HSS2305 Molecular Mechanisms of Disease Lecture Notes PDF
Document Details
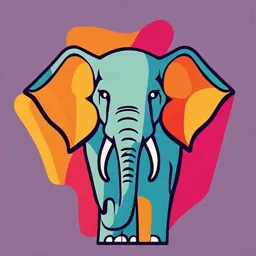
Uploaded by RockStarArlington
University of Ottawa
Prof. Keir Menzies
Tags
Related
Summary
These lecture notes cover bioenergetics, enzymes, and metabolism. Sample questions and concepts are included.
Full Transcript
HSS2305: Molecular Mechanisms of Disease Lecture 4 – Bioenergetics, Enzymes and Metabolism Prof. Keir Menzies Bioenergetics and Enzymes Today Outline for Today Sample Questions Bioenergetics, Enzymes and Metabolism Sample Questions 1. What mac...
HSS2305: Molecular Mechanisms of Disease Lecture 4 – Bioenergetics, Enzymes and Metabolism Prof. Keir Menzies Bioenergetics and Enzymes Today Outline for Today Sample Questions Bioenergetics, Enzymes and Metabolism Sample Questions 1. What macromolecule contains a sugar backbone: a) Ribonucleic Acid (RNA) b) Proteins c) Triglycerides d) Phospholipids 2. What is not a unique feature of DNA when compared to RNA: a) Contains Thymine b) Double stranded c) Occurs only in the nucleus d) Contains a phosphate group 3. What type of amino acids can form ionic bonds: a) Polar uncharged b) Nonpolar c) Polar charged d) Essential 4. Which amino acid has only a Hydrogen in its sidechain and can fit in either a hydrophilic or hydrophobic environment? a) Proline b) Cysteine c) Histidine d) Glycine Application 5. How do amino acids like hydroxylysine and thyroxine, which are not among the 20 amino acids inserted into amino acid chains, get into proteins? a) They are inserted due to mutations. b) They are the result of the alteration of R groups of the 20 amino acids after their incorporation into the polypeptide. c) They occur as carboxyl groups are altered, reversing the chirality of the molecules. d) Insertion of the amino acid into the polypeptide causes bonds to break and new amino acids to form. Bioenergetics A living cell bustles with activity A cell must acquire and expend energy http://www.ted.com/ Bioenergetics = Study of energy transformations in a living organism Energy: capacity to do work; change or move things Thermodynamics: study of changes of energy What is the Biomed Relevance? Understanding chemical reactions requires basic comprehension of physics, chemistry properties Understand different conditions can make interactions between molecules more or less favorable (Molecules randomly bouncing around) By adding energy to the system (ATP) it can drive chemical reactions in a particular direction, yielding specific biological products First Law of Thermodynamics Conservation of energy Energy can be neither created or destroyed but can be converted into different forms (i.e. transduction) I.e. Energy in universe/closed system is constant (no increase or decrease) o E.g. Chemical energy in ATP → mechanical energy for organelles to move about the cell E = Q - W o ∆E = change in internal energy Chemical energy is converted o Q = heat (energy in/out) to mechanical and thermal o W = work (energy in/out) energy E>0 = Endothermic -> reactions that GAIN heat E reactions that LOSE heat The Second Law of Thermodynamics In any natural process, the total entropy (measure of randomness or disorder) of a closed system will increase or remain constant, but never decrease; energy tends to spread out and systems naturally evolve towards a state of greater randomness or disorder Entropy (S) Entropy - measure of Highly Ordered Highly Random randomness or disorder Less Entropy More Entropy it also reflects the tendency of energy to spread out over time in isolated systems. Every event is accompanied by an increase in the entropy of the universe living systems maintain a state of ‘system’ order (low entropy) at the expense of higher ‘universe’ disorder (high entropy) → i.e. metabolism H2O (l) -> H2O (g) >S Free-Energy Changes in Chemical RATES OF ReactionsCHEMICAL REACTIONS All chemical reactions are theoretically reversible A+B→C+D The rates of chemical reactions are proportional to the concentration of reactants and sometimes: Temperature pH Pressure Location within cell All chemical reactions spontaneously proceed toward equilibrium (Keq = [C][D]/[A][B]) At equilibrium, the “free energies” of the products and reactants are equal However, we cannot make wood from ashes: C6H10O5(cellulose)+O2→CO2+H2 O+ash Equilibrium constant (Keq) approaches infinity GIBBS FREE ENERGY Gibbs Free energy (G) = is a thermodynamic quantity that represents the maximum amount of work that can be performed by a system at constant temperature and pressure (a.k.a. available energy) Used to predict whether a chemical reaction or process will occur spontaneously ∆G = ∆H – (T x ∆S) ∆H = is the change in enthalpy (heat content), Sum of the internal energy (E) and product of the pressure (P) and volume (V) of a thermodynamic system H= E+ PxV T = Temperature in kelvin (absolute zero is 0 K and -273.15 °C; convert Celsius to kelvin by adding 273.15) ∆S = Entropy http://youtu.be/DPjMPeU5OeM GIBBS FREE ENERGY Gibbs free energy equation tells us if a system is at equilibrium ΔG=0 or if a reaction will occur (ΔG≠0) G is negative→ exergonic, reaction will be spontaneous - towards state of lower energy G is positive→ endergonic, cannot occur spontaneously, require energy input or coupling EA = Activation Energy – determines the kinetics (rate) of the reaction A higher activation energy means the reaction will occur more slowly because fewer molecules will have sufficient energy to overcome the energy barrier. While Gibbs free energy tells you whether a reaction will occur, activation energy tells you how quickly or easily the reaction will start. G0 GIBBS FREE ENERGY Questions ∆G = ∆H – (T x ∆S) 1. Which one of the following process has ΔS (Entropy) > 0? a) 2H2(g) + O2(g) --> 2H2O(g) b) 2NO2(g) --> N2O4(g) c) BaF2(s) --> Ba2+(aq) + 2F–(aq) d) CO2(g) --> CO2(s) 2. Consider a process for which ΔH (Enthalpy) = 211 kJ and ΔS (Entropy) = -57 J/K. How will raising the temperature affect ΔG for this process? a) ΔG will increase b) ΔG will decrease c) ΔG will not change d) The effect on ΔG cannot be predicted from the information given. Bioenergetics Equilibrium vs. steady state Equilibrium and steady state are both conditions in systems(reactions) where certain variables remain constant over time, but they differ in how this constancy is achieved. Equilibrium In a chemical reaction like A⇌B, equilibrium is reached when the concentration of A and B remains constant, with the forward and reverse reactions happening at the same rate. No energy required (ΔG=0); Occurs in closed systems with no external inputs. Steady state In a steady-state system, the variables (such as concentrations of reactants and products) remain constant over time, but this constancy is due to a continuous input and output of materials or energy. Requires energy (ΔG≠0) Occurs in open systems with continuous inputs and outputs. E.g. The ‘steady-state’ intracellular Na+ concentration is very low. This is maintained by the Na+/K+ ATPase which requires ATP energy. Homeostais in Biology Homeostasis in biology steady state plays a crucial role in maintaining homeostasis, which is the process by which living organisms regulate their internal environment to maintain stable i.e. Intracellular ion distributions, osmotic balance, intracellular pH etc. maintained in spite of changing extracellular conditions for cell survival Example: Cellular metabolism is NOT at equilibrium, instead are at STEADY STATE Open thermodynamic system –exchange of matter between system and surroundings New substrates enter and products are removed Maintaining a steady state requires a constant input of energy However, at death, biochemicals will redistribute according to equilibrium in movements that do not require energy (b) Steady state versus Another way of saying this: Cells exist in a dynamic equilibrium in the amoeba nonequilibrium →rates of forward and reverse reactions can be increased or decreased in response Chemical Reactions - ATP One of the most important chemical reactions in a cell is the hydrolysis of ATP Adenosine triphosphate present all parts of the cell Synthesized in cytoplasm/mitochondria End product of glycolysis/cellular respiration http://bioap.wikispaces.com/file/view/c9x6cell-respiration.jpg http://1.bp.blogspot.com/-IirWRJZGmsQ/UOSWw3x5ZhI/AAAAAAAAA8Y/nqc_WVSjBHU/s1600/ATP.jpg Chemical Reactions - ATP Liberation of one phosphate group produces ADP and energy G is negative G is negative =Exergonic Chemical Reactions - ATP In a cell Energy from “downhill” ATP hydrolysis reaction (exergonic) Drive most energy requiring (endergonic) “uphill” chemical reactions/processes Ex. Separation of charge across a membrane Properties of proteins Movement of filaments in a muscle cell Free energy in glycolysis and the TCA cycle Free energy in Glycolysis and TCA cycle G is positive G is negative Entire reaction: G is negative Rates of Chemical Reactions Dependent on relative concentrations of reagents: The concentration of reactants determines how frequently reactant molecules collide and have the opportunity to react Dependant on EA = Activation Energy - the minimum energy barrier that the reactants must overcome for a reaction to occur May also depend on environmental conditions Temperature pH Pressure Location within cell Can be altered by enzymes Presence of enzyme cofactors or catalysts Enzymes as Biological Catalysts Enzymes are catalysts that speed up chemical reactions Enzymes lower the EA barrier Not permanently altered during the course of a reaction Only affect reaction rates Required for metabolism Present in cells in small amounts Enzymes as Biological Catalysts Enzymes: Are almost always proteins Exception - RNA catalyst molecules called Ribozyme May contain non-protein components: Cofactors are inorganic enzyme conjugates Metal ions (iron, magnesium, copper, zinc) Coenzymes are organic enzyme conjugates Vitamins, NAD, FAD, ATP Are highly regulated Expression levels, modifications, etc. Enzymes as Biological Catalysts Example:Pyruvate dehydrogenase Multienzyme complex Junction of glycolysis and the TCA cycle Requires five organic coenzymes and one metal ion cofactor Coenzymes: Thiamine pyrophosphate (TPP) Lipoamide (covalently bound) Flavin adenine dinucleotide (FAD) Nicotinamide adenine dinucleotide (NAD+) Coenzyme A (CoA) Cofactor: Magnesium ion (Mg2+). Modifications Phosphorylation at specific sites by Pyruvate Dehydrogenase Kinase (PDK) inactivates enzyme Enzymes as Biological Catalysts The Active Site PK Enzymes (E) bind specific PEP + ADP + Pi ATP + Pyruvate substrates (S) at the active Reactants/ Products site Substrates Substrate (Key) Enzyme (Lock) E+S Forms Enzyme-Substrate ES (ES) Complex Lock Turning Products (P) released and E+P Enzyme returned to original state Formation of the Pyruvate Kinase (PK) enzyme–substrate complex: pyruvate kinase, PEP, and ADP Enzymes as Biological Catalysts Overcoming Activation Energy Barrier Activation energy (EA) is required for any chemical transformation Substrates/ Enzyme reduces EA required E = Hexokinase (HK) Reactant molecules form very unstable molecule called a transition state at peak of EA Glucose + Pi -> Glucose 6-Phoshpate: ΔG°′= +3.3 kcal/mol (unfavorable reaction) ATP -> Pi + ADP: ΔG°′= -7.3 kcal/mol Free energy for entire recation: Glucose + ATP -> Glucose 6-Phoshpate: ΔG°′= -4.0 kcal/mol HK favorable reaction Enzymes as Biological Catalysts Overcoming Activation Energy Barrier Catalyst No Catalyst No enzyme Few substrate molecules reach the transition state (pink) at a given temp With Enzyme Large proportion of substrate molecules reach the transition state (blue) With Increased Temperature (T) Decrease in delta G (if entropy is positive) More molecules form transition state and reach EA Remember: The effect of lowering activation ∆G = ∆H – (T x ∆S) energy on the rate of a reaction. Limitations: Free Energy (G) can either increase or decrease for a https://www.khanacademy.org/science/biology/energy- reaction when the temperature increases. It depends on the entropy (S) change (also too high of a temp can denature and-enzymes/free-energy-tutorial/v/gibbs-free-energy- proteins and slow a reaction if thinking about biology). and-spontaneous-reactions Enzymes as Biological Catalysts Mechanism 1. Substrate Orientation Multiple substrates close proximity Substates correct in favorable orientation 2. Changing Substrate Reactivity Amino acids in active site alter chemical properties (e.g., charge) of substrate 3. Inducing Strain in the Substrate Enzyme changes conformation of substrate to bring closer to conformation of transition state Enzymes as Biological Catalysts Mechanism Changing Substrate Reactivity/Stabilize Transition States Acidic or basic R groups on the enzyme may change the charge of the substrate. Charged R groups may attract the substrate. Cofactors of the enzyme increase the reactivity of the substrate by removing or donating electrons. Example: Chymotrypsin Proteolytic enzyme: Chymotrypsin cleaves at the aromatic amino acids phenylalanine, tryptophan, and tyrosine Digestive system Cleaves peptide bonds Occurs naturally but very slowly Chymotrypsin lowers EA and increases reaction Picture from : https://www.worthington-biochem.com/products/chymotrypsin/manual Enzymes as Biological Catalysts Mechanism Inducing strain in the substrate Enzyme (lock) changes when substrate (S) binds i.e. conformational change Called “Induced Fit Model” Strains covalent bonds in substrate Conformational change of hexokinase when a glucose molecule binds – protein undergoes a conformational change that encloses the substrate Enzymes as Biological Catalysts Mechanism How do we identify conformational changes and catalytic intermediates Various changes in atomic and electronic structure occur in both the enzyme and substrate during a reaction. Using time-resolved crystallography, researchers have determined the three- dimensional structure of an enzyme at successive Electron density stages during a reaction Myoglobin: structure by map of a single time resolved X-ray hydrogen bond crystallography. Enzymes as Biological Catalysts Enzyme Kinetics Kinetics Study of rates of enzymatic reactions under various experimental conditions Reaction rates increase with increasing substrate concentrations until the enzyme is saturated Saturation Every enzyme (lock) is binding substrate (key) Working at maximum capacity More substrate (keys) does not increase rate of product formation. No more locks! Maximal velocity (Vmax) Reaction speed (velocity) at saturation Turnover number Number of substrate molecules converted to product per second per enzyme molecule at Vmax Ranges of 1 → 103 typical (at saturation 1 molecule of substrate/second/molecule of enzyme) Enzymes as Biological Catalysts Enzyme Kinetics Michaelis-Menten model Michaelis-Menten plots Michaelis Constant (KM) = substrate concentration when the reaction is at half-maximal velocity (Vmax/2) Michaelis constant (KM) is used to describe the affinity of the enzyme for the substrate Note that: Temperature: Changes the steepness of the curve and Vmax, depending on whether the temperature is optimal or not. pH: Affects the shape of the curve by altering Vmax and KM depending on how close the pH is to the enzyme’s optimal pH. Enzymes as Biological Catalysts Enzyme Kinetics Temperature and pH can affect enzymatic reaction rates Each enzyme is most active under specific conditions pH Optimize amino acid R group charge May improve transition state stabilization Too much -> Denaturing of enzyme Not all enzymes denature at the same pH Different Amino Acid sequences -> different properties Temperature decreases ∆G (if entropy is positive) Too much -> Denaturing of enzyme Not all enzymes denature at the same temperature Usually an optimum temperature for each enzyme. Enzymes as Biological Catalysts Enzyme Inhibitors Enzyme inhibitors - slow the rates for enzymatic reactions Irreversible inhibitors bind tightly to the enzyme (could be a covalent bond Reversible inhibitors bind loosely to the enzyme https://youtu.be/Sl_JDjsssIM Enzymes as Biological Catalysts Enzyme Inhibitors and Allosteric Inhibitors 1. Competitive inhibitors: Compete with substrate for active site Resemble the substrate structure Overcome with high substrate/inhibitor ratios Reversible Km increased Vmax not altered 2. Noncompetitive inhibitors: Bind to sites other than active sites Vmax reduced Km not altered Usually, reversible Most Pharmaceuticals are allosteric noncompetitive inhibitors: Enzymes as Biological Catalysts Metabolic regulation Cellular activity is highly regulated End product can inhibit the enzyme that helped create that product --> Enzymes are controlled by alterations in active or allosteric sites Covalent modification of enzymes with addition of phosphate group (P) → protein kinases Non-competitive Allosteric modulation of enzymes by inhibition compounds binding to allosteric sites End i.e. End Product inhibition → Product product of pathway inhibits inhibition enzymes of the pathway (example of feedback regulation) Questions? Next Lecture Bioenergetics, Enzymes and Metabolism (Ch. 3) cont. HSS2305: Molecular Mechanisms of Disease Lecture 5 –Metabolism Today’s Outline Sample Questions Bioenergetics, Enzymes and Metabolism Announcement Rare Disease Assignment Topics 1st choice you made was accepted: please do not change Deadline Nov 5 at 11:59 PM Lets explore enzymes! A few examples of how enzymes are important: As Targets for Antibiotics for Bacteria For Active Transport in the Cell (steady state vs. equilibrium) For Metabolism Human Perspective antibiotics -enzyme inhibitors Antibiotics – Enzyme Inhibitor Pharmaceuticals: Target enzymes involved in: Synthesis of the bacterial cell wall I.e: penicillin, vancomycin Components of bacteria duplication, transcription, and translation Quinolone (i.e. Cipro/Ciprofloxacin) – targets bacterial DNA Gyrase (releases strain on DNA during replication) Bacteria-specific metabolic reactions specific to bacteria Sulfa drugs – resembles PABA (natural product used in sunscreen) →PABA is required for synthesis of folic acid coenzyme (bacteria must synthesize folic acid-therefore Sulfa drugs competitively inhibit this reaction) Human Perspective antibiotic resistance Antibiotics have been misused with dire consequences Susceptible cells are destroyed, leaving rare and resistant cells to survive and replicate if full dose of antibiotics are not used Bacteria become resistant to antibiotics by acquiring genes from other bacteria by various mechanisms Enzymes: Active Transport Active transport is the pumping of molecules or ions through a membrane against their concentration gradient. Example of steady state (cell metabolism) vs. equilibrium (death) Without enzymes or ATP is this thermodynamically favourable? It requires: Transmembrane protein ATP The energy of ATP may be used directly or indirectly Direct Active Transport → ATP binds directly to transporter and energy from ATP hydrolysis drives transport across a membrane Active Transport http://www.au.dk/fileadmin/www.au.dk/forskning/nobelprisen_i_kemi_1997/natrium-kalium-pumpen/cel The Na+/K+ ATPase Cellular cytoplasm → very high [K+], very low [Na+] This ionic distribution is crucial for maintaining the cell’s resting membrane potential Gradients established by Na+/K+ ATPase Uses energy from the hydrolysis of ATP 3 Na+ ions pumped OUT of cell 2 K+ ions pumped INTO cell Almost one-third of all the ATP energy in animal cells used on Na+/K+ ATPase In neurons it take up about two- thirds of the cells ATP Active Transport Na+/K+ ATPase pump inhibition- Digoxin The Na+/K+ ATPase and Congestive Heart Failure- treatment is to increase the heart’s force of contraction: Na+/K+ Sodium ATPase calcium pump exchanger How digoxin corrects heart failure: Digoxin is a cardiac glycoside that acts as ionotropic agent that increases the force of contraction of the heart -digoxin only partially inhibits the pump -because it affects the Na⁺/K⁺ pump in other cells, digoxin can have systemic side effects, such as gastrointestinal disturbances, neurological symptoms, and arrhythmias Metabolism Metabolism Metabolism Collection of biochemical reactions that occur within a cell Metabolic pathways are sequences of chemical reactions Pathways convert substrates into end products via a series of metabolic intermediates Each reaction in the sequence is catalyzed by a specific enzyme Pathways are usually confined to specific subcellular locations (i.e. glycolysis in the cytoplasm) Anabolism Collection of biochemical reactions that build new molecules within a cell Catabolism Collection of biochemical reactions that degrade molecules within a cell Metabolism Catabolic pathways break down complex substrates into simple end products Provide raw materials for the cell (e.g. amino acids) Provide chemical energy for the cell (i.e. ATP) Anabolic pathways synthesize complex end products from simple substrates Require energy (i.e. ATP and NADPH from catabolic pathways) Use raw materials to build macromolecules (e.g. amino acids to form proteins) Energy Metabolism Simplified Macromolecules Intermediates Waste Example of three stages of energy metabolism Green Arrows = Catabolic Reactions Blue Arrows – Anabolic Reactions Stage I: Macromolecules hydrolyzed into building blocks (ex. monomers like glucose, etc.) Stage II Building blocks degraded into interchangeable metabolites (i.e. metabolic intermediates) Stage III Small molecular weight metabolites are degraded yielding products (e.x. Acetyl-CoA -> ATP) Metabolic pathways for nucleic acids are more complex and are not shown here Metabolism oxidation-reduction Oxidation-Reduction (Redox) reactions involve a change in the electron numbers of reactants Substrate gains electrons → Reduced GER – Gain of Electrons is Reduction Substrate loses electrons → Oxidized LEO – Loss of Electrons is Oxidation Electrons must be donated/accepted from other molecules Electron gaining molecule is Oxidizing Agent Electron donating molecule is Reducing Agent Reduced atoms can then be oxidized, releasing energy to do work Metabolism oxidation-reduction Metabolism oxidation-reduction Metabolism is highly dependent on oxidation- reduction reactions Ex. NAD+ and NADH Catabolic processes (i.e. break down of glucose) transfer electrons to NAD+ to form NADH NADH used to form ATP Anabolic processes use electrons from NADPH (NADPH is created from NADH) to form macromolecules Oxidized State Reduced State Low Energetic State High Energetic State Metabolism capture (harvesting) and utilization of energy 2 Metabolism capture (harvesting) and utilization of energy Major Pathways: Glycolysis Occurs in cytosol Metabolizes Glycose and other sugars Tricarboxylic Acid Cycle (TCA) a.k.a. Kreb’s cycle or Citric Acid Cycle Occurs in mitochondrial matrix Metabolizes Acetyl-CoA (derived from Pyruvate or Fatty Acids) Electron Transport Chain Occurs in inner mitochondrial membrane Converts NADH/FADH2 (highly reduced molecules) to ATP Glycolysis Glycolysis http://cfcc.edu/faculty/dnorris/pdf/glycolysis.jpg Metabolizes monosaccharides Investment Phase Primarily glucose Produces: Net 2 ATPs/glucose 2 NADH/glucose Used to make more ATP 2 Pyruvate/glucose Used to make more NADH/FADH2 and then Or to fermentation more ATP Anaerobic pathway Requires no O2 End product is pyruvate Catabolized aerobically or anaerobically Payoff Phase Or to fermentation Investment Phase Glycolysis Keep in mind that two molecules of glyceraldehyde 3-phosphate are formed from Glycolysis each molecule of glucose, so reactions 6 through 10 shown here occur twice per glucose molecule oxidized (as denoted in the diagram and text here). Payoff Phase Anaerobic metabolism of Pyruvate Anaerobic metabolism of Pyruvate Fermentation = anaerobic reduction of pyruvate Why? Glycolysis depletes the supply of NAD+ by reducing it to NADH Fermentation oxidizes NADH back to NAD+ by reducing pyruvate In muscle and tumor cells for example pyruvate → lactate In yeast and other microbes pyruvate → ethanol Fermentation is inefficient with only about 8% of the energy of glucose captured as ATP https://youtu.be/7Hr4Eyi4-mo?t=243 https://www.youtube.com/watch?v=8Y_FdjI2v4I (some errors in this one but kind of funny) Anaerobic metabolism of Pyruvate Why? Glycolysis requires NAD+ If no O2 Electron Transport Chain (ETC) can’t use NADH NAD+ levels decrease Glycolysis slows Fermentation Pyruvate + NADH -> Lactate + NAD+ NAD+ used in glycolysis Aerobic metabolism of Pyruvate Anabolic Metabolism Examples of what we used reducing power for: Anabolic pathways require a source of electrons Anabolic Metabolism reducing power NADPH and NADH are interconvertible Different metabolic roles: NAD+ is reduced to NADH in catabolic pathways Then used to form ATP NADPH is oxidized to NADP+ in anabolic pathways Transhydrogenase transfers hydrogen atoms and electrons from NADH + NADP+ NAD+ + NADPH – NADPH is favored when energy is abundant – NADH is used to make ATP when energy is scarce Questions? Next Lecture Aerobic Respiration (Ch. 5)