Early Tooth Development PDF
Document Details
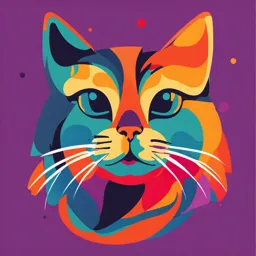
Uploaded by RenownedIvy
Tags
Summary
This document provides an overview of early tooth development, encompassing initiation, morphogenesis, and histogenesis stages. It details the cellular interactions and tissue differentiation involved in tooth formation, from the bud, cap, to bell stages. The document includes illustrations showing the developing teeth.
Full Transcript
Early tooth development development is characterised by complex interactions between epithelial...
Early tooth development development is characterised by complex interactions between epithelial Overview and mesenchymal tissues. The teeth develop by the mutual cooperation and interaction of an The first histological sign of tooth development is the appearance of a ectodermal tissue (the enamel organ) and mesenchymal tissue (the condensation of mesenchymal tissue and capillary networks beneath the dental papilla) that is of neural crest origin. As tooth development presumptive dental epithelium of the primitive oral cavity. The mesenchymal proceeds, there is an increased complexity apparent in terms of cells are ectomesenchymal (neural crest) in origin, having migrated into the histogenesis and morphogenesis (both of these being under the ‘control’ of the mesenchymal dental papilla). On the basis of the shape of the jaws from the margins of the neural tube. Recent research on amphibians enamel organ, the stages of tooth development progress from a bud and mammals suggests that, in addition to oral ectoderm and neural crest stage to a cap stage to a bell stage (when dentine and enamel begin mesenchyme, foregut endoderm plays a role in tooth initiation. Indeed, to be synthesised). Over 300 genes are involved in the control of tooth there is evidence that the specification of dental epithelium takes place development. in the oral epithelium adjacent to foregut endoderm and above midbrain neural crest cells. By the 6th week of development, the oral epithelium thickens and invaginates into the mesenchyme to form a primary epithelial band Tooth development can be divided into three overlapping phases: initiation, (Fig. 21.1). By the 7th week, the primary epithelial band divides into morphogenesis and histogenesis. During initiation, the sites of the two processes: a buccally located vestibular lamina and a lingually future teeth are established, with the appearance of tooth germs along situated dental lamina (Fig. 21.2). The vestibular lamina contributes to an invagination of the oral epithelium called the dental lamina. During the development of the vestibule of the mouth, delineating the lips and morphogenesis, the shape of the tooth is determined by a combination of cheeks from the tooth-bearing regions. The dental lamina contributes to cell proliferation and cell movement. During histogenesis, differentiation of the development of the teeth. To form the vestibule of the oral cavity, the cells (begun during morphogenesis) proceeds to give rise to the fully formed cells of the vestibular lamina proliferate, with subsequent degeneration dental tissues, both mineralised (i.e., enamel, dentine and cementum) of the central epithelial cells to produce the sulcus of the vestibule (Fig. and unmineralised (i.e., dental pulp and periodontal ligament). Tooth 21.3). Further development of the dental lamina (Fig. 21.4) is characterised Presumptive lip Presumptive jaw B A Fig. 21.2 The vestibular lamina (A) and dental lamina (B) seen at the 7th week of intrauterine life (H & E; ×120). Courtesy Dr D Adams. Fig. 21.1 Primary epithelial band (arrowed) at the 6th week of intrauterine life (H & E; ×115). Lip Jaw Fig. 21.3 Diagram illustrating the formation of the vestibule of the oral cavity. Cap stage 351 A Fig. 21.4 The developing dental A lamina (A) (Masson’s trichrome; B ×55). B A A Fig. 21.5 Model showing the Fig. 21.6 Bud stage of tooth development. A = Fig. 21.7 Early cap stage of tooth development stage of tooth development by the enamel organ; B = mesenchymal condensation (arrows). A = Meckel’s cartilage; B = developing 8th week of intrauterine life when (Masson’s trichrome; ×60). tongue (Masson’s trichrome; ×32). a series of swellings representing developing tooth germs (arrows) develops on the deep surface of the dental lamina. A = vestibular fold. by an increase in length, although it is not known whether this results from active invagination of the lamina or upward proliferation of the mesenchyme. By the 8th week, a series of swellings develops on the deep B surface of the dental lamina (Fig. 21.5). The complete dental lamina of A the lower jaw is shown in green on the model and the epithelial swellings E indicating early developing tooth germs are arrowed. It is important to C appreciate that the dental lamina appears as an arch-shaped band of D tissue, which follows the line of the vestibular fold. Although not shown on the model, each epithelial swelling is almost completely surrounded by a mesenchymal condensation. For descriptive purposes, tooth germs are classified into bud, cap and bell stages according to the degree of morphodifferentiation and Fig. 21.8 Late cap stage of tooth development. A = stellate reticulum; B = external enamel epithelium; C = histodifferentiation of their epithelial components (enamel organs). Leading internal enamel epithelium; D = dental papilla; E = dental follicle (H & E; ×75). up to the late bell stage, the tooth germ changes rapidly both in its size and shape; the cells are dividing and morphogenetic processes are taking place. At the late bell stage, hard tissues are forming and further growth Cap stage of the crown is related mainly to the deposition of enamel, the rate of cell division being reduced. By the 11th week, morphogenesis has progressed, the deeper surface of the enamel organ invaginating to form a cap-shaped structure. In the section Bud stage shown in Fig. 21.7, both maxillary and mandibular early cap stages are shown, each enamel organ appearing relatively poorly histodifferentiated. The enamel organ in the bud stage (Fig. 21.6) appears as a simple, spherical However, a greater distinction develops between the more rounded cells to ovoid, epithelial condensation that is poorly morphodifferentiated and in the central portion of the enamel organ and the peripheral cells, which histodifferentiated. It is surrounded by mesenchyme. The cells of the are becoming arranged to form the external and internal enamel epithelia. tooth bud have a higher RNA content than those of the overlying oral In the late cap stage of tooth development (Fig. 21.8), by about the 12th epithelium, a lower glycogen content and increased oxidative enzyme week, the central cells of the enlarging enamel organ have become separated activity. It would appear that the epithelium is instructive in tooth initiation (although maintaining contact by desmosomes), the intercellular spaces (see pages 356–357). Nevertheless, the successful development of the containing significant quantities of glycosaminoglycans. The resulting tooth germ relies upon a complex interaction of the mesenchymal and tissue is termed the stellate reticulum, although it is not fully developed epithelial components since, should these components be separated and until the later bell stage. The cells of the external enamel epithelium remain cultured individually, neither will differentiate further. The epithelial cuboidal, whereas those of the internal enamel epithelium become more component is separated from the adjacent mesenchyme by a basement columnar. The latter show an increase in RNA content and hydrolytic membrane. and oxidative enzyme activity, while the adjacent mesenchymal cells 352 Chapter 21 Early tooth development continue to proliferate and surround the enamel organ. The part of the It is during the bell stage of development that the dental lamina breaks mesenchyme lying beneath the internal enamel epithelium is termed the down and the enamel organ loses connection with the oral epithelium. At dental papilla, while that surrounding the tooth germ forms the dental the same time, the dental lamina between tooth germs also degenerates. follicle. A model describing the arrangement of deciduous tooth germs Remnants of the dental lamina may remain in the adult mucosa (Fig. at 13 weeks on the dental lamina of the lower jaw is shown in Fig. 21.9. 21.11) as clumps of resting cells (epithelial pearls [of Serres]) that may contain keratin and can be involved in the aetiology of cysts. Interposed between the enamel organ and the wall of the developing Early bell stage bony crypt is the mesenchymal tissue of the dental follicle, which is By the 14th week, further morphodifferentiation and histodifferentiation of generally considered to have three layers (Fig. 21.10). The inner investing the tooth germ lead to the early bell stage (Fig. 21.10). The configuration layer is a vascular, fibrocellular condensation, three to four cells thick, of the internal enamel epithelium broadly maps out the occlusal pattern of immediately surrounding the tooth germ; the nuclei of the cells tend to the crown of the tooth. This folding is related to differential mitosis along be elongated circumferentially. The outer layer of the dental follicle is the internal enamel epithelium. The future cusps and incisal margins are represented by a vascular mesenchymal layer that lines the developing sites of precocious cell maturation associated with cessation of mitosis, alveolus. Between the two layers is loose connective tissue with no marked while areas corresponding to the fissures and margins of the tooth remain concentration of blood vessels. There is evidence that the cells of the mitotically active. Thus, cusp height is related more to continued downward inner layer of the dental follicle may be derived from the neural crest. growth at the margin and fissures than to upward extension of the cusps. A high degree of histodifferentiation is achieved in the early bell stage During the bell stage, any bone resorption defects that restrict the space (Fig. 21.12). The enamel organ shows four distinct layers: external enamel for development of the tooth germ may be associated with the increased epithelium, stellate reticulum, stratum intermedium and internal enamel folding pattern of the internal enamel epithelium, leading to changes in tooth epithelium. shape. Consequently, spatial impediment, and the changing mechanical The cervical loop at the margins of the enlarging bell-shaped enamel forces that ensue, may be a cofactor in dental morphogenesis. organ is a site of mitotic activity. Here, the central cells of the stellate reticulum/stratum intermedium may be the site of a stem cell niche providing cells that pass to the internal enamel epithelium and later form ameloblasts. This may be under the control of Notch protein in the epithelium and growth factors, such as BMP-4 (bone morphogenetic protein-4) and FGF-10 (fibroblast growth factor-10), in the adjacent dental mesenchyme (see page 352). V External enamel epithelium B A D C As its name suggests, this forms the outer layer of cuboidal cells that E limits the enamel organ. It is separated from the surrounding mesenchymal tissue by a basement membrane 1–2 µm thick, which, at the ultrastructural level, corresponds to the much narrower basal lamina with associated hemidesmosomes (Fig. 14.29). The external enamel epithelial cells contain large, centrally placed nuclei. Ultrastructurally, they contain relatively small amounts of the intracellular organelles associated with protein synthesis (e.g., endoplasmic reticulum, Golgi material, mitochondria) Fig. 21.9 Model illustrating the arrangement of deciduous tooth germs (identified by the Palmer-Zsigmondy and they contact each other via desmosomes and gap junctions. The system, A–E) at 13 weeks on the dental lamina of the lower jaw. V = vestibular fold. B A A Fig. 21.10 Early bell stage of tooth development. A = inner investing layer of dental follicle; B = outer layer of Fig. 21.11 Epithelial pearls (of Serres) (arrows). A = dental follicle (Masson’s trichrome; ×45). enamel space (H & E; ×8). Courtesy Dr D A Luke. Early bell stage 353 D A SR F C E G Fig. 21.13 Immunohistological labelling of type II collagen showing positive (white) labelling in the stellate reticulum (SR) (×350). B Fig. 21.12 A high-power view of the early bell. A = external enamel epithelium; B = cervical loop; C = stellate reticulum; D = enamel cord; E = stratum intermedium; F = internal enamel epithelium; G = dental papilla (Masson’s trichrome; ×120). B external enamel epithelium is thought to be involved in the maintenance of the shape of the enamel organ and in the exchange of substances between the enamel organ and the environment. The cervical loop, at A which there is considerable mitotic activity, lies at the growing margin of the enamel organ where the external enamel epithelium is continuous with the internal enamel epithelium. Stellate reticulum This tissue is most fully developed at the bell stage. The intercellular spaces become filled with fluid, presumably related to osmotic effects B arising from the high concentration of glycosaminoglycans. The cells are star-shaped with bodies containing conspicuous nuclei and many branching processes. In addition to glycosaminoglycans, the cells also Fig. 21.14 Ultrastructural appearance of the stellate reticulum. A = desmosomes; B = intercellular space; arrows contain alkaline phosphatase but have only small amounts of RNA and show tonofilaments (TEM; ×6,380). Courtesy Dr H Azawa and Dr H Nakamura. glycogen. The mesenchyme-like features of the stellate reticulum include the synthesis of collagens in the tissue. Collagens types I, II and III are expressed in the cells of the stellate reticulum, although their functional suggested that the hydrostatic pressure generated within the stellate significance is unclear (Fig. 21.13). reticulum is in equilibrium with that of the dental papilla, allowing the The cells of this layer (Fig. 21.14) possess little endoplasmic reticulum proliferative pattern of the intervening internal enamel epithelium to and few mitochondria. However, there is a relatively well developed determine crown morphogenesis. However, a change in either of these Golgi apparatus, which, together with the presence of microvilli on the pressures might lead to a change in the outline of the internal enamel cell surface, has been interpreted as indicating that the cells contribute epithelium, and this could be important for crown morphogenesis. to the secretion of the extracellular material. Numerous tonofilaments The stellate reticulum also produces macrophage colony-stimulating are present within the cytoplasm, and desmosomes and gap junctions factor (MCSF), transforming growth factor (TGF)β1 and parathyroid are present between the cells. hormone-related protein (PTHrP). These molecules may be released into The main function ascribed to the stellate reticulum is a mechanical the dental follicle and help recruit, and activate, the osteoclasts necessary one. This relates to the protection of the underlying dental tissues against to resorb the adjacent alveolar bone as the developing tooth enlarges and physical disturbance and to the maintenance of tooth shape. It has been erupts (see pages 422–423). 354 Chapter 21 Early tooth development Stratum intermedium Late bell stage This first appears at the bell stage and consists of two or three layers of The late bell stage (appositional stage) of tooth development (Fig. 21.16) flattened cells lying over the internal enamel epithelium (and its derivatives). is associated with the formation of the dental hard tissues, commencing at The cells of the stratum intermedium resemble the cells of the stellate about the 18th week. Dentine formation always precedes enamel formation. reticulum, although their intercellular spaces are smaller and the cells Detailed accounts of amelogenesis and dentinogenesis are given in Chapters contain more alkaline phosphatase. It has been suggested that the stratum 22 and 23. In the section shown in Fig. 21.16, downgrowths of the intermedium is concerned with the synthesis of proteins, the transport of external enamel epithelium appear from the lingual sides of the enamel materials to and from the enamel-forming cells (the ameloblasts) derived organs. In deciduous teeth, these lingual downgrowths give rise to the from the internal enamel epithelium and/or the concentration of materials. tooth germs of the permanent successors and first appear alongside the Internal enamel epithelium incisors at about 5 months in utero. In enamel organs of permanent teeth, however, these downgrowths eventually disappear. Behind the deciduous The cells of this layer are columnar at the bell stage but, beginning at second molar, the dental lamina grows backwards to bud off successively the regions associated with the future cusp tips (i.e., the sites of initial the permanent molar teeth. The first permanent molar appears at about 4 enamel formation), the cells become elongated. The internal enamel months in utero, the tooth bud for the second permanent molar appears epithelial cells are rich in RNA but, unlike the stratum intermedium and about 6 months after birth, while that for the third permanent molar stellate reticulum, do not contain alkaline phosphatase. Desmosomes appears at about 4–5 years after birth. connect the internal enamel epithelial cells and link this layer to the Fig. 21.17 provides a high-power view of a region of a tooth germ at stratum intermedium. The internal enamel epithelium is separated from the late bell stage to show enamel and dentine formation commencing at the peripheral cells of the dental papilla by a basement membrane and a the tips of future cusps (or incisal edges). Under the inductive influence of cell-free zone 1–2 µm wide. developing ameloblasts (preameloblasts), the adjacent mesenchymal cells The differentiation of the dental papilla is less striking than that of the of the dental papilla become columnar and differentiate into odontoblasts. enamel organ. Until the late bell stage, the dental papilla consists of closely The odontoblasts then become involved in the formation of predentine packed mesenchymal cells with only a few delicate extracellular fibrils. and dentine. The presence of dentine then induces the ameloblasts to Histochemically, the dental papilla becomes rich in glycosaminoglycans. secrete enamel. Fig. 21.15 provides a model demonstrating the arrangement of deciduous tooth germs at 17 weeks on the dental lamina of a lower jaw quadrant. Transitory structures The dental lamina (green) is beginning to degenerate. Downgrowths on the lingual aspect of the enamel organs indicate the early development During the early stages of tooth development, three transitory structures of the successional (permanent) teeth. may be seen: the enamel knot, enamel cord and enamel niche. A A B C D E Fig. 21.15 The arrangement of deciduous enamel organs (identified by the Palmer-Zsigmondy system, A–E) at Fig. 21.16 Late bell stage (appositional stage) of tooth development. Dentine matrix stained blue; enamel matrix 17 weeks on the dental lamina of a lower jaw quadrant. Arrow indicates developing permanent tooth. stained red. A = permanent tooth (Masson’s trichrome; ×60). Transitory structures 355 Enamel knot of the BMP, FGF, Shh (sonic hedgehog) and Wnt families. The primary enamel knot is not a permanent feature of the tooth germ and is removed An important development during the bud stage is the induction by by apoptosis at the end of the cap stage. In monocuspid teeth (incisors, the mesenchyme of the primary enamel knot (Fig. 21.18). This is a canines), the primary knot is the only one to form and it marks the site localised, nonproliferating cluster of cells at the centre of the inner dental of the tooth tip. However, in premolars and molars, additional secondary epithelium, which is central to establishing the form of the tooth crown. knots are formed, possibly with contributions from the primary knot, at Characteristically, the enamel knot forms a bulge into the dental papilla, at the sites of the future cusps and in a sequence that matches the relative the centre of the enamel organ. The primary enamel knot is an important importance of the cusps in the mature tooth. Although the primary and signalling centre expressing ten or more growth factors, including members secondary knots consist of nondividing cells, it is thought that they secrete mitogenic factors that diffuse outwards to stimulate proliferation and folding of the adjacent internal enamel epithelial and mesenchymal cells. This results in expansion of the epithelial cap and also in folding of the inner dental epithelium; downgrowth of the expanding dental epithelium E on the flanks of the enamel knots result in the formation of cusps during the bell stage. Many mutations affecting signalling factors associated B with enamel knots result in altered tooth morphology. As many genes participate in the signalling activities of the enamel knots, the system is capable of very fine control of crown morphology. Fig. 21.19A shows Shh in the primary enamel knot and Fig. 21.19B shows BMP-2 expression in secondary enamel knots in molars at the sites of cusp formation. Fig. 21.20 demonstrates FGF-20 expression in the enamel knots of molars. C Enamel cord A The enamel cord (Fig. 21.21) is a strand of cells seen at the early bell stage of development that extends from the stratum intermedium into D the stellate reticulum. When present, the enamel cord overlies the incisal margin of a tooth or the apex of the first cusp to develop (primary cusp). When it completely divides the stellate reticulum into two parts, reaching the external enamel epithelium, it is termed the enamel septum. Where the enamel cord meets the external enamel epithelium, a small invagination termed the enamel navel may be seen. The cells of the enamel cord are distinguished from their surrounding stellate reticulum cells by their elongated nuclei. It has been suggested that the enamel cord may be involved in the process by which the cap stage is transformed into the Fig. 21.17 High-power view of a region of a tooth germ at the late bell stage to show enamel and dentine formation. A = odontoblasts; B = ameloblasts; C = stratum intermedium; D = stellate reticulum; E = external bell stage (acting as a mechanical tie) or that it is a focus for the origin enamel epithelium; dentine matrix stained green; enamel matrix stained red (Masson’s trichrome; ×130). of stellate reticulum cells. A A B Fig. 21.18 The enamel knot (A) (H & E; ×120). Fig. 21.19 (A) Staining for Shh (sonic hedgehog – red stain) in the primary enamel knot of a molar tooth by in situ hybridization (×140). (B) Staining for BMP-2 (red stain) in the secondary enamel knots of a molar tooth by in situ hybridization (×140). Courtesy Dr M Jussila. 356 Chapter 21 Early tooth development B B C A A Fig. 21.22 The enamel niche (C). A = lateral enamel strand; B = medial Fig. 21.20 Staining for FGF20 expression (red stain) in upper and lower enamel strand (H & E; ×40). molar tooth germs by in situ hybridization (×50). Courtesy Dr M Jussila. Fig. 21.21 The enamel cord (A). B = enamel navel (Masson’s trichrome; ×120). Enamel niche external enamel epithelium. Although vessels may lie in invaginations of the external enamel epithelium, they never penetrate the stellate reticulum. The enamel niche (Fig. 21.22) is seen where the tooth germ appears to have a double attachment to the dental lamina (the lateral and medial enamel strands). These strands enclose the enamel niche, which appears Epithelial–mesenchymal interactions during as a funnel-shaped depression containing connective tissue. The functional tooth development significance of the enamel niche is unknown. The enamel cord and the Tooth development (odontogenesis) is a very complex process involving double attachment of the tooth germ around the enamel niche were once many growth and transcription factors to ensure an ordered, and controlled, regarded as evidence supporting the view that the complex crown form development for both individual tooth germs and the whole dentition. of mammalian teeth evolved from fusion of several individual, simpler Epithelial–mesenchymal interactions are particularly in evidence and require elements. However, this view is not now accepted. signalling between the two major components of the tooth germ, one derived from the oral epithelium and one from the underlying mesenchyme. Nerve fibres The tooth germ can be readily manipulated experimentally and therefore has been used not only to investigate the processes involved in odontogenesis There is conflicting evidence as to when, and where, nerve fibres first but also as a useful model for understanding epithelial–mesenchymal appear during tooth development. It has been reported that nerve fibres interactions in general. Increasingly, the main purpose of research into are present in the immediate vicinity of presumptive dental epithelium at odontogenesis is being seen in the context of tooth ‘regeneration’ for the very earliest stage of tooth induction and subsequently form a plexus clinical dentistry. By understanding early odontogenic signals, it may below the dental papilla at the cap stage. From such plexuses, the nerves eventually be possible to engineer dental tissues from stem cells. Although spread into the dental follicle as it develops. Penetration of nerves into many problems await, arriving at such techniques to allow for tooth the dental papilla occurs with the onset of dentinogenesis. The nerve regeneration would undoubtedly revolutionise dentistry. fibres associated with blood vessels are presumed to be autonomic; others lying free within the papilla are presumed to be sensory. However, the Initiation of tooth development innervation of the dental papilla remains rudimentary until after birth and may be fully developed only after the tooth has erupted. Controversy also Techniques have been developed whereby it is possible to dissect out, remains concerning the role of neuronal cells and neurotrophins in tooth and recombine, mammalian epithelium and mesenchyme in different development. Recent work indicates that, although nerve fibres are not combinations before there are any signs of tooth development. Tissue required for odontogenesis, the pattern of localisation of neurotrophins recombination experiments have shown that the epithelium lining the first (and their associated receptors) does suggest a role for neural-like cells – pharyngeal (branchial) arch has ‘odontogenic potential’ as, when first arch perhaps related to the neural crest derivation of the dental mesenchyme. epithelium is combined with first arch mesenchyme, tooth germs form. However, in transgenic animals where neurotrophins and their receptors However, with the reciprocal experiment, no tooth germs develop when are not expressed, tooth development is not affected. the epithelium from the second arch is combined with first or second arch mesenchyme (instead, bone and cartilage develop). The potential for Blood supply first arch epithelium to initiate tooth development only exists in the very early stages of odontogenesis. Thereafter, when first arch mesenchyme Small blood vessels invade the dental papilla at the early bell stage. is combined with second arch epithelium (which, as stated above, does They are also evident in the dental follicle in close association with the not initiate odontogenesis), tooth germs are formed. This suggests that, Epithelial–mesenchymal interactions during tooth development 357 Table 21.1 Derivatives of the cranial neural crest cells Ganglia Skin Carotid body cells Craniofacial skeleton Sensory cranial ganglia Melanocytes C cells of the ultimobranchial Dermal bone-forming cells body and thyroid Parasympathetic (ciliary) Pigment cells of Endochondral osteocytes ganglia the inner ear Enteric ganglia Chondrocytes Satellite glial cells in ganglia Other cells in head and neck Enteric glia Myofibroblasts/smooth muscle cells (conotruncus and aortic arch-derived arteries) Schwann cells along PNS Pericytes in brain nerves Ensheathing and olfactory cells Meninges (forebrain) lining the olfactory nerve Odontoblasts, cells in periodontal ligament and tooth papillae Adipocytes Dermal cells of the face Connective tissue cells of glands, muscles and tendons Corneal cells in endothelium and stroma Ciliary muscles after initiation by the oral epithelium, the ‘control’ of tooth development passes to the mesenchyme. This view is supported by research into the role of the enamel organ and the papilla at the cap stage of development (see page 359). Many investigations have been conducted into the role of neural crest cells (ectomesenchyme) in odontogenesis and there is much evidence from both nonmammalians and mammalians showing that the mesenchyme within a tooth germ is derived from the neural crest. Table 21.1 provides information regarding the derivatives of the cranial neural crest. It has also been shown that cranial neural crest cells taken from their site of origin (near the developing neural tube), and before their migration into the pharyngeal arches, can produce tooth germs when combined with first arch epithelium (Fig. 21.23) but not when combined with second arch epithelium or epithelium from other sites (Fig. 21.24). It seems that the neural crest tissue migrating into the first arch is ‘unspecified’ and requires an interaction with the oral epithelium before it becomes odontogenic. Thus, the oral epithelium is instructive. The signals from the oral epithelium include Shh, BMP-4, FGF-8 and Pitx2. These initiate the expression of transcription factors such as Msx1 and Pax9. It seems that the earliest markers of odontogenesis are the expression, well in advance of histogenesis, of Pitx2 in the epithelium and of Pax9 in the mesenchyme, followed by expression of other factors (e.g., Shh). The origins of the oral epithelium involved in tooth development has also been investigated, it being commonly believed that the epithelium Fig. 21.23 The result of culturing premigratory cranial neural crest with oral epithelium. Note the developing is derived from ectoderm. Recent studies to investigate this matter have tooth (arrowed) (Masson’s trichrome; ×65). From Lumsden AGS 1988 Spatial organisation of the epithelium and the role of neural crest cells in the initiation of the mammalian tooth germ. Development 103: 155–169. involved comparing the molecular characteristics of oral ectoderm with those of pharyngeal endoderm. It was found that Claudin6, Hnf3β, α-fetoprotein, Rbm35a and Sox2 are expressed in the endoderm and Mechanisms responsible for specifying the also where molars are developing, suggesting that molar teeth (but not tooth-forming zones in the oral region and for more anterior teeth) are derived from epithelium of endodermal origin. controlling tooth number This being the case, multicuspid teeth would evolve from ‘externalisation’ of pharyngeal tooth-like structures while monocuspids would evolve from The transcription factor Pitx2 is selectively expressed in the oral epithelium ‘internalisation’ of ectodermal structures (e.g., the ectodermal armour from the earliest stages of oral cavity formation and its expression is odontodes found in ancient fish). thereafter restricted to dental epithelium. As with a broad range of other 358 Chapter 21 Early tooth development B Fig. 21.25 (A) 42 mineralised teeth of various sizes collected from one developing molar tooth in a mouse in which Wnt signalling was sustained. (B) The three molars present in normal mouse controls (×13). From Jarvinen E et al 2006 Continuous tooth generation in mouse is induced by activated epithelial Wnt/β-catenin signalling. Proceedings of the National Academy of Sciences of the United States of America 103: A 18627–18632. Fig. 21.24 The result of culturing premigratory neural crest with limb epithelium. Teeth do not develop; only islands of bone and cartilage (Masson’s trichrome; ×200). From Lumsden AGS 1988 Spatial organisation of the epithelium and the role of neural crest cells in the initiation of the mammalian tooth germ. Development 103: 155–169. tissues, Sonic hedgehog (Shh) is a major determinant of (or at least marks) the sites at which teeth develop. Shh gene expression is restricted to the dental epithelium at sites of tooth development and also appears to be involved in epithelial–mesenchymal interactions. As Shh increases Fig. 21.26 Chick ‘tooth-like structure’ formed by recombining chick mandibular epithelium (E) with chick skin cell proliferation, restriction of expression to tooth-forming regions is mesenchyme (M) and culturing for 2 days (×80). From Chen Y et al 2000 Conservation of early odontogenic signalling pathways in Aves. Proceedings of the National Academy of Sciences of the United States of America 97: important and seems to be accomplished by interactions with Wnt-signalling 10044–10049. molecules. Indeed, Wnt helps maintain boundaries between tooth-forming areas and non-tooth-forming areas. The importance of Wnt/7b signalling can also be seen from experiments where its activity is inhibited, leading to into epithelial–mesenchymal interactions. Because the ancestors of birds the arrest of tooth morphogenesis at an early stage. Conversely, where Wnt had teeth, the question arises as to whether there is any latent potential signalling is stimulated (by stabilising beta-catenin) in the oral epithelium, in either the epithelium and/or mesenchyme of birds to form teeth and it results in the production of dozens of extra teeth. In the molar region whether this potential can be unlocked. Research has shown that several of mice, these supernumerary teeth are in the form of unicuspid cones growth and transcription factors expressed during tooth development in (Fig. 21.25) and this has been interpreted as evidence of a direct role for mammals are present in the oral epithelial and mesenchymal tissues of Wnt signalling in the formation of supernumerary enamel knots. It has chicks (e.g., FGF-8, Pitx2, Barx1 and Pax9). However, other factors are also been suggested that the retention of Wnt signalling may account for missing, such as Shh, BMP-4, Msx1 and Msx2. When BMP-4 protein is the continuous succession of teeth in nonmammalian vertebrates. added exogenously to chick mandibles, it induces the expression of Msx1 An additional way of restricting Shh signalling activity involves the and Msx2 in the mesenchyme. When chick epithelium is cultured with upregulation of its receptor, Patched (Ptc1) in excess of the Shh ligand chick dorsal skin mesenchyme that does contain BMP-4, tooth-bud-like and this mechanism has been found in the non-tooth-forming areas (the structures are produced (Fig. 21.26). diastema region of rodents). Experiments have been undertaken in which chick neural crest is The Pax9 and Msx1 genes appear to be required to enable the tooth replaced by mouse neural crest (Fig. 21.27). The resulting mouse/chick germ to progress beyond the bud stage, as absence of the gene arrests chimaeras produce tooth germs (Fig. 21.28) up to the stage of the initial the tooth germ at the bud stage. formation of organic matrix. This matrix is regarded as a dentine matrix, Some important information concerning the mechanisms responsible as the odontoblast-like cells produce dentine sialoprotein and nestin. This for specifying tooth–forming zones has arisen from study of ‘hens’ teeth’. experiment indicates that mouse neural-crest-derived mesenchymal cells in The absence of teeth in modern birds has given rise to the phrase ‘as rare avian oral epithelium have the ability to induce the avian oral epithelium as hens’ teeth’. However, the topic also provides a stimulus to research and thus initiate the avian tooth developmental programme. That the teeth Epithelial–mesenchymal interactions during tooth development 359 Chick embryo wt ta 2 ec Head me ab or l Chimaera Fig. 21.29 Model of alteration in the inductive interactions in wild-type chicks (wt) and ta2 jaw leading to the initiation of teeth in the ta2 mutant. In the wild type, a regional signalling centre is localised in the epithelium (yellow) by the interaction between FGF-8, BMP-4 and Shh signalling. This signalling centre demarcates the boundary between the oral and aboral epithelium (vertical mark on horizontal ab–orl line). This epithelial signalling centre does not overlie oral mesenchyme (purple) competent to make appendage structures. In the ta2 mutant, early changes in FGF-8, BMP-4 and Shh signalling lead to medial positioning of the forming oral/ aboral boundary such that the signalling centre and underlying competent mesenchyme are juxtaposed, permitting initiation of tooth developmental programmes. ab = aboral; ec = ectoderm; me = mesenchyme; orl = oral epidermis. From Harris MP, Hasso SM, Ferguson MWJ, Fallon JF 2006 The development of Archosaurian first- Mouse embryo generation teeth in a chicken mutant. Current Biology 16: 371–377. Head Fig. 21.27 Diagram of the experimental procedure of mouse neural tube transplantation into chick host embryos for the creation of a chick/mouse chimaera that has an abnormal head morphology. From Mitsiadis TA, Caton J, Cobourne M 2006 Waking up the sleeping beauty: recovery of the ancestral bird odontogenic program. Journal of Experimental Zoology (Molecular and Developmental Evolution) 306B: 227–233. A Fig. 21.30 The result of culturing dental papilla mesenchyme with epithelium from the developing foot pad. A normal tooth B develops (Masson’s trichrome; ×40). From Kollar EJ, Baird GR 1970 Tissue interactions in embryonic mouse tooth germs II. The inductive role of the dental papilla. Journal of Embryology and Experimental Morphology 24: 173–186. Fig. 21.28 ‘Chick tooth’ derived from the chick/mouse chimaera experiments shown in Fig. 21.27 at the bell stage. The epithelial enamel organ (A) is chick-derived and the dental papilla (B) is mouse-derived. The blue staining represents initial dentine matrix (Masson’s blue trichrome; ×63). Courtesy Dr T A Mitsiadis. components between different developing teeth (incisors and molars) and with tissues from nondental regions. The results indicated that, at the cap fail to mineralise properly and produce enamel is not surprising, as the stage of tooth development, the principal organiser is the dental papilla, chick genome appears to lack the genes associated with the production in terms of both morphogenesis and histogenesis. The result of culturing of enamel matrix. dental papilla mesenchyme with epithelium from the developing foot pad A recent experiment that sheds further light on why modern birds lack (Fig. 21.30) is normal tooth development, illustrating the importance of teeth relates to a chick mutant known as ‘talpid’ (ta2). This is an autosomal the dental papilla. On the other hand, if the enamel organ of a tooth is recessive mutation that has serious consequences for the development cultured with mesenchyme from the developing foot pad (Fig. 21.31), tooth of many organ systems. However, this mutant also uniquely exhibits the development does not occur. Similar experiments have been conducted to development of simple, unmineralised, toothlike structures in the jaws. In determine whether the enamel organ or the dental papilla determine tooth explanation, from analysis of the distribution of growth and transcription shape. Such experiments involve separating and recombining enamel organ factors (Fig. 21.29), it can be seen that a responsive mesenchyme is and papilla at the cap stage. Should an incisor enamel organ be combined present in the normal (wild-type) situation that is displaced away from the with a molar papilla, the resulting tooth is molariform. Furthermore, if responsive epithelium above, whereas in the mutant talpid the responsive a molar enamel organ is combined with an incisor papilla, the resulting mesenchyme is displaced to lie directly beneath the responsive epithelium. tooth is incisiform. Thus, as for histogenesis, the dental papilla is the dominant tissue determining tooth shape at the cap stage. Mechanisms responsible for specifying tooth type From the above experiments, research has progressed to try to understand and tooth shape (morphogenesis) the molecular mechanisms involved in morphogenesis. The underlying control of such patterning is based upon the hypothesis that, as in other Early experiments were designed to answer the question posed by parts of the body (such as the vertebral column), patterning is related to the investigators: which of the two components is more important for inducing spatially restricted expression of homeobox genes in the ectomesenchyme morphogenesis and histogenesis – the enamel organ or the dental papilla? of the developing jaws (referred to as the odontogenic homeobox code). Such experiments involved interchanging the epithelial and mesenchymal These genes contain DNA-binding proteins that regulate gene transcription, 360 Chapter 21 Early tooth development Barx1 Dlx1/2 Msx1 Msx2 Alx2 Fig. 21.31 The result of culturing the enamel organ of a tooth with mesenchyme from the developing foot pad. Note the absence of tooth development (Masson’s trichrome; ×75). Courtesy Prof. E Kollar and the editor of the Journal of Embryology and Experimental Morphology. thus controlling the expression of other genes necessary for the development A of a particular structure, in this case a tooth. Accordingly, the group of homeobox genes expressed in the presumptive incisor region will dictate that incisiform teeth develop here, while the group of homeobox genes expressed at the back of the tooth row (in the presumptive molar region) will specify molariform teeth. For this hypothesis to be tenable, an important prerequisite is to establish that the presumptive incisor and molar regions do indeed contain some difference in their homeobox gene array and this feature has been demonstrated. It is known that the neural crest cells for the mesenchyme of the first pharyngeal arch express non-Hox B homeobox genes, including Msx1, Msx2, Dlx1, Dlx2, Barx1 and Alx3. Different combinations of these homeobox genes appear to instruct the tooth germ with respect to its tooth type. Thus, the neural-crest-derived C mesenchyme in the incisor region expresses Msx1 (but not Barx1) while Fig. 21.32 Schematic diagrams to show the mesenchymal odontogenic homeobox gene code. (A) Diagram neural-crest-derived mesenchyme in the molar region expresses Barx1 (but illustrating the lower jaw of the mouse. Note the overlap (orange) between the domains of Msx1 (red) and Msx2 not Msx1) (Figs 21.32, 21.33). As with other systems of early development (yellow). (B) Model representing the mouse dentition. Note that molars develop from cells expressing Barx1 and incisors from cells expressing Msx1, Msx2 and Alx3. (C) Model representing the human dentition, where it is and differentiation, temporal factors mean that there is only a narrow predicted that incisors develop from cells expressing Msx1, Msx2 and Alx3, canines and premolars from cells window of opportunity to successfully manipulate the homeobox genes. expressing Msx1 and Msx2 and molars from cells expressing Barx1. Courtesy Prof. P T Sharpe and the editor of Bioessays. The difference in distribution of homeobox genes between the incisor and molar regions is at least consistent with the odontogenic homeobox code hypothesis. Evidence to support the importance of such molecules Msx-1 Msx-2 Lhx-7 in initiating tooth development can be seen in transgenic mice where, e.g., an absence of the homeobox gene Msx1 results in tooth development being arrested at the bud stage. Similarly, early mesenchyme isolated from the overlying epithelium can still continue to develop in tissue culture if molecules such as BMPs and FGFs are added to the medium. Dlx-2 Barx-1 The odontogenic homeobox code can be considered in the light of a ‘field model hypothesis’ where each tooth germ starts the same but different concentrations of morphogens (e.g., growth factors) in the local environment are responsible for producing different tooth types. The patterning of the dentition has been shown to be related to the polarity of the epithelium. This epithelial polarity is thought to be associated with Fig. 21.33 Whole-mount in situ hybridisation to show localisation of homeobox genes (the darkly ‘staining’ regions) for the developing mandible. Courtesy Prof. P T Sharpe. expression of FGF-8 and BMP-4 growth factors. Accordingly, FGF-8 (with FGF-9) is expressed in the future molar region, whereas BMP-4 is expressed in the future incisor region. In relation to the homeobox genes, developing tooth can be altered. In this context, it is possible to culture FGF-8 stimulates expression of Barx1 and Dlx2 and BMP4 stimulates early incisor tooth germs and absorb the BMP produced by the epithelium Msx1 and Msx2 but inhibits Barx1. (so that it does not switch on Msx1 in the underlying mesenchyme cells). One way of assessing the ‘field model hypothesis’ is to try to change This is achieved by placing on the epithelial surface very small beads the odontogenic homeobox code and determine whether the shape of a soaked in noggin protein, which is a BMP antagonist. In this situation, Epithelial–mesenchymal interactions during tooth development 361 A B C D Fig. 21.34 The results of experiments to transform teeth from incisor to molar shape following downregulation of Msx1 and upregulation of Barx1 in incisors. (A) Control section of normal molar. (D) Control section of normal M3 incisor. (B, C) Sections from incisor teeth showing change to molar shape following upregulation of Barx1 in the mesenchyme (×25). From Tucker AS, Matthews KL, Sharpe PT 1998 Transformation of tooth type induced by M1 inhibition of BMP signaling. Science 282: 1136–1138. M2 not only is Msx1 downregulated in the mesenchyme but Barx1 (normally only present in the molar region) is seen to be expressed. When such tooth germs are then transplanted in vivo to renal capsules and allowed to continue to develop, multicuspid teeth with a molariform rather than an incisiform morphology can be produced (Fig. 21.34). The odontogenic homeobox code therefore would explain the formation of different tooth homologies. There is also evidence that the odontogenic homeobox code differs in Fig. 21.35 A tooth germ of the first molar at the cap stage of development has been removed from an embryo the mandibular and maxillary regions. This is based upon the observation and transplanted for culture in a different site. Note the development of first (M1), second (M2) and third molars (M3) (Alizarin red whole-mount; ×10). From Lumsden AGS 1988 Spatial organisation of the epithelium and the that, when Dlx1 and Dlx2 gene expression is disrupted, mandibular molars role of neural crest cells in the initiation of the mammalian tooth germ. Development 103: 155–169. still developed but not maxillary molars. In contrast to the ‘field model hypothesis’, a ‘clonal model hypothesis’ has been put forward to explain tooth form. Accordingly, the tooth type is changes from the bud to the cap. Indeed, the survival of the enamel knot prespecified (probably at the original site of the neural crest cells) and is is a balance between growth and apoptosis (FGF-4 for growth, BMP-4 for not dependent on the environment within the jaws. An experiment in which apoptosis). the possible contribution of the jaw environment was assessed required the removal of the dental lamina in the developing molar region, together The nature of the inductive message with the surrounding mesenchyme. This very early, and undifferentiated, tooth germ (which would have given rise to the first molar) was then During tooth development, ‘messages’ pass between the epithelium cultured in a site well away from the jaws. A tooth germ of the first molar and mesenchyme to produce changes of increasing complexity (i.e., at the cap stage of development removed from an embryo and transplanted differentiation) within the cell layers. The term ‘induction’ is used to for culture at a different site was seen to continue to develop normally describe the effect that one cell layer has on another. It has clearly been and the remaining second and third molars also developed (Fig. 21.35). shown that bioactive signalling molecules in the form of small proteins This finding is consistent with the idea that a series of related structures pass between the epithelium and mesenchyme and usually have important can form by budding off from a single precursor and that the differences interactions with the receptors on the cell membrane. These interactions between the individual structures (e.g., size and crown complexity) result set off a series of intracellular cascades that regulate gene expression from the increasing age of the tooth-budding region as it grows distally and change the behaviour of the cell. It can still be instructive to refer from the jaw (rather than from local environmental factors in the jaws). to early experiments designed to investigate the nature of the inductive This ‘clonal model hypothesis’ could explain the successionary tooth message. Three main hypotheses were put forward to explain how formation of the second and third molars. information leading to induction might be transferred between epithelium The formation of the specific shape of a tooth (including cusps) is and mesenchyme. signalled during the bud and cap stages of tooth development. Particular 1) A chemical substance (short-range hormone) is produced by one cell significance in morphogenesis has recently been placed on the presence of layer and diffuses across the narrow intervening space to be taken up the enamel knot (Fig. 21.19). The shape of the enamel organ (producing and cause induction in the other cell layer. the shape of the crown of the tooth) relates to the pattern of folding 2) Induction is triggered by direct cell-to-cell contact and does not involve seen within the internal enamel epithelium and/or the forces present a diffusible molecule. on either side of it. The enamel knot is thought to play a role in this 3) Induction results from the presence of the initial extracellular matrix, morphogenesis as it is associated with each main cusp that develops and a thin layer situated between the epithelium and mesenchyme and as it possesses molecules seen in other patterning situations. The enamel comprising the basal lamina and adjacent region. The extracellular knot is under the direction of signals emanating from the underlying matrix has a complex composition, consisting of collagen (mainly type mesenchyme, BMP-4 stimulating the expression of p21 (an inhibitor of IV but possibly some type I and III), proteoglycans and glycoproteins. cell proliferation) to produce the enamel knot. The enamel knot itself also expresses signalling molecules (FGF-4, Shh and BMPs and Wnt growth To assess which of the three hypotheses is likely to provide the correct factors). Shh in particular is involved in the formation of the cap stage of explanation for reciprocal control of differentiation, experiments have the tooth germ, regulating cell survival at the tip of the enamel organ as it been undertaken in which the epithelial and mesenchymal components are 362 Chapter 21 Early tooth development dissected out and separated at the early bell stage, before any significant differentiation of the tooth germ. Second, isolated pieces of extracellular degree of cytodifferentiation. They are then recombined for tissue culture, matrix will produce histological signs of differentiation in internal enamel but with a porous membrane placed between; the size of the pores in the epithelial cells of the enamel organ. It is conceivable that, in relation membrane can be varied to ascertain the point at which both ameloblasts to the physical properties of the initial extracellular matrix, this matrix and odontoblasts differentiate. As molecules can readily diffuse through provides a surface for attachment of cells or enables interactions between a pore size just less than 0.2 µm, the absence of differentiation seen bioactive molecules that induce early differentiation. in Fig. 21.36 appears to argue against hypothesis 1 above (a diffusible chemical substance). The lack of differentiation with pores less than Concluding remarks 0.2 µm coincides with both the absence of the extracellular matrix and with the absence of cell processes invading the porous membrane. Thus, The experiments described above indicate that successful tooth development either cell-to-cell contact or the extracellular matrix could be implicated depends on complex reciprocal interactions between the dental epithelium in differentiation. Differentiation of the enamel organ and dental papilla and underlying mesenchyme. They also show that, initially, the epithelium are seen when pore size is 0.6 µm and cell processes are evident passing from the first pharyngeal arch is instructive on the underlying neural-crest- through the micropores (Fig. 21.37). However, as specialised cell contacts derived ectomesenchyme. At a later stage, however, this instructive capacity between differentiating odontoblasts and ameloblasts do not appear to is transferred to the mesenchyme, which can then induce epithelium of occur in vivo (although the processes do come very close together), it is non-first-arch origin to help form a tooth germ. Signals involving bioactive likely that the extracellular matrix may have an important role in induction. molecules (such as transcription factors, growth factors, cytokines, etc.) The extracellular matrix itself is a product of both the epithelial and the are produced in a specific spatial and temporal sequence and the cascade mesenchymal cells. of events results in a tooth consisting of the appropriate tissues and of Evidence indicating the importance of the extracellular matrix in the an appropriate shape. inductive process can be obtained from the following experiments. First, As mentioned earlier, a considerable number of genes and growth factors drugs are available that can inhibit the formation of specific components are expressed during early tooth development. It has been estimated that of the extracellular matrix: e.g., lathyrogens interfere with cross-linking more than 300 genes are involved with tooth development. A database has of collagen. When added to tissue culture medium, these drugs inhibit been established by the University of Helsinki (http://bite-it.helsinki.fi/) to allow comparisons of the expression patterns as a starting point for experimental studies. This database presently describes the expression at different stages of tooth development of growth factors, receptors, signalling molecules, transcription factors, intracellular and extracellular molecules and plasma membrane molecules. For growth factors, for example, a variety of BMPs, FGFs, Shh and TGFs are expressed at different stages of development. Epidermal growth factor (EGF) appears B to be expressed only during the bell stage of development. Neurotrophins, which are growth factors promoting neuronal growth, are also expressed at various developmental stages. Recent research has suggested that the A dental epithelium signals to the underlying mesenchymal cells via BMPs (particularly BMP-2 and BMP-4) and FGFs. Consequently, mesenchymal Fig. 21.36 The enamel organ (A) and dental papilla mesenchyme (B) cultured on either side of a porous cell proliferation and condensation are stimulated and the expression in membrane (pore size, 0.1 µm) (arrowed). No differentiation has occurred and cell processes do not pass through the membrane (toluidine blue; ×70). Courtesy Prof. I Thesleff and the editor of the Journal of Embryology and the mesenchyme of syndecan and tenascin are upregulated (syndecan Experimental Morphology. is a cell-surface heparan sulphate proteoglycan that binds to tenascin, probably influencing cell condensation). BMPs also induce the expression in the mesenchyme of Msx1 and Msx2 homeobox gene transcription factors. B Particular attention has been paid to the earliest stages of tooth development to help determine the biological mechanisms responsible for switching on the cascade of events. As previously mentioned, the activation of non-Hox homeobox genes is of crucial importance at this C time. These genes contain DNA-binding proteins that regulate gene transcription, thus controlling the expression of other genes necessary for the development of a particular structure, in this case a tooth. To date, the precise functions of such homeobox genes are not known, but they may be assumed to regulate the production of molecules important in A morphogenesis (such as growth factors, factors controlling cell division Fig. 21.37 The enamel organ (A) and dental papilla and apoptosis, cell-surface receptors, integrins and cell adhesion molecules mesenchyme (B) cultured on either side of a porous membrane (pore size, 0.6 µm). C = predentine; cell and cytoskeletal elements). The complexity of the topic is partly indicated processes from odontoblasts passing through pores in Fig. 21.38 (which contains just a selection of the molecules known are arrowed (Mallory’s trichrome stain; ×45). Courtesy Prof. I Thesleff and the editor of the Journal of to be present in the developing tooth and their number is frequently Embryology and Experimental Morphology. being added to). Evidence to support the importance of such molecules Epithelial–mesenchymal interactions during tooth development 363 Oral ectoderm Dental placode Enamel knot Pitx-2 Lef-1, Edar Edar BMP BMP BMP FGF FGF FGF Shh Shh Shh Wnt TNF Wnt Fig. 21.38 Model of the molecular regulation of tooth development from initiation to crown morphogenesis. Interactions between epithelial (green) and mesenchymal tissues (blue) are BMP BMP mediated by signal molecules (BMP = bone morphogenetic Activin FGF BMP proteins; FGF = fibroblast growth factors; Shh = sonic hedgehog; FGF FGF TNF = tumour necrosis factor). These signals operate throughout Wnt development and regulate the expression of genes in the Dental mesenchyme Condensed dental Dental papilla responding tissues (shown in boxes). Signalling centres (red) appear in the epithelium reiteratively and secrete locally many Msx-1/Msx-2 Gli-2/Gli-3 Dlx-1/Dlx-2 mesenchyme different signals that regulate morphogenesis and tooth shape. Msx-1, Pax-9, Runx-2 Courtesy Prof. I Thesleff. Box 21.1 Stem cell populations in teeth Dental pulp stem cells (DPSC) DPSCs TGPCs Periodontal ligament stem cells (PDLSC) SHED Stem cells from apical papilla (of erupting and newly erupted teeth) (SCAP) Dental follicle stem cells (DFSC) Stem cell from exfoliated deciduous teeth (SHED) in initiating tooth development can be seen in transgenic mice where, e.g., deletion of the homeobox genes such as Msx1 and Pax9 results BMSCs DFSCs in tooth development being arrested at the bud stage. Similarly, early SCAP mesenchyme isolated from the overlying epithelium can still continue POLSCs to develop in tissue culture if molecules such as BMPs and FGFs are Fig. 21.39 Diagram showing source of stem cells in the regions of the jaws and teeth. Sites of various added to the medium. Consideration also needs to be given as to how populations of dental stem cells. BMSC = bone marrow stem cells; DPSC = dental pulp stem cells; SHED = stem cells from the pulp of human exfoliate deciduous teeth; TGPC = stem cells of the tooth germ’s papilla cells. forces are generated during morphogenesis. Redrawn from Fouad SAA 2015 Dental stem cells: a perspective area in dentistry. International Journal of Dental Sciences and Research 3: 15–25. Stem cells During the development, growth and differentiation of any organ, there of r