying 1. 2. 3. 4. A peristaltic contraction originates in the upper fundus and sweeps down toward the pyloric sphincter. The contraction becomes more vigorous as it reaches the thick-muscled antrum. The strong antral peristaltic contraction propels the chyme forward. A small portion of chyme is pushed through the partially open sphincter into the duodenum. The stronger the antral contraction, the more chyme is emptied with each contractile wave. Gastric mixing 5. 6. When the peristaltic contraction reaches the pyloric sphincter, the sphincter is tightly closed and no further emptying takes place. When chyme that was being propelled forward hits the closed sphincter, it is tossed back into the antrum. Mixing of chyme is accomplished as chyme is propelled forward and tossed back into the antrum with each peristaltic contraction, a process called retropulsion. Factors Regulating Gastric Motility and Emptying There are three types of contractions in the small intestine Segmentation Peristalsis Migrating motility complex (MMC) Segmentation (cont’d) Segmentation consists of ringlike contractions along the length of the small intestine. Within a matter of seconds, the contracted segments relax and the previously relaxed areas contract. These oscillating contractions thoroughly mix the chyme within the small-intestine lumen. Segmentation is slight or absent between meals but becomes vigorous immediately after a meal. The duodenum starts to segment primarily in response to local distension caused by the presence of chyme. Segmentation of the empty ileum, in contrast, is brought about by gastrin secreted in response to the presence of chyme in the stomach, a mechanism known as the gastroileal reflex. Peristalsis The Migrating motor complex (MMC) (MMC) 1. Phase I: A long period lasting about 40 to 60 minutes of relative quiet with very few contractions 2. Phase II: A 20- to 30-minute period with some peristaltic contractions, with the time varying between contractions 3. Phase III: The shortest phase, where intense peristaltic contractions begin in the upper stomach and propagate (mi grate) through to the end of the small intestine. The contractions rhythmically repeat for 5 to 10 minutes. During this period, the pyloric sphincter relaxes and opens completely. (cont’d) During periods of short fasting, when most of the meal has been absorbed, the stomach and small intestine exhibit a unique motor activity. Intestinal segmentation contractions cease and are replaced by the migrating motility complex (MMC), or “intestinal housekeeper” activity. The motor activity of the MMC is thought to sweep any remnants of the preceding meal plus mucosal debris and bacteria forward toward the colon, just like a good “intestinal housekeeper.” The MMC is regulated between meals by the hormone motilin, which is secreted during the unfed state by endocrine cells of the small-intestine mucosa. When the next meal arrives, the MMC ceases and the motor activity associated with a meal takes over. Motilin release is inhibited by feeding. The Large Intestine The large intestine is primarily a drying and storage organ The colon normally receives about 500 mL of chyme from the small intestine each day. Because most digestion and absorption have been accomplished in the small intestine, the contents delivered to the colon consist of indigestible food residues (such as cellulose), unabsorbed biliary components, and the remaining fluid. The colon extracts more H2O and salt, drying and compacting the contents to form a firm mass known as feces for elimination from the body. The primary function of the large intestine is to store feces before defecation. Cellulose and other indigestible substances in the diet provide bulk and help maintain regular bowel movements by contributing to the volume of the colonic contents. Large Intestinal Motility (Haustral contractions) Segmentation contractions occur in the cecum and proximal colon. As in the small intestine, these contractions function to mix the contents of the large intestine. In the large intestine, the contractions are associated with characteristic saclike segments called haustra. Haustral contractions slowly shuffle the colonic contents back and forth. These movements are nonpropulsive; they slowly shuffle the contents in a back-and-forth mixing movement that exposes the colonic contents to the absorptive mucosa. Haustral contractions are largely controlled by locally mediated reflexes involving the intrinsic plexuses. Large Intestinal Motility (Mass movements) Mass movements occur in the colon and function to move the contents of the large intestine over long distances, such as from the transverse colon to the sigmoid colon. These massive contractions drive the colonic contents into the distal part of the large intestine, where material is stored until defecation occurs. Mass movements occur anywhere from 1 to 3 times per day. Water absorption occurs in the distal colon, making the fecal contents of the large intestine semisolid and increasingly difficult to move. Gastrocolic and gastroileal reflexes When food enters the stomach, mass movements are triggered in the colon primarily by the gastrocolic reflex, which is mediated from the stomach to the colon by gastrin and by parasympathetic innervation. In many people, this reflex is most evident after the first meal of the day and is often followed by the urge to defecate. Thus, when a new meal enters the digestive tract, reflexes are initiated to move the existing contents farther along the tract to make way for the incoming food. The gastroileal reflex moves the remaining smallintestine contents into the large intestine, and the gastrocolic reflex pushes the colonic contents into the rectum, triggering the defecation reflex. Defecation reflex As the rectum fills with feces, the smooth muscle wall of the rectum contracts and the internal anal sphincter relaxes in the rectosphincteric reflex. When it is appropriate, the external anal sphincter is relaxed voluntarily, the smooth muscle of the rectum contracts to create pressure, and feces are forced out through the anal canal. The intraabdominal pressure created for defecation can be increased by a Valsalva maneuver (expiring against a closed glottis). Gastrontestinal Physiology 2 Prof.Dr. Haluk KELEŞTİMUR Istanbul Okan University Medical School Department of Physiology Salivary Secretion Major Secretions into the GI Tract Regulation of Gastrointestinal Secretions Major Salivary Glands The epithelial cells of the salivary glands are arranged in secretory endpiece (acinus) connected to the oral mucosa by a system of ducts The acinus and canal system of the salivary glands The general features of the salivary glands The composition of salivary secretion in the different flow rate (a) and representation of the two-stage model of salivary secretion (b) Saliva is hypotonic to plasma at all flow rates. [HCO3-] in saliva exceeds that in plasma except at very low flow rates. The primary secretion is produced in the acinar cell. The concentration of electrolytes in plasma is similar to that in the primary secretion, but is modified as it passes through ducts that absorb Na+ and Cl- and secrete K+ and HCO3-. The duct cells are not permeable to H2O. The reflex control of salivary secretion Regulation of salivary secretion by the autonomic nervous system Control of salivary secretion by nerves Model for acinar ion transport mechanisms involved in salivary fluid formation (cont’d) A. B. C. Fluid secretion is an active process, dependent upon the active transport of electrolytes by the secretory cells. The parasympathetic innervation provides the main stimulus for fluid and electrolyte secretion. Acetylcholine shows its effect via binding to G-protein coupled muscarinic receptors with IP3-Ca2+ second messenger pathway. The increase in cytoplasmic Ca2+ opens Cl- channels in the luminal membrane and K+ channels in the basolateral membrane of the secretory cells. Intracellular Cl- enters the lumen, drawing extracellular Na+ ions through the tight junctions to balance the electrochemical gradient. The resulting osmotic gradient pulls water into the lumen, both through the tight junctions and via water channels (aquaporins) in the secretory cell membranes. The secretion of proteins and glycoproteins occurs by the process of exocytosis. Binding of norepinephrine released from sympathetic nerve terminals to G-protein coupled β-adrenergic receptors activates cAMP second messenger, leading to exocytosis in serous cells. During active fluid secretion, intracellular ionic and osmotic balance is maintained by Na+/K+/2Cl- cotransporters, Na+/H+ exchangers, and Na+/K+-ATPase in the basolateral membrane. With strong stimulation that results in high salivary flow rates, HCO3- , which may be formed intracellularly by carbonic anhydrase, enter the lumen via the Cl− channels. The transient intracellular acidification caused by the efflux of HCO3- is recovered by up-regulated activity of the basolateral Na+/H+ exchanger, which can use the Na+ gradient established by the Na+/K+ ATPase to export protons. Sources of salivary biomarkers Salivary fluid is primarily derived from salivary gland secretion. Most of the protein content of saliva comes from the salivary proteins that are synthesized and secreted by salivary acinar cells. Saliva in the mouth also contains epithelial cells shed from the mucosal surfaces, blood cells (neutrophils) from gingivae and oral microorganisms made up of a rich mix of bacterial species and candida. Small amounts of blood and tissue fluid proteins enter saliva mainly from the gingivae as gingival crevicular fluid (GCF) content. The composition of whole saliva The Features of Salive The amount produced at any given time depends on several factors, including autonomic nervous system activity, body hydration, the time of day, and drug use. Secretion from the major glands also follows a circadian pattern, with the greatest (unstimulated) output between noon and 6 p.m., and the least output between midnight and 6 a.m. Although producing only a small volume, the minor glands secrete almost continuously, and therefore have an important role in moistening, lubricating, and protecting the oral mucosa and teeth during sleep. A side effect of many commonly prescribed medications is dry mouth, caused by central or peripheral inhibition of saliva secretion. The flow rate of unstimulated whole saliva varies between 0.2 and 0.5 mL/minute. With maximal stimulation, the whole saliva flow rate can exceed 7 mL/minute. The volume of saliva in the mouth at any given time varies between about 0.6 and 1.2 mL, following and just prior to swallowing. This volume is spread over the entire oral cavity, creating a salivary film 0.07 to 0.1 mm thick coating the mucosa and teeth. The functions of saliva The Protective Functions of Saliva Clearance: The dilution and clearance of food debris, microbial acids, and other products is facilitated by the constant flow of the salivary film across the teeth and mucosa, which moves at different rates with 0.8 mm per minute along the facial surfaces of the maxillary incisors, whereas under stimulated conditions 300 mm per minute along the lingual surfaces of the mandibular incisors. Lubrication: The negatively charged mucins and glycoproteins, with abundant bound water, help to lubricate the mucosa and teeth and allow tissues to slide past one another during chewing, swallowing, and speaking. Thermal/Chemical Insulation: A coating of mucins on the oral surfaces helps to protect against thermal, chemical, and mechanical insults. Pellicle: Many salivary constituents, including mucins, acidic proline-rich proteins (PRPs), statherin, histatins, and cystatins, bind to the enamel surface, forming a salivary pellicle. These bound constituents create a reservoir of calcium and phosphate at the tooth surface that opposes demineralization and promotes remineralization of initial caries lesions, especially in the presence of F− ions. Toxin Binding: The basic PRPs and histatins bind tannins (common constituents of many plant-derived foods that may inhibit growth and have various toxic effects) and prevent their uptake by intestinal epithelial cells. Buffering Salivary HCO3− and carbonic anhydrase play important roles in counteracting the effects of microbial acids generated from sugars on the hydroxyapatite crystals of enamel and dentin. Salivary HCO3− levels increase as salivary flow increases, and in the presence of H+, carbonic anhydrase in saliva or bound to teeth in the salivary pellicle forms H2O and CO2, and the CO2 escapes into the air. Some buffering also is achieved by HPO42− and H2PO4− in saliva, as well as by cationic, histidine-rich proteins. Additionally, urea and ammonia formed by microbial metabolism help to neutralize acids. Antimicrobial Activity Saliva contains a number of proteins that function in regulating the oral microbial flora. Some of the proteins, such as lysozyme and peroxidase, are enzymes that attack microbial cell walls or generate products that inhibit microbial metabolism. Other proteins, such as mucins, salivary agglutinin (gp340), and the major salivary immunoglobulin, secretory immunoglobulin A (sIgA), bind to and aggregate microorganisms, preventing them from binding to oral tissues. SPLUNC (short palate lung nasal epithelium clone) family proteins, in addition to binding and inhibiting growth of certain bacteria, bind lipopolysaccharide (LPS) and prevent release of inflammatory mediators from macrophages. Some small peptides, such as defensins, cathelicidin LL-37, and the histatins, insert into and disrupt microbial cellular membranes, resulting in osmotic lysis or depletion of various metabolites. Lactoferrin binds iron, reducing its availability to microorganisms, and also disrupts cellular membranes. Secretory leukocyte protease inhibitor (SLPI) binds to and inhibits certain bacteria and exhibits anti-viral activity. Tissue Repair Growth factors present in saliva, such as epidermal growth factor, and other salivary proteins (e.g., trefoil factor family peptides, histatins) are believed to facilitate wound healing and tissue repair. These factors may induce cell proliferation and migration during wound healing and re-epithelialization in the oral cavity and the digestive tract. Trefoil proteins are thought to promote healing of ulcers in the oral cavity and digestive tract; they are produced by salivary mucous cells and also by keratinocytes of the oral epithelium. Digestion Saliva has an important role in eating and digestion. Saliva serves to facilitate mastication and swallowing of food and solubilizes many food constituents. During mastication, the water and mucins in saliva protect the oral tissues and help to create a food bolus suitable for swallowing. Saliva also contains several enzymes that initiate digestion of various dietary constituents. These include amylase, which hydrolyzes starches to maltose and limit dextrins, ribonuclease (RNAse) and deoxyribonuclease (DNAse). Lingual lipase, produced by the lingual serous glands and pharyngeal glands, initiates digestion of dietary triglycerides. Although digestion of these substances is achieved mainly by pancreatic and gastric enzymes, the salivary enzymes may play a significant role in cases of pancreatic insufficiency. Taste The solubilization of food makes possible the detection of taste substances by taste receptors in taste buds of the tongue. Clearance of taste substances by saliva permits detection of new taste stimuli. The salivary salt (NaCl) concentration is only one seventh of that in the plasma, which is important in perceiving salty taste.The taste receptors are adapted to the concentration of Na+ in saliva; the threshold for the salty taste of NaCl is greater and the supra-threshold intensity is less in the presence of saliva than distilled water. The absence of glucose in saliva at all is important in the formation of the feeling of sweetness. Some components of saliva may interact with taste substances and modify their effect. For example, HCO3− in saliva reacts with acid (H+), reducing its taste intensity. Basic PRPs bind to tannins, diminishing or altering their bitter taste or interfering with taste reception. Gastric Secretion Structure of a gastric oxyntic gland showing the various cell types lining the gland Secretory products of various gastric cells Functional anatomy of the stomach Mechanism of HCl secretion by gastric parietal cells 1. In intracellular fluid, carbon dioxide (CO2) produced from aerobic metabolism combines with H2O to form H2CO3, catalyzed by carbonic anhydrase. H2CO3 dissociates into H+ and HCO3−. The H+ is secreted with Cl− into the lumen of the stomach, and the HCO3 − is absorbed into the blood, as described in Steps 2 and 3, respectively. 2. At the apical membrane, H+ is secreted into the lumen of the stomach via the H+-K+ ATPase. The H+-K+ ATPase is a primary active process that transports H+ and K+ against their electrochemical gradients (uphill). H+-K+ ATPase is inhibited by the drug omeprazole, which is used in the treatment of ulcers to reduce H+ secretion. Cl− follows H+ into the lumen by diffusing through Cl− channels in the apical membrane. Mechanism of HCl secretion by gastric parietal cells 3. At the basolateral membrane, HCO3− is absorbed from the cell into the blood via a Cl− -HCO3− exchanger. The absorbed HCO3 − is responsible for the “alkaline tide” (high pH) that can be observed in gastric venous blood after a meal. Eventually this HCO3− will be secreted back into the gastrointestinal tract in pancreatic secretions. 4. In combination, the events occurring at the apical and basolateral membranes of gastric parietal cells result in net secretion of HCl and net absorption of HCO3−. Functions of HCl HCl activates the enzyme precursor pepsinogen to an active enzyme, pepsin, and provides an acid environment optimal for pepsin action. It aids in the breakdown of connective tissue and muscle fibers, reducing large food particles into smaller particles. It denatures protein—that is, it uncoils proteins from their highly folded final form, thus exposing more of the peptide bonds for enzymatic attack. Along with salivary lysozyme, HCl kills most of the microorganisms ingested with food, although some escape and then grow and multiply in the large intestine. Agents that stimulate and inhibit H+ secretion by gastric parietal cells Stimulation of gastric secretion Inhibition of gastric secretion Gastric mucosal barrier 1. 2. 3. 4. The luminal membranes of the gastric mucosal cells are impermeable to H+ so that HCI cannot penetrate into the cells. The cells are joined by tight junctions that prevent HCI from penetrating between them. A mucus coating over the gastric mucosa serves as a physical barrier to acid penetration. The HCO3 – -rich mucus also serves as a chemical barrier that neutralizes acid in the vicinity of the mucosa. Even when luminal pH is 2, the mucus pH is 7. Pepsinogen secretion Pepsinogen, the inactive precursor to pepsin, is secreted by chief cells and by mucous cells in the oxyntic glands. When the pH of gastric contents is lowered by H+ secretion from parietal cells, pepsinogen is converted to pepsin, beginning the process of protein digestion. Once formed, pepsin acts on other pepsinogen molecules to produce more pepsin, a mechanism called an autocatalytic process. In the cephalic and gastric phases of H+ secretion, vagal stimulation is the most important stimulus for pepsinogen secretion. H+ also triggers local reflexes, which stimulate the chief cells to secrete pepsinogen. Intrinsic Factor Secretion Intrinsic factor, a mucoprotein, is the “other” secretory product of the parietal cells. Intrinsic factor is required for absorption of vitamin B12 in the ileum, and its absence causes pernicious anemia. Intrinsic factor is the only essential secretion of the stomach. Thus following gastrectomy (removal of the stomach), patients must receive injections of vitamin B12 to bypass the absorption defect caused by the loss of gastric intrinsic factor. Pancreatic secretion The pancreas is a mixture of exocrine and endocrine tissue. The smaller endocrine part consists of isolated islands of endocrine tissue, the islets of Langerhans, which are dispersed throughout the pancreas. The most important hormones secreted by the islet cells are insulin and glucagon. The exocrine pancreas secretes digestive enzymes and an alkaline fluid. (1) pancreatic enzymes actively secreted by the acinar cells that form the acini and (2) an aqueous alkaline solution actively secreted by the duct cells that line the pancreatic ducts. The aqueous (watery) alkaline component is rich in sodium bicarbonate (NaHCO3). Composition of pancreatic juice The activation of pancreatic juice enzymes When trypsinogen is secreted into the duodenal lumen, it is activated to its active enzyme form, trypsin, by enteropeptidase (formerly known as enterokinase), an enzyme embedded in the luminal membrane of the cells that line the duodenal mucosa. Trypsin then autocatalytically activates more trypsinogen. Like pepsinogen, trypsinogen must remain inactive within the pancreas to prevent this proteolytic enzyme from digesting the proteins of the cells in which it is formed. As further protection, the pancreas also produces a chemical known as trypsin inhibitor, which blocks trypsin’s actions if spontaneous activation of trypsinogen inadvertently occurs within the pancreas. Chymotrypsinogen and procarboxypeptidase are converted by trypsin to their active forms, chymotrypsin and carboxypeptidase, respectively, within the duodenal lumen. Thus, once enteropeptidase has activated some of the trypsin, trypsin carries out the rest of the activation process Mechanism of pancreatic secretion Hormonal control of pancreatic exocrine secretion The factors regulating pancreas secretion following a meal The liver and bile secretion 1. 2. 3. 4. 5. 6. 7. 8. 9. 10. Secretion of bile salts, which aid fat digestion and absorption. This is the only liver function directly related to digestion. The first role of bile salts is to emulsify dietary lipids. Without emulsification, dietary lipids would coalesce into large “blobs,” with relatively little surface area for digestion. The second role of bile salts is to form micelles with the products of lipid digestion including monoglycerides, lysolecithin, and fatty acids. Metabolic processing of the major categories of nutrients (carbohydrates, proteins, and lipids) after their absorption from the digestive tract. Detoxifying or degrading body wastes and hormones, as well as drugs and other foreign compounds Synthesizing plasma proteins, including those needed for blood clotting, those that transport steroid and thyroid hormones and cholesterol in the blood, and angiotensinogen important in the salt conserving renin–angiotensin–aldosterone system Storing glycogen, fats, iron, copper, and many vitamins Activating vitamin D, which the liver does in conjunction with the kidneys Secreting the hormones thrombopoietin (stimulates platelet production), hepcidin (inhibits iron uptake from the intestine), and insulin-like growth factor-I (stimulates growth) Producing acute phase proteins important in inflammation Excreting cholesterol and bilirubin, the latter being a breakdown product derived from the destruction of worn-out red blood cells Removing bacteria and worn-out red blood cells Enterohepatic circulation of bile salts 1. Bile contains several organic constituents, namely, bile salts, cholesterol, lecithin, and bilirubin. Even though bile does not contain any digestive enzymes, it is important for the digestion and absorption of fats, primarily through the activity of bile salts. 2. Bile salts are derivatives of cholesterol. They are actively secreted into the bile and eventually enter the duodenum, along with the other biliary constituents. Following their participation in fat digestion and absorption, most bile salts are reabsorbed into the blood by special active-transport mechanisms located only in the terminal ileum. From here, bile salts are returned by the hepatic portal system to the liver, which resecretes them into the bile. This recycling of bile salts (and some of the other biliary constituents) between the small intestine and the liver is called the enterohepatic circulation. 3. The total amount of bile salts in the body averages about 3 to 4 g, yet 3 to 15 g of bile salts may be emptied into the duodenum in a single meal. On average, bile salts cycle between the liver and the small intestine twice during the digestion of a typical meal. Usually, only about 5% of the secreted bile escapes into the feces daily. These lost bile salts are replaced by new bile salts synthesized by the liver; thus, the size of the pool of bile salts is kept constant. DIGESTION AND ABSORPTION Digestive Processes for the Three Major Categories of Nutrients Carbohydrate digestion Carbohydrate absorption Protein digestion Protein absorption 1. 2. 3. 4. Dietary fat in the form of large fat globules composed of triglycerides is emulsified by the detergent action of bile salts into a suspension of smaller fat droplets. Lipase hydrolyzes the triglycerides into monoglycerides and free fatty acids. These water-insoluble products are carried to the luminal surface of the smallintestine epithelial cells within water-soluble micelles, which are formed by bile salts and other bile constituents. When a micelle approaches the absorptive epithelial surface, the monoglycerides and fatty acids leave the micelle and passively diffuse through the lipid bilayer of the luminal membranes. 5. The monoglycerides and free fatty acids are resynthesized into triglycerides inside the epithelial cells. 6. These triglycerides aggregate and are coated with a layer of lipoprotein from the endoplasmic reticulum to form water-soluble chylomicrons. 7. Chylomicrons are extruded through the basal membrane of the cells by exocytosis. 8. Chylomicrons enter the lymphatic vessels, the central lacteals. The Regulation of Hunger and Satiety Prof. Dr. Haluk KELEŞTİMUR Istanbul Okan University Medical School Department of Physiology Descriptions of hunger and satiety Hunger is a feeling caused by lack of food. It is an important signal, saying to the body about the need for food intake and energy from it. Satiety is the feeling of fullness and the suppression of hunger for a period of time after a meal. Appetite is the desire for food. Prevalence of adult overweight & obesity (%) - World Obesity A sample of Complications of Obesity The hypothalamus is the brain’s appetite control center. Structure of Hypothalamus Hypothalamic Nuclei and Its Functions The centres of hunger and satiety are located in the hypothalamus Dorsomedial hypothalamus (DMH) provides the relationship between satiety and hunger centers. Neuropeptides Involved in Control of Food Intake and Metabolism Peripheral Peptides Involved in Control of Food Intake and Metabolism Orexinergic and anorexinergic neurotransmitters and hormones that influence hunger and satiety centers in the hypothalamus Metabolic Pathways During the Fasting Phase and Absorptive Phase of Metabolism Absorptive phase. During the absorptive phase the activity of parasympathetic nervous system (Rest and digest) increases, and the activity of the sympathetic nervous system falls. We receive glucose, amino acids, and fats from the intestines. The blood level of insülin is high, which permits all cells to metabolize glucose. In addition, the liver and the muscles convert glucose to glycogen, which replenishes the short-term reservoir. Excess carbohydrates and amino acids are converted to fats, and fats are placed into the long-term reservoir in the adipose tissue. Effects of Insulin and Glucagon on Glucose and Glycogen (cont’d) Fasting phase. During the fasting phase the activity of the parasympathetic nervous system falls, and the activity of the sympathetic nervous system (Fight or flight) increases. In response, the level of insulin decreases, and the levels of glucagon and the adrenal catecholamines rise. These events cause liver glycogen to be converted to glucose and triglycerides to be broken down into glycerol and fatty acids. In the absence of insulin, only the central nervous system can use the glucose that is available in the blood; the rest of the body lives on fatty acids. Glycerol is converted to glucose by the liver, and the glucose is metabolized by the brain. Effects of Insulin and Glucagon on Glucose and Glycogen Neural circuitries concerned with the control of drinking and eating The connections of the melanin concentrating hormone (MCH) neurons and orexin neurons of the lateral hypothalamus Melanin-concentrating hormone (MCH). A peptide neurotransmitter found in a system of lateral hypothalamic neurons that stimulate appetite and reduce metabolic rate. Orexin. A peptide neurotransmitter found in a system of lateral hypothalamic neurons that stimulate appetite and reduce metabolic rate. The connections of the NPY neurons of the arcuate nucleus. Activity of MCH and orexin neurons of the lateral hypothalamus increases food intake and decreases metabolic rate. These neurons are activated by NPY/AGRP-secreting neurons of the arcuate nucleus, which are sensitive to ghrelin and which receive excitatory input from NPY neurons in the medulla that are sensitive to glucoprivation. The NPY/AGRP neurons of the arcuate nucleus also project to the paraventricular nucleus, which plays a role in control of insulin secretion and metabolism. The endocannabinoids stimulate appetite by increasing the release of MCH and orexin. Control of energy balance by two types of neurons of the arcuate nuclei Pro-opiomelanocortin (POMC) neurons that release alphamelanocyte–stimulating hormone (α-MSH) and cocaine- and amphetamine-regulated transcript (CART), decreasing food intake and increasing energy expenditure. Neurons that produce agoutirelated protein (AGRP) and neuropeptide Y (NPY), increasing food intake and reducing energy expenditure. (cont’d) α-MSH released by POMC neurons stimulates melanocortin receptors (MCR-3 and MCR-4) in the paraventricular nuclei (PVN), which then activate neuronal pathways that project to the nucleus tractus solitarius (NTS) and increase sympathetic activity and energy expenditure. AGRP acts as an antagonist of MCR-4. Insulin, leptin, and cholecystokinin (CCK) are hormones that inhibit AGRP-NPY neurons and stimulate adjacent POMC-CART neurons, thereby reducing food intake. Ghrelin, a hormone secreted from the stomach, activates AGRP-NPY neurons and stimulates food intake. First-order neurons in arcuate nucleus of hypothalamus The arcuate nucleus has two subsets of neurons that function in an opposing manner. One subset releases neuropeptide Y (NPY), and the other releases melanocortins derived from proopiomelanocortin (POMC), a precursor molecule that can be cleaved in different ways to produce several hormone products. NPY, one of the most potent appetite stimulators ever found, leads to increased food intake, thus promoting weight gain. Melanocortins, most notably amelanocyte stimulating hormone (a-MSH) from the hypothalamus, suppress appetite, thus leading to reduced food intake and weight loss. Second-order neurons in the hypothalamus Two hypothalamic areas are richly supplied by axons from the NPY and a-MSH neurons of the arcuate nucleus. These second-order neuronal areas involved in energy balance and food intake are the lateral hypothalamic area (LHA) and paraventricular nucleus (PVN). The LHA produces orexins, which are potent stimulators of food intake. NPY stimulates and melanocortins inhibit the release of appetite-enhancing orexins. By contrast, the PVN releases chemical messengers, for example, corticotropin-releasing hormone (CRH), that decrease appetite and food intake. Melanocortins stimulate and NPY inhibits the release of these appetite-suppressing chemicals. Short-Term Eating Behavior Two blood-borne peptides secreted by the digestive tract that are important in regulating how often and how much we eat in a given day are ghrelin and peptide YY3-36 (PYY336), which signify hunger and fullness, respectively. Ghrelin, the so-called hunger hormone, is a potent appetite stimulator produced by the stomach. Ghrelin stimulates appetite by activating the hypothalamic NPYsecreting neurons. PYY3-36 is a counterpart of ghrelin. The secretion of PYY3-36, which is produced by the small and large intestines, is at its lowest level before a meal but rises during meals and signals satiety. This peptide acts by inhibiting the appetite stimulating NPY secreting neurons in the arcuate nucleus. Long-Term Maintenance of Energy Balance The arcuate nucleus is the major site for leptin (an adipokine) action. Leptin suppresses appetite, thus decreasing food consumption and promoting weight loss, by inhibiting hypothalamic output of appetitestimulating NPY and stimulating output of appetite suppressing melanocortins. The leptin signal is generally considered the dominant factor responsible for the long-term matching of food intake to energy expenditure so that total body energy content remains balanced and body weight remains constant. Long-Term Maintenance of Energy Balance Another blood-borne signal besides leptin that plays an important role in long-term control of body weight is insulin. Insulin, a hormone secreted by the pancreas in response to a rise in the concentration of glucose and other nutrients in the blood following a meal, stimulates cellular uptake, use, and storage of these nutrients. Thus, the increase in insulin secretion that accompanies nutrient abundance, use, and storage appropriately inhibits the NPY-secreting cells of the arcuate nucleus, thus suppressing further food intake. Satiety center in brain stem In addition to the key role the hypothalamus plays in maintaining energy balance, a satiety center in the brain stem known as the nucleus tractus solitarius (NTS) processes signals important in the feeling of being full and thus contributes to short-term control of meals. Not only does the NTS receive input from the higher hypothalamic neurons involved in energy homeostasis, but it also receives afferent inputs from the digestive tract (for example, afferent input indicating the extent of stomach distension) and elsewhere that signal satiety. Cholecystokinin as a Satiety Signal 1. CCK is one of the gastrointestinal hormones released from the duodenal mucosa during digestion of a meal and is an important satiety signal for regulating meal size. 2. CCK is secreted in response to the presence of nutrients in the small intestine. It contributes to the sense of being filled after a meal has been consumed but before it has been digested and absorbed. This explains why we stop eating before the ingested food is made available to meet the body’s energy needs. 3. Other related, more recently discovered gut peptides released in response to a meal that serve as satiety signals include glucagon like peptide 1 (GLP 1) and oxyntomodulin. Psychosocial and Environmental Influences Often our decision to eat or stop eating is not determined merely by whether we are hungry or full, respectively. Eating foods with an enjoyable taste, smell, and texture can increase appetite and food intake. Stress, anxiety, depression, and boredom have also been shown to alter feeding behavior in ways unrelated to energy needs. People often eat to satisfy psychological needs rather than to satisfy hunger. Furthermore, environmental influences, such as the amount of food available, play an important role in determining the extent of food intake. Thus, any comprehensive explanation of how food intake is controlled must take into account these voluntary eating acts that can reinforce or override the internal signals governing feeding behavior. The homeostatic control of food intake upon peripheric signals The reward system controlling non-homeostatic food intake Food intake is also influenced by non-homeostatic signals, inducing food intake for pleasure. The hedonic intake of food depends mainly on taste, odor, texture, and appearance. The reward system can lead to overeating and positive energy balance and induce obesity. The reward system involves the mesocorticolimbic pathway that includes dopaminergic neurons located in the VTA and their axons projecting to the striatum, including the Nac and to the prefrontal cortex (PFC). RESPIRATORY SYSTEM PULMONARY FUNCTION (Faculty of Dentistry and Health Science) Prof. Dr. Lamia Pınar Okan Üniversitesi Tıp Fakültesi Fizyoloji Anabilim Dalı The main functions of respiration are to provide oxygen to the tissues by inspiration (inhalation) and remove carbon dioxide from the body by expiration (exhalation) Gas exchange O2 CO2 3 Respiratory Physiology O2 External respiration: - transport of O2 from atmosphere cell’s mitochondria - transport of CO2: CO2 lung from the cell’s mitochondria atmosphere Internal respiration: heart Cell metabolism by using Oxygen and releasing Carbon dioxide +Water + ATP O2 CO2 tissues (Oxidative Phosphorylation) 4 The function of the respiratory system (in detail) Exchange of oxygen (O₂) and carbon dioxide (CO₂) between the air and the cells of the body. During inspiration, fresh air (O₂) is brought into the lungs through the respiratory passage (respiratory pathway) CO₂, as a waste product of tissue metabolism, is carried to the lungs and is expelled from the respiratory passage CO₂ and O₂ are carried by the capillaries to the alveoli of the lungs and exchanged in the alveoli Other functions of the respiratory system The lung also plays a role in: Provide a defensive mechanism to viruses, bacteria, and foreign particles. A primary barrier between the outside world and the inside of the body. The lung is also a metabolic organ that synthesizes and metabolizes numerous compounds. Circuitry of the Cardiovascular system The heart has right and left atriums and right and left ventricles. All the vessels coming from the body and lungs flow into the atriums, All the vessels carry the blood to the body, and lungs emerge from the ventricles As a rule; All the vessels that return to the heart are called veins. All the vessels that eject the blood from the heart to the body are called arteries. Veins and Arteries of the Cardio-Respiratory system Vena Cavae sup and inf carry venous blood (high amount of CO₂) from the organs, and flows into the right atrium then, the blood flows into the right ventricle (through the tricuspid valve). From the right ventricle, the dirty blood (having high CO₂) is pumped to the lungs by way of the pulmonary artery. Left and Right Sides of the Heart Oxygenated blood (having high O₂) in the lungs turns to the left atrium by way of the pulmonary veins. Then flows into the left ventricle. Left ventricle pumps blood to all organs of the body except the lungs. Left heart and the systemic arteries, capillaries, and veins constitute systemic circulation. The rate at which blood is pumped from either ventricle is called the cardiac output (CO). The blood flowing from the left atrium to the left ventricle passes through the mitral valve (bicuspid valve). When the left ventricle contracts (systole), blood is ejected into the aorta via the aortic semilunar valve and goes to the organs. When the right ventricle contracts (systole) blood is ejected through the pulmonary semilunar valve and goes to the lungs The pumping amount of blood from both ventricles are same and is called cardiac output (CO) CO= stroke volume x heart rate in 1 minute Stroke volume, is the volume of blood pumped from each ventricle per beat. CO= 70 ml x 70-80beats/per min CO (at resting)= 4900-5000ml= 5 L In the capillary beds of the lungs Oxygen (O₂) is transferred to the blood from the alveolus, and Carbondioxide (CO₂) is removed from the blood and transferred to the alveoli then, it is expelled out of the lungs. Thus, the blood leaving the lungs has more O₂ (clear blood) and less CO₂ The RESPIRATORY SYSTEM Respiratory Passage (Respiratory Airway) Structure and Function of Airways (Respiratory Passage) Respiratory Airways Nose, Larynx Trachea Right and left primary bronchus Bronchioles Terminal bronchioles FUNCTION: Filtration, Conduction, Moistening, and Heating of air Mucociliary cells trap the foreign particles and throw them out of the respiratory passage RESPIRATION (Gas Exchange) begins in the: Respiratory bronchioles, Ductus alveolaris and Alveoli (plural of alveolus) There are also macrophages in the alveoli to scavenge (remove) the bacteria and viruses The right primary bronchus is wider and vertical. For this reason, foreign bodies escaping into the respiratory passage are mostly stuck in this bronchus. alveoli Bronchial tree Ankara, Oct. 2006 Blood vessels 18 Capillaries of Pulmonary arteries carry dirty blood (deoxygenated) from de Right ventricle, Capillaries of Pulmonary veins carry the clean (oxygenated) blood from the alveoli. Respiratory mechanics depends on Boyle Marriot Law means that the pressure of a given quantity of gas varies inversely with its volume at a constant temperature. It means that, if the volume of the gas filled in an area increases, the pressure of the gas decreases, or if the volume of the gas decreases, the pressure of the gas increases. Lung Membranes (Pleuras) There are 2 membranes around the lungs. These are called Pleura. The inner Visceral pleura surrounds the lungs, The outer Parietal pleura surrounds the thoracic cavity. The 2 pleura are connected. Between the two membranes, there is negative pressure as they attract each other in opposite directions. Also, there is a very little amount of liquid that facilitates the sliding of the membranes over each other. In the resting state between the inspiration and expiration this negative pressure is about (- 4mmHg or, even -5mmHg). You can refer to it as water pressure (-4 or -5 H2O). During inspiration, intrapleural pressure becomes - 6mmHg or - 7mmHg If one or both of these membranes are punctured, air enters the intrapleural space, and the positive pressure puts pressure on the lung, deflating it. This is called Pneumothorax. Mechanics of breathing: Pleura intrapleural pressure 24 Mechanics of breathing: Inspiration begins with the contraction of the diaphragm and external intercostal muscles. This increases the negative pressure in the chest wall and causes the lungs to be expanded. Expiration occurs passively by elastic recoil (deflation) of the lungs. 26 The mechanics of breathing (continue) Auxiliary muscles of respiration During the forced inspiration (stronger than normal); Neck and Shoulder muscles (sternocleidomastoid and pectoralis) contract and Enlarges the thorax. During the forced expiration Abdominal, internal intercostal muscles contract and decrease thorax volume. exspiration inspiration 28 Muscles of Respiration The accessory muscles Exercise of inspiration are not involved during normal quiet breathing but may be called into play during exercise; during the inspiratory phase of coughing or sneezing; or in a pathologic state, such as asthma. Alveoli 300 millions of alveoli Total surface area 85 m2 Each alveolus has a very little amount of water in it. Surface tension occurs due to collapsing (shrivel, shrink) aim of the water molecules When a liquid interfaces with air, it tends to make its shape spherical. Such as seen in rain droplets. Thus, the very small amount of liquid in the alveoli, interfacing with air, tends to minimize its surface area (surface tension). This can cause difficulty to inflate the lungs. Fortunately, a substance secreted from the alveolar cells, called Surfactant prevents this surface tension Lung structure Alveoli: Function: Gas exchange Structure: Alveolar epithelial cells (type I) Surfactant (type II) Around alveoli, there is Capillary endothelium Ankara, Oct. 2006 33 ALVEOLUS ” Neonatal respiratory distress syndrome (RDS)” In the gestational period, the fetus’s lungs complete producing surfactant by 35. week, in the intrauterine life. If a baby is born before 26 weeks of the normal period, this premature infant (early born) will have a lack of surfactant and suffer respiratory distress syndrome. It is called Neonatal RDS. They exhibit atelectasis (lung collapse) and cannot inflate their lungs so the baby will suffer hypoxemia. TYPES of BREATHING Epnea: Normal breathing Apnea – Absence of breathing. e.g., Sleep apnea Dyspnea – Difficult or labored breathing Orthopnea - Changing of the breath due to positions (During congestive heart failure, asthma, and lung failure, Dyspnea mostly occurs when recumbent (lying), but can be relieved by sitting or standing. Dead Space Anatomic dead space is the normal volume of the conducting airways and approximately 150 ml. This volume of the pulmonary system does not participate in Gas Exchange. Physiologic dead space constitutes some of the nonfunctional alveoli due to some pathologies. It is defined as a volume of the lungs that does not participate in gas exchange. Differences between Pulmonary Ventilation and Alveolar Ventilation PULMONARY VENTILATION: the amount of air moved in and out of the lungs in one minute. We breathe about 12-20 times in one minute We get about 500mL air in each breath Pulmonary Ventilation = 500 x 12 = ~ 6000 mL/min (6 L/min) air ALVEOLAR (MINUTE) VENTILATION: the amount of air that moves in and out of the ALVEOLUS in one minute. We get 500 mL of air in one breath. 150 mL of it stays in the Anatomic Dead Space. In the alveoli 500 -150 =350 mL of air participate in gas Exchange. Alveolar minute ventilation = 350 x 12 = ~ 4200 mL/min (4,2 L/min) Alveolar ventilation alveolar ventilation = total ventilation minus ventilation of dead space The proportion of alveolar ventilation increases with increasing tidal volume decreases with increasing respiratory frequency an constant ventilation condition tidal frequency total dead alveolar of volume minute space ventilation breathing ventilation ventilation --------------------------------------------------------------------------------------------------shallow 150ml 40 6000ml 150 x 40 0ml normal 500ml 12 6000ml 150 x 12 4200ml deep 1000ml 6 6000ml 150 x 6 5100ml deep breaths = large alveolar ventilation = improvement of gas exchange 40 Physics of Gases: Gas diffusion - Diffusion requires a concentration gradient - Diffusion goes from higher to lower concentration (“downhill”) O2 O2 consumes oxygen 42 Partial Atmospheric Pressures of Air Atmospheric pressure of air: 760 mm Hg in sea level Oxygen makes up 21% of atm pressure - Partial Oxygen pressure (PO₂): 760 x 0.21 = 160 mmHg Nitrogen makes up 78% of atm pressure - Partial nitrogen pressure: 760 x 0.78 = 600 mmHg Carbon Dioxide makes up 0.04% of atm pressure - Partial CO2 pressure (PCO₂): 760 x 0.0004=0.3 mmHg Bronchodilatation depends on; Airway resistance, When bronchus diameter increases, airway resistance decreases Physiologic Factors that cause bronchodilation Sympathetic nervous system activation (β₂ adrenergic receptors) – Adrenaline – Increased CO2 pressure – Nitric oxide (NO) Normal lung Smokers die 10 years early A British long-term study indicates that smokers die 10 years earlier than non-smokers. However, it’s worth stopping smoking at any time. Smoker‘s lung Some results were surprising: Those who stopped smoking at the age of 30 lived as long as nonsmokers. Men, who stopped before age 40 lived only one year less than non-smokers. Institute of Plastination, Heidelberg, 2004 46 Lung Volume and Capacities (Static Evaluation) EVALUATION of LUNG’S VOLUME and CAPACITIES by SPIROMETRY Volumen Volumen Volume Pulmonary ventilation: TIDAL VOLUME per each Breathing time Tidal volüme: 500 mL in each breathing Breathing frequency: 12-20 times/min 49 Pulmonary Volumes 1-The tidal volume (VT) is the volume of air inspired or expired with each normal breath; it is about 500 ml in the average adult male. 2- The inspiratory reserve volume (IRV) is the extra volume of air that can be inspired over and above the normal tidal volume when the person inspires with full force; it is about 3000 ml 3- The expiratory reserve volume (ERV) is the maximum extra volume of air that can be achieved by forceful expiration after the end of a normal tidal expiration; It is about 1100 ml 4- The residual volume (RV) is the volume of air remaining in the lungs even after the most forceful expiration. It is about 1200 ml Pulmonary Capacities 1 1-The inspiratory capacity (IC) equals the tidal volume plus the inspiratory reserve volume (IC=TV+IRV). The person begins to inspire from a normal expiratory level and continues to inspire forcefully. It is about 3500 ml 2. The functional residual capacity (FRC) is the amount of air that remains in the lungs at the end of normal expiration. FRC = expiratory reserve volume + the resi