ID 2322 Materials & Production Week 2 PDF
Document Details
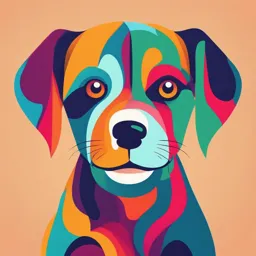
Uploaded by ObtainableChlorine1747
National University of Singapore
Tags
Summary
This document is from the National University of Singapore, discussing various materials used in engineering. It covers topics including metals, their types, structures, and properties. The document also explores the different properties of engineering materials, including thermal and electrical conductivity, malleability, and various applications.
Full Transcript
ID 2322 Materials & Production Week 2 national university of singapore DIVISION OF INDUSTRIAL DESIGN College of Design and Engineering BA(ID) - Level 2 SEMESTER 1 Module Leader - Kouo Wai Chiau...
ID 2322 Materials & Production Week 2 national university of singapore DIVISION OF INDUSTRIAL DESIGN College of Design and Engineering BA(ID) - Level 2 SEMESTER 1 Module Leader - Kouo Wai Chiau email : [email protected] Engineering Materials Types Basic Material Structure Comparison: common engineering materials New Advanced Materials TYPES OF ENGINEERING MATERIALS Engineering materials can be classified into 4 categories: Engineering materials Metals Polymers Ceramics Composites These materials are very different from one another in terms of their structures, properties and applications. TYPES OF ENGINEERING MATERIALS Engineering materials Metals Polymers Ceramics Composites METALS Crystalline in structure Opaque lustrous in nature good electrical conductivity good thermal conductivity Capable of changing their geometrical shapes permanently under external forces Relatively high strength, stiffness and ductility METALS Alloy: when two or more metals are combined together, an alloy is formed. Common examples of metals and alloys are: Copper Aluminium Gold Steels Brasses Bronzes, phosphor bronze. Bell metal (copper 77% + tin 23%) Sterling silver (silver 92.5% + copper 7.5%) Nichrome (nickel 80-85% + chromium 15-20%) Super alloys METALS Their applications are wide and varied. For instances, they are used for car bodies, tin cans, construction reinforcement, rails, engine blocks, conducting wires, cabinets, valves and etc. The Nature of Metals A metal can best be defined by the nature of the bonds between the atoms that make up the metal crystals. Metals can be defined as solids comprised of atoms held together by a matrix of electrons (Figure 1-8). STRUCTURE OF METALS When a metal is in its molten state, the atoms are arranged randomly but are held together by weak forces of attraction. When it solidifies, the atoms arrange themselves according to a regular 3-dimensional pattern with the density of packing as close as possible. This orderly arrangement of atoms is known as space lattice. STRUCTURE OF METALS Such a lattice is made up of series of points in space representing locations of atoms inter-connected by a network of imaginary lines. The smallest unit that made up the building block of the space lattice is called the unit cell or lattice cell or lattice structure. STRUCTURE OF METALS There are altogether 14 basic unit cells that can be identified. STRUCTURE OF METALS In metals, the common unit cells are body-centred cubic, face-centred cubic, body-centred tetragonal, body-centred orthorhombic and hexagonal close-packed. BODY-CENTRED CUBIC (BCC) Because of the closed packing of atoms, metals of BCC unit cell are normally of high melting point, high yield strength but low ductility. Metals such as tungsten, molybdenum, vanadium, crystallise into this form of lattice structure. FACE-CENTRED CUBIC (FCC) In a FCC lattice cell, the atoms arrange themselves with an atom in each corner of a cube, and an additional one atom at the centre of each face of the cube, Fig.3. Because of the more open-spaced arrangement of atoms, metals in this group tend to be ductile and easily deformed. Examples of metals with FCC arrangement are copper, aluminium, lead, nickel, silver, gold and iron. HEXAGONAL CLOSE-PACKED (HCP) The HCP lattice structure is found in many of the least common metals. Beryllium, zinc, titanium, magnesium and zirconium are examples of metals that crystallise into this structure. Deformation takes place readily on the 2 basal planes of the lattice structure. Because of the wide spacing between the 2 basal planes, metals with this form of lattice structure tend to have a low plasticity. In general, embrittlement arises readily upon deformation at room temperature (i.e. cold working). For metals, the electrons associated with each individual atom are free to move throughout the volume of the crystal. This is why metals are good conductors of electricity; current flow requires a flow of electrons. Other properties that distinguish metals from other materials are: their malleability (their ability to deform plastically), their opacity (light cannot pass through them), and their ability to be strengthened. When crystalline solids are subjected to loads, on the atomic scale, there is a tendency to pull the atoms apart. If the bonds between the atoms are very strong, there is a tendency to cleave(split) the crystals apart (Figure 1-9). In metals the inter-atomic bonds are such that rather than causing cleavage, loading can cause atomic slip. Since dislocations are atomic in size, they can usually only be seen and studied with the use of special microscopic and etching techniques. Where do dislocations come from? Dislocations can be produced by crystal mismatch in solidification. They can be introduced by external stresses such as plastic deformation (example: autofrettage gun barrel), they can occur by phase transformations that cause atomic mismatch, or they can be caused by the atomic mismatch effects of adding alloy elements. Dislocation interactions within a metal are a primary means by which metals are strengthened. When metals deform by dislocation motion, the more barriers the dislocations meet, the stronger the metal. Metals can be strengthened by solid solution strengthening. This term means that impurity atoms are added to a pure metal to make an alloy. If the atoms of the alloying element are significantly larger than the atoms of the host metal, there large atoms can impede(to hinder) the motion of dislocations and thus strengthen the metal (Figure 1-11a). Mechanical working strengthens metals by multiplication of dislocations. The dislocations interact with each other and with such things as grain boundaries, and thus movement of individual dislocations becomes difficult and the metal is strengthened (Figure 1-11b). Precipitation hardening is used to strengthen many nonferrous metals. By choosing a suitable alloying element, it is possible by heat treating techniques to get alloying elements to agglomerate within the metal lattice. The agglomerated (become collected into a mass) alloy element atoms create atomic mismatches and strains that serve as barriers to dislocation motion and thus strengthen the metal (Figure 1-11 c). The final and most industrially important strengthening mechanism in metals is quench hardening (Figure 1-11d). Quench hardening is a heat treating process used to induce atomic strains into a metal lattice. The strains are produced by quench- induced trapping of solute atoms into the lattice. The trapped atoms actually change the atomic spacing. The distorted lattice and the action of the quenched-in solute atoms impede dislocation motion and thus strengthen the metal. Types of Metals Metals Ferrous Nonferrous 4000 years ago human began to take iron as a usable metal. The earliest iron tools were made by chipping pieces of iron from meteorites containing metallic iron. In the 14th century, the technology was developed to melt and cast iron into a useful shape and form. Steel Products Today, steel is a very useful metal that our automobiles, tools, and buildings all rely on steel for their manufacture. Steel by definition is an alloy of iron and carbon, with the carbon being restricted within certain concentration limits. Though steel is so ordinary to us has been in large-scale commercial use for only about 150 years. Chemical Composition of steel Steel by definition is an alloy of iron and carbon. Pure Iron is when iron-carbon alloys have less than 0.005% carbon present at room temperature. Pure iron is soft, ductile and relatively weak. It is not normally used as an engineering material because of its low strength, but it is used for special applications, such as magnetic devices and enameling (to coat) steels. Chemical Composition of steel From the commercial standpoint, steels have a low carbon limit of approximately 0.06% carbon. Cast irons are iron-carbon alloys with more than 2% by weight of carbon. Above this carbon level, casting is about the only way that a useful shape can be made from the alloy, since the high carbon makes the iron alloy too brittle for rolling, forming, shearing, or other fabrication techniques. Thus, steels are alloys of iron and carbon with carbon limits between approximately 0.06% and 2.0%. Chemical Composition of steel Cast irons PURE IRON Too Hard & Brittle Too soft & weak STEEL 0.005% 0.06% Rolling, forming, shearing, 2.0% Carbon Carbon or other fabrication Carbon Use as techniques. magnetic devices and enameling (to coat) steels. Steel Steel production The entire ore-to-steel process is schematically illustrated as below. Schematic of a blast furnace Processing of refined steel into products Note: High-strength low-alloy steels (HSLA) Steel products Schematic of hot and cold finishing of steels Steel Terminology The selection of steels requires consultation on property information and supplier information on availability. The following is a tabulation of steel product terms and what they mean: Carbon steel: Steels with a carbon as the principal hardening agent. All other alloying elements are present in small percentages, with manganese being limited to 1.65% maximum, silicon to 0.60% maximum, copper to 0.60% maximum, and 0.05% maximum for sulfur and phosphorus. Solid solution strengthening Alloy steels: This term can refer to any steel that has significant additions of any element other than carbon, but in general usage alloy steels are steels with total alloy additions of less than about 5%. They are used primarily for structural applications. Galvanized Steel: Zinc-coated steel products. The zinc is applied by hot dipping. There are usually paint-adhesion problems with galvanized steels. Galvannealed Steel: Zinc-coated and heat-treated steel. The heat treatment given to galvannealed steels create an oxide layer that allows better paint adhesion. Different forms of Steel Products Sheet: Rolled steel primarily in the thickness range of 0.010 to 0.250 in. (0.25 to 6.4 mm thick and with a width of 24 in. (610 mm) or more. Bar: Hot- or cold-rolled rounds, squares, hexes, rectangles, and small shapes. Round bar can be as small as 0.25 in. (6.4 mm), flats can have a minimum thickness of 0.20 in. (5.0 mm), shapes having a maximum dimension less than 3 in. (76 mm). Coil: Rolled steel in the thickness range of sheet or strip. Flat wire: Small hot- or cold-rolled rectangles often made by cold-reducing rounds to rectangular shape. Wire: Hot- or cold-drawn coiled rounds in varying diameters, usually not exceeding 6.4 mm. Shapes: Hot-rolled I beams, channels, angles, wide-flange beams, and other structural shapes. At least one dimension of the cross section is greater than 76 mm. Tin plate: Cold-rolled steel with a usual thickness range of 0.1 to 0.4 mm. It may or may not be tin coated. Free machining: Steels with additions of sulfur, lead, selenium, or other elements in sufficient quantity that they machine easier than untreated grades. Drawing quality: Hot- or cold-rolled steel specially produced or selected to satisfy the elongation requirements of deep drawing operations. Merchant quality: Steels with an M suffix on the designation intended for nonstructural applications. A low-quality material. Pickling: Use of acids to remove oxides and scale on hot-worked steels. Stainless steels Stainless steels Stainless steels have a minimum of 10.5 % chromium as the principal alloying element. The four major categories of wrought stainless steel based on their metallurgical structure are: austenitic, ferritic, martensitic, precipitation hardening. Austenitic stainless steels Austenitic stainless steels are commonly used for processing chemicals and food and dairy products, as well as for shafts, pumps, fasteners, and piping in sea water equipment where corrosion resistance and toughness are primary requirements. Ferritic wrought alloys Ferritic wrought alloys are used for automotive exhaust systems and heat-transfer equipment for the chemical and petrochemical industries. These alloys are magnetic, with moderate toughness and corrosion resistance. Martensitic stainless steels Martensitic stainless steels are typically used for bearings, molds, cutlery, medical instruments, aircraft structural parts and turbine components. They are magnetic and can be hardened by heat treatment. These alloys are normally used where strength and/ or hardness is the primary concern, in a relatively mild corrosive environment. Precipitation-hardening stainless steels Precipitation-hardening stainless steels are used for aircraft components, high-temper springs, fasteners, and high-pressure pump parts. The precipitation-hardening process produces very high strength in a low-temperature heat treatment that does not significantly distort precision parts. These alloys are used where high strength, moderate corrosion resistance, and good fabricability are required. Tool steels Tool steels are metallurgically "clean," high-alloy steels that are used for tools and dies and for parts that require resistance to wear, stability during heat treatment, strength at high temperatures, or toughness. Tool steels are often specified for critical high- strength or wear-resistant applications. To develop their best properties, tool steels are always heat treated. Tool steels Shock-resisting tool steels (type S) are used for pneumatic tooling parts, chisels, punches, shear blades, bolts, and springs subjected to moderate heat in service. They are strong and tough, but not as wear resistant as other tool steels. Hot-work steels (type H) are used for high performance aircraft parts such as primary airframe structures, cargo-support lugs, catapult hooks, and elevon hinges. High-speed tool steels (type T/M) make good cutting tools because they resist softening and maintain a sharp cutting edge at high service temperatures. Type P mold steels are created specifically for plastic and die-cast tooling. POWDERED METALLURGY Powdered metallurgy (P/M) has provided new processes and new metal alloys that can significantly reduce weight while providing enhanced mechanical properties. P/M parts are used in sports products; electronic and office equipment components such as actuators, sprockets, levers, fasteners, bearings, impellers, cams, and gears; and for automotive engines, transmissions, and chassis, as well as off-road vehicles. POWDERED METALLURGY POWDERED METALLURGY Heated at high temperature Compacting pressure is between 80 MPa and 1600 MPa POWDERED METALLURGY Parts are formed by a compaction process and then sintered. They can then be forged in a second step for greater strength. Additional forming processes include injection molding, hot isostatic pressing, and cold isostatic pressing. In addition to conventional iron and steel alloys, the list of available powders includes new classes of tool steels, alloys of aluminum, copper, nickel, titanium and other nonferrous metals. Metal Injection Moulding Metal injection moulding (MIM) is a hybrid technology whereby it integrates the shaping capabilities of plastic injection moulding and materials flexibility of conventional powder metallurgy. MIM is preferred for mass manufacturing of small, intricate-geometry components of a variety of materials as it can achieve 95% to 98% of its wrought materials properties at a much reduced cost. See: http://www.douyeetech.com/home.htm Metal Injection Moulding Metal Injection Moulding Metal Pendant Watch Links ID2321_Metals Metal Injection Moulding 1453°C 1670°C 1287°C 1860°C 232°C 3400°C 328°C 660°C 1084°C 650°C 420°C 1063°C 961°C 1770°C Nonferrous Metals Many of the same manufacturing methods used with ferrous metals are also used to form, cut, and join nonferrous metals and their alloys. Low Melt Temperature Metals Aluminum is one of the most commonly used metals and is particularly important in industrial design. It has a high strength-to-weight ratio, good formability, and its own anticorrosion mechanism. When exposed to air, a hard microscopic oxide coating forms on the surface, sealing the metal. Aluminum and most aluminum alloys, available in commercial forms, can be easily formed, cut, joined, and finished. Aluminum is an efficient electrical conductor, is non sparking and nonmagnetic. It is available in two forms: as wrought products or as castings or ingots. Aluminum products Copper Copper is known for its ease of forming and joining, excellent electrical and thermal conductivity, attractive color, and excellent corrosion resistance. Copper (Cu) and its alloys have relatively low strength-to-weight ratios and low strengths at elevated temperatures, and unless stressed relieved, some alloys are susceptible to stress cracking. Copper Brass (Cu + Zinc, 930°C) and Bronze (Cu + Tin , 950°C) are available in many standard shapes and are used extensively in the plumbing industries and in fine art casting. Magnesium Magnesium is an important metal for product design because it is the lightest structural metal available. Typical gravity filled castings are aircraft engine components and wheels for racing and sports vehicles. Die-cast examples are chain-saw and power tool housings and attache case frames. Because of its light weight, magnesium is used extensively in the transportation and recreation industries. It has an excellent combination of low density and good mechanical strength, which gives it a high strength-to-weight ratio. Magnesium Magnesium alloys can absorb energy elastically and have moderate strength, which provides excellent dent resistance and a high dampening capacity. Magnesium Magnesium has good fatigue resistance and performs well in applications involving a large number of cycles. The metal is sensitive to stress concentrations, so partial design should avoid notches, sharp corners, and abrupt section changes. Zinc alloy Zinc alloy die-cast components are used in numerous products that range from automotive components, building hardware products such as electrical boxes, and industrial tool components to toys. Zinc has moderate strength and ductility. Because it has excellent corrosion resistance, it is used as a coating for steel, called galvanized steel. galvanized steel TYPES OF ENGINEERING MATERIALS Engineering materials Metals Polymers Ceramics Composites POLYMERS Long molecular chains Each chain being on the backbone of carbon atoms Light weight Low electrical conductivity Low thermal conductivity Generally resistant to atmospheric attack Inherent low strengths render unsuitability of polymers for high temperature applications Monomer structures of different polymers POLYMERS Common examples of polymers are polyamide, polyvinyl chloride (PVC), polystyrene, ABS, rubber and nylon. Typical applications include carrier bags, plumbing pipes, hoses, tyres and domestic items. POLYMERS Types of Polymers Polymers Thermoplastics Thermosets Composites Thermoplastics 1) The classification of thermoplastics 2) Plastics mixtures 3) Copolymers 4) Blends 5) Terpolymers 6) Additives 7) Factors affecting mechanical properties 8) Polymers product line creation and structure Thermoplastics can be classified into : Amorphous (noncrystalline) Crystalline Liquid crystalline polymers (LCP) Amorphous thermoplastics examples : polycarbonate, polystyrene, ABS (acrylonitrile-butadiene-styrene), SAN (styrene- acrylonitrile), and PVC (polyvinylchloride). Broad softening point Very good mechanical properties (strength, stiffness, impact, etc.) Dimensional stability Consistent, predictable shrinkage Intrinsically transparency possible Amorphous thermoplastics Summary: Amorphous Polymers Thermoplastics with extensive chain branching, large pendent groups, and low stereoregularity tend to favor an amorphous structure. Thermosetting polymers are amorphous because the cross- linking inhibits crystallization. Amorphous thermoplastics melt or liquefy over an extended temperature range. Thermoplastic and thermosetting amorphous polymers exhibit glass transition temperatures (Tg). The mechanical properties of amorphous polymers significantly degrade near the Tg. Thermosetting polymers do not melt but will degrade above the Tg. Crystalline thermoplastics They have polymer chains that are packed together in an organized way. Examples : acetal, nylon, polyethylene, polypropylene, and polyester. The organized regions in crystalline thermoplastics are joined by noncrystalline (amorphous) zones, and the structure is such that the materials are stronger and stiffer, though less impact resistant than completely noncrystalline materials. Crystalline thermoplastics Higher melting temperature Sharp melting point Resistance to some severe chemical environments Anisotropic (differential) shrinkage and warpage factors Very good electrical insulation properties Higher heat capabilities with reinforcement Amorphous/Crystalline Blends Tailored products to enhance specific, performance capabilities Characteristics dependent on blend proportions Generally provide a balance of performance selected for specific application needs. Summary: Semicrystalline Polymers Polymers with long, slender aliphatic chains and lower levels of chain branching tend to crystallize. Semicrystalline polymers have a defined melting point (Tm). Most crystalline polymers contain some degree of amorphous polymer. A Tg may be detected for the amorphous phase present in a crystalline polymer. The amorphous phase in a crystalline polymer can have profound effects on the polymer's mechanical properties. Liquid crystalline plastics (LCP's) LCP’s are polymers with highly ordered rod-like structures and posses high mechanical property values, good dimensional stability, good chemical resistance and are easy to process. The melting temperatures are similar to those of crystalline plastics. Unlike amorphous and crystalline plastics, liquid crystalline plastics retain significant order in the melt phase. As a result, they have the lowest shrinkage and warpage of the three types of thermoplastics. Mixtures characteristics of plastics materials can be changed by : 1) Mixing or combining different types of polymers 2) Adding nonplastics materials. Particulate fillers such as wood, flour, silica, sand, ceramic, carbon powder, tiny glass balls, and powdered metal are added to increase modulus and electrical conductivity, to improve resistance to heat or ultraviolet light and to reduce cost. 3) Plasticizers are added to decrease modulus and increase flexibility. 4) Other additives may be used to increase resistance to ultraviolet light and heat or to prevent oxidation. Polymers can be strengthen by adding fibers Reinforcing fibers of glass, carbon, or Aramid (aromatic polyamide fibers having high tensile strength, a range of moduli, good toughness, and stress-strain behavior similar to that of metals) are added to improve mechanical properties. Careful design and selection must be used to position the fibers so that they will provide the required strength where it is needed. The effect of glass-fiber reinforcement on the properties of nylon 6/6 Continuous fiber may be positioned carefully in either a thermoplastics or thermoset matrix to produce basic parts generally called composites. These products have the highest mechanical properties and cost of the reinforced plastics. Copolymers Copolymers embody two different monomers and may have properties that are completely different from those of the individual polymers (homopolymers) from which they are made. Types of copolymers Since a copolymer consists of at least two types of repeating units (not structural units), copolymers can be classified based on how these units are arranged along the chain. These include Random copolymer: -A-A-B-B-A-A-A-B-A-A-B-B-A-B-A-A-A- B-A-A-A-B-B- Alternating copolymer: -A-B-A-B-A-B-A-B-A-B-, or -(-A-B-)n- Block copolymer: -A-A-A-A-A-A-A-B-B-B-B-B-B-B-A-A-A-A- A-A-A-B-B-... Graft copolymer: -A-A-A-A-A-A-A-A-A-A-A-A-A-A-A-A-A-A- | | B-B-B-B B-B-B-B Where A=monomer of A chemistry B= monomer of B chemistry Blends Akin to copolymerization is the use of plastic blends and alloys. Blends and alloys are made by physically mixing two or more polymers (also called homopolymers). As alloying consists of pure mechanical blending of two or more different polymers, often with special additives to make them compatible. These "alloys" are compounded so as to retain the most desirable characteristics of each constituent, especially in impact strength and flame resistance. However, properties usually are intermediate between those of the constituent materials. Polymer Blend A-A-A-A-A-A-A-A-A B-B-B-B-B-B-B A-A-A B-B-B-B A-A-A-A-A-A-A-A-A-A-A-A B-B-B-B-B-B A-A-A-A-A-A-A-A-A-A-A Terpolymers In terpolymers-polymers with three different repeating units-individual components can also be tailored to offer a wide range of properties. An example is ABS, a terpolymer containing repeating units of acrylonitrile, butadiene, and styrene. Acrylonitrile Butadiene Styrene (ABS) ABS is a family of amorphous thermoplastics that are hard, tough and rigid. microscopic polybutadiene rubber particles Molecular Weight A polymer's molecular weight - the sum of the weights of individual atoms that make up a molecule-indicates the average length of the bulk polymer chains. Low-molecular weight polyethylene chains have backbones as small as 1000 carbon atoms long. Ultrahigh-molecular-weight polyethylene chains, can have a half-million carbon atoms along their length. Molecular Weight Many plastics such as polycarbonate, for instance, are available in a variety of chain lengths, or different molecular weight grades. These resins can also be classified by an indirect viscosity value, rather than molecular weight. Within a resin family, higher-molecular weight grades have higher viscosities. Molecular Weight Selecting the correct molecular weight for injection- molding applications generally involves a balance between filling ease and material performance. If an application has thin-walled sections, a lower- molecularweight / lower-viscosity grade offers better flow. For normal wall thicknesses, these resins also offer faster mold-cycle times and fewer molded-in stresses. The stiffer-flowing, high- molecular-weight resins offer the ultimate material performance, because they are tougher and more resistant to chemical and environmental attack. Additives Fillers and modifiers are additives used to produce specific changes in the properties and/or characteristics of resins. A large number of common additives are used in the plastics industry, including glass and mineral reinforcements, flame retardants, release agents and UV stabilizers. Many Plastics’ materials are available with one or more of these additives included, in order to address the specific needs of an application or environment. Flame Retardants These additives are used when a plastic part must pass some type of industry or agency standard. Flame retardants are carefully chosen for each material to avoid potential inherent incompatibilities. The purpose of anti-flash (Nomex) gear is to provide protection to the head, neck, face and hands from short- duration flame exposure and heat. This equipment is donned by shipboard navy personnel whenever a fire breaks out or during periods of Nomex/Kevlar Heat resistant heightened readiness. UV Stabilizers A number of additives are used to deal with potential issues arising from exposure to ultraviolet light. The most common problems arising from extended exposure of thermoplastics to UV are color shift of colored resins and yellowing of clear or light colored resins. Some thermoplastics can also suffer significant loss in mechanical properties. Glass and Mineral Filters Summary Factors Affecting Mechanical Properties during production Weld Lines Residual Stress Ultimate Strength Weld Lines The hairline grooves on the surface of a molded part where flow fronts join during filling - called weld lines or knit lines - cause potential cosmetic flaws and reduced mechanical performance. Weld Lines If the flow fronts are covered with a film from additives or a layer of impurities, they may not bind properly, which again can reduce impact and tensile strength. Resins can suffer more than a 50% loss of tensile strength at the weld line. Residual Stress Molding factors such as uneven part cooling, differential material shrinkage, or frozen-in flow stresses cause undesirable residual stresses in molded thermoplastics. High levels of residual stress can adversely affect mechanical properties (impact and tensile strength is reduced), chemical resistance and dimensional stability. Ultimate Strength During injection moulding, its polymer chains tend to align with the direction of the flow (see Figure). Generally, mechanical properties in the cross-flow direction are lower than those in the flow direction. Ultimate Strength The glass fibers in outer layers of glass-reinforced plastics tend to align in the direction of flow, resulting in higher tensile strength and stiffness in this direction. Generally, fiber-filled materials have much higher shrinkage (as much as 2 to 3 times greater) in the cross-flow than in the flow direction. Product Line Creation & Structure Most plastics manufacturers have broad product line of engineering thermoplastics. Their product line usually comprised of a number major product families with over 100 specific grades. For example : GE Plastics’ product line includes resins classified as amorphous, semi-crystalline and unique blends of both, specifically designed to meet diverse performance requirements. Figure below from GE Plastics positions the property profiles of these materials. Example: GE Plastics amorphous resin families include: Polymers Selection Dimensional Intricate Dark Max wall Material Colors Stiffness tolerance design colors thickness, mm ABS Many High Good Good Fair 5 Nylon Many High Good Fair Fair 4 Delrin Usually white Med Good Good Good 4 Polyethylene Many Low Poor Good Good 4 Polypropylene Many Low Fair Good Good 2 Many Polystyrene (translucent, opaque, Low Good Good Good 5 transparent, tinted) Many Polycarbonate (translucent, Med Good Good Fair 4 opaque, transparent, tinted) Some plastic trade names PP PC LDPE Adpro (Huntsman) Lexan (GE) Dowlex Cefor (Shell) Calibre (Dow) Eltex Comshield (ComAlloy) Makrolon (Bayer) BP Polyethylene Empee (Monmouth) Xantar Novapol (Nova Escalloy (ComAlloy) Kevlar (DuPont) chemical) Escorene (Exxon FR-PC (Lucky) Chemical) Iupilon (Mitsubishi) Ferrex (Ferro) Naxell (MRC Fina (Fina Oil) Polymer) Fortilene (Solvay) Panlite (Teijin Hostalen PP (Hoechst- chemical) Celanese) Sinvet (EniChem) Magnacomp (LNP) Trirex (Sam Yang) Marlex (Phillips Xenoy (GE) chemical) Multi-Flam (Multibase) Multi-Pro (Multibase) Nortuff (Polymerland) Polyfort (A Schulman) Propak (PolyPacific) Rexflex (Rexene) Verton (LNP) TYPES OF ENGINEERING MATERIALS Engineering materials Metals Polymers Ceramics Composites CERAMICS Formed from a combination of one or more metals with a non-metallic element such as oxygen, nitrogen or carbon. This combination leads to the formation of oxides, nitrides, borides, silicides and carbides. Generally very brittle. Possess good thermal and insulating properties. Good heat resistant. They have very high compressive strengths but the plasticity is poor. CERAMICS Some examples of ceramics are refractory, glasses, concrete, silica, magnesium oxide and fireclays. Typical applications are glasswares, electrical insulators, cutting tools and building bricks. Other Ceramic products Ceramic turbo-charge rotor 400 - 900 degree C TYPES OF ENGINEERING MATERIALS Engineering materials Metals Polymers Ceramics Composites COMPOSITES Composites are materials that are made from two or more materials uniquely combined together with properties which are not found in any single material. Within a composite material, one material will serve as a matrix to hold another material, the reinforcement. COMPOSITES The properties of composites vary in accordance to the type of composite concerned. For example, fibrous composites (such as roof covers) could be light and strong whereas the particulate composites (such as cermets) are hard and abrasive resistant. COMPOSITES One material will serve as a matrix (cement) to hold another material, the reinforcement (steel bars). COMPOSITES COMPOSITES Most common composite materials are made of at least two parts, the substrate and the resin. Substrate materials are like fiberglass, carbon fiber, Kevlar etc. Matrix/Resin materials act as a structural glue are like Polyester resin, Vinylester resin, Epoxy etc. Epoxy resin is a transparent matrix when cured and is used in the aerospace industry, COMPOSITES The end