Organic Chemistry I Lecture 5 PDF
Document Details
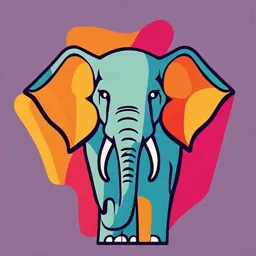
Uploaded by PanoramicGrowth
Dr. Noor Muneer
Tags
Summary
This document is a lecture on organic chemistry, focusing on alkenes. It covers their formation, naming, and key chemical properties.
Full Transcript
ORGANIC CHEMISTRY I Lec. 5 By Dr. Noor Muneer (PhD. Pharmaceutical Chemistry) 1 Alkenes: The alkenes were described as being obtained from alkanes by loss of hydrogen in the cracking process. The general formula for...
ORGANIC CHEMISTRY I Lec. 5 By Dr. Noor Muneer (PhD. Pharmaceutical Chemistry) 1 Alkenes: The alkenes were described as being obtained from alkanes by loss of hydrogen in the cracking process. The general formula for this family is CnH2n. Since alkenes evidently contain less than the maximum quantity of hydrogen, they are referred to as unsaturated hydrocarbons. The simplest member of the alkene family is ethylene, C2H4. The carbon-carbon double bond is the distinguishing feature of the alkene structure. In forming the sp2 orbitals, each carbon atom has used only two of its three p orbitals. The remaining p orbital consists of two equal lobes, one lying above and the other lying below the plane of the three sp2 orbitals; it is occupied by a single electron. If the p orbital of one carbon atom overlaps the p orbital of the other carbon atom, the electrons pair up and an additional bond is formed. Because it is formed by the overlap of p orbitals, and to distinguish it from the differently shaped σ bonds, this bond is called a π bond (pi bond). It consists of two parts, one electron cloud that lies above the plane of the atoms, and another electron cloud that lies below. Because of less overlap, the π bond is weaker than the carbon-carbon σ bond. This overlap can occur only when all six atoms lie in the same plane. Ethylene, then, is a flat molecule. The carbon-carbon "double bond" is thus made up of a strong σ bond and a weak π bond. Since the carbon atoms are held more tightly together, the C─C distance in ethylene is less than the C─C distance in ethane; that is to say, the carbon-carbon double bond is shorter than the carbon-carbon single bond. ⚫ Names of alkenes: Common names are seldom used except for three simple alkenes: ethylene, propylene, and isobutylene. The various alkenes of a given carbon number are sometimes referred to collectively as the pentylenes (amylenes), hexylenes, heptylenes, and so on. (One sometimes encounters the naming of alkenes as derivatives of ethylene: as, for example, tetramethylethylene for Most alkenes are named by the IUPAC system. The rules of the IUPAC system are: 1- Select as the parent structure the longest continuous chain that contains the carbon-carbon double bond; then consider the compound to have been derived from this structure by replacement of hydrogen by various alkyl groups. The parent structure is known as ethene, propene, butene, pentene, and so on, depending upon the number of carbon atoms; each name is derived by changing the ending -ane of the corresponding alkane name to -ene: 2. Indicate by a number the position of the double bond in the parent chain. Although the double bond involves two carbon atoms, designate its position by the number of the first doubly- bonded carbon encountered when numbering from the end of the chain nearest the double bond; thus 1-butene and 2-butene. 3- Indicate by numbers the positions of the alkyl groups attached to the parent chain. Physical properties: The alkenes possess physical properties that are essentially the same as those of the alkanes. They are insoluble in water, but quite soluble in nonpolar solvents like benzene, ether, and chloroform, the boiling point rises with increasing carbon number; as with the alkanes Preparation of alkenes Alkyl halides are converted into alkenes by dehydrohalogenation: elimination of the elements of hydrogen halide. Dehydrohalogenation involves removal of the halogen atom together with a hydrogen atom from a carbon adjacent to the one bearing the halogen. As we can see, in some cases this reaction yields a single alkene and in other cases yields a mixture For example n-Butyl chloride can eliminate hydrogen only from C-2 and hence yields only 1-butene. sec-Butyl chloride, on the other hand, can eliminate hydrogen from either C-l or C-3 and hence yields both 1- butene and 2-butene. Where the two alkenes can be formed, 2-butene is the chief product Mechanism of dehydrohalogenation: The function of hydroxide ion is to pull a hydrogen ion away from carbon; simultaneously a halide ion separates and the double bond forms. We should notice that, in contrast to free radical reactions, the breaking of the C─H and C ─X bonds occurs in an unsymmetrical fashion: hydrogen relinquishes both electrons to carbon, and halogen retains both electrons. The electrons left behind by hydrogen are now available for formation of the second bond (the π bond) between the carbon atoms Alcohols are compounds of the general formula, ROH, where R is any alkyl group and the hydroxyl group OH, is characteristic of alcohols Dehydration requires the presence of an acid and the application of heat. It is generally carried out in either of two ways: (a) heating the alcohol with sulfuric or phosphoric acid to temperatures as high as 200, or (b) passing the alcohol vapor over alumina, A12O3, at 350- 400, alumina here serving as a Lewis acid. Mechanism of dehydration of alcohols: The generally accepted mechanism for the dehydration of alcohols is summarized in the following equations; ethyl alcohol is used as the example. The alcohol unites (step 1) with a hydrogen ion to form the protonated alcohol which dissociates (step 2) into water and a carbonium ion; the carbonium ion then loses (step 3) a hydrogen ion to form the alkene The double bond is thus formed in two stages, OH being lost (as H2O) in step (2) and H being lost in step (3). Rearrangement of carbonium ions: Very often, dehydration gives alkenes that do not fit the mechanism as we have so far seen it. The double bond appears in unexpected places; sometimes the carbon skeleton is even changed. For example: Take the formation of 2-butene from n-butyl alcohol. Loss of water from the protonated alcohol gives the n-butyl carbonium ion. Loss of the proton from the carbon adjacent to the positive carbon could give 1-butene but not the 2-butene that is the major product. The other examples are similar. In each case we conclude that if, indeed, the alkene is formed from a carbonium ion, it is not the same carbonium ion that is initially formed from the alcohol. The idea of intermediate carbonium ions accounts for the facts only if we add this to the theory: a carbonium ion can rearrange to form a more stable carbonium ion. n-Butyl alcohol, for example, yields the n-butyl cation; this rearranges to the sec-butyl cation, which loses a hydrogen ion to give (predominantly) 2-butene: In a similar way, the 2-methyl-l -butyl cation rearranges to the 2- methyl-2-butyl cation, and the 3,3-dimethyl-2-butyl cation rearranges to the 2,3-dimethyl- 2-butyl cation ⚫ We notice that in each case rearrangement occurs in the way that yields the more stable carbonium ion: primary to a secondary, primary to a tertiary, or secondary to a tertiary. ⚫ Rearrangement as taking place in this way: a hydrogen atom or alkyl group migrates with a pair of electrons from an adjacent carbon to the carbon bearing the positive charge. The carbon that loses the migrating group acquires the positive charge. A migration of hydrogen with a pair of electrons is known as a hydride shift; a similar migration of an alkyl group is known as an alkyl shift. These are just two examples of the most common kind of rearrangement, the 1, 2-shifts: rearrangements in which the migrating group moves from one atom to the very next atom We can account for rearrangements in dehydration in the following way. A carbonium ion is formed by the loss of water from the protonated alcohol. If a 1, 2-shift of hydrogen or alkyl can form a more stable carbonium ion, then such a rearrangement takes place. The new carbonium ion now loses a proton to yield an alkene. In the case of the n-butyl cation, a shift of hydrogen yields the more stable sec-butyl cation; migration of an ethyl group would simply form a different n-butyl cation. In the case of the 2-methyl-l-butyl cation, a hydride shift yields a tertiary cation, and hence is preferred over a methyl shift, which would only yield a secondary cation In the case of the 3,3-dimethyl-2-butyl cation, on the other hand, a methyl shift can yield a tertiary cation and is the rearrangement that takes place. If isomeric alkenes can be formed in this step, which, if any, will predominate? The examples we have already encountered give us the answer: Reduction of an alkyne to the double-bond stage can yield either a cis-alkene or a trans-alkene. Just which isomer predominates depends upon the choice of reducing agent, except when the triple bond is at the end of a chain. Predominantly trans-alkene is obtained by reduction of alkynes with sodium or lithium in liquid ammonia. Almost entirely cis-alkene (as high as 98%) is obtained by hydrogenation of alkynes with several different catalysts: a specially prepared palladium or a nickel boride Reactions of the carbon-carbon double bond: addition The double bond consists of a strong σ bond and a weak π bond; therefore, the reaction would involve the breaking of this weaker bond. The typical reactions of the double bond are: Where the π bond is broken and two strong σ bonds are formed in its place. A reaction in which two molecules combine to yield a single molecule of product is called an addition reaction The typical reaction of an alkene is electrophilic addition, or, in other words, addition of acidic reagents. In many of its reactions the carbon-carbon double bond serves as a source of electrons: that is, it acts as a base. The compounds with which it reacts are those that are deficient in electrons, that is, are acids.. These acidic reagents that are seeking a pair of electrons are called electrophilic reagents (Greek: electron-loving). The typical reaction of an alkene is electrophilic addition, or, in other words, addition of acidic reagents Reagents of another kind, free radicals, seek electrons or, rather, seek an electron. And so we find that alkenes also undergo free- radical addition. Besides the addition reactions characteristic of the carbon-carbon double bond, alkenes may undergo the free-radical substitution characteristic of alkanes. Addition reactions: We have already encountered hydrogenation as the most useful method for preparing alkanes. The greater the number of alkyl groups attached to the doubly-bonded carbon atoms, the more stable the alkene. 2- Addition of halogens: Alkenes are readily converted by chlorine or bromine into saturated compounds that contain two atoms of halogen attached to adjacent carbons; iodine generally fails to react. 3- Addition of hydrogen halides. Markovnikov's rule: An alkene is converted by hydrogen chloride, hydrogen bromide, or hydrogen iodide into the corresponding alkyl halide Propylene could yield either of two products, the n-propyl halide or the isopropyl halide, depending upon the orientation of addition, that is, depending upon which carbon atoms the hydrogen and halogen become attached to. Actually, it is found that the isopropyl halide greatly predominates In the ionic addition of an acid to the carbon-carbon double bond of an alkene, the hydrogen of the acid attaches itself to the carbon atom that already holds the greater number of hydrogens. This statement is generally known as Markovnikov's rule. Using Markovnikov's rule, we can correctly predict the principal product of many reactions. For example: In 2-pentene each of the doubly-bonded carbons holds one hydrogen, so that according to the rule we should expect neither product to predominate. Here again the prediction is essentially correct, roughly equal quantities of the two isomers actually being obtained. The examples have involved the addition of hydrogen iodide; exactly similar results are obtained in the addition of hydrogen chloride, except for special conditions indicated in the following section, of hydrogen bromide. Addition of hydrogen bromide. Peroxide effect: Addition of hydrogen chloride and hydrogen iodide to alkenes follows Markovnikov's rule. Addition of hydrogen bromide to a particular alkene yields a product in agreement with Markovnikov's rule; by others, a product in contradiction to Markovnikov's rule; and by still others, a mixture of both products. The orientation of addition of hydrogen bromide to the carbon-carbon double bond is determined by the presence or absence of peroxides. Organic peroxides are compounds containing the ─O─O─ linkage. Certain peroxides are deliberately synthesized, and used as reagents, if one deliberately puts peroxides into the reaction system, HBr adds to alkenes in exactly the reverse direction. This reversal of the orientation of addition caused by the presence of peroxides is known as the peroxide effect. Of the reactions we are studying, only the addition of hydrogen bromide shows the peroxide effect. The presence or absence of peroxides has no effect on the orientation of addition of hydrogen chloride, hydrogen iodide, sulfuric acid, water, etc.