Histology Final PDF
Document Details
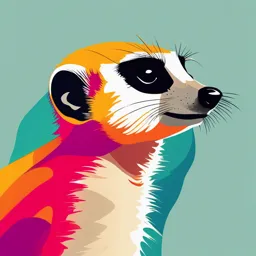
Uploaded by SpiritualHonor
College of Medicine
Tags
Summary
This document contains notes on cell biology topics, such as the structures and functions of cells, specifically focusing on the nucleus and cell cycle. It includes diagrams and illustrations to help explain the concepts.
Full Transcript
8 Nucleus and phases of cell cycle ILOs By the end of this lecture, students will be able to 1. Correlate structure of different components to the nucleus to its function. 2. Differentiate between functional forms of the chromatin. 3. Interpret structural organization...
8 Nucleus and phases of cell cycle ILOs By the end of this lecture, students will be able to 1. Correlate structure of different components to the nucleus to its function. 2. Differentiate between functional forms of the chromatin. 3. Interpret structural organization of the chromosome. 4. Discuss the nuclear and cellular changes during the phases of the cell cycle The Cell Nucleus: Introduction The nucleus contains a blueprint for all cell structures and activities, encoded in the DNA of the chromosomes. It also contains the molecular machinery to replicate its DNA and to synthesize and process the three types of RNA; ribosomal (rRNA), messenger (mRNA), and transfer (tRNA). (Are there DNA in the cell outside the nucleus?) The nucleus does not produce proteins; the numerous protein molecules needed for the activities of the nucleus are imported from the cytoplasm. Structure of the nucleus as seen by LM The nucleus frequently appears as a rounded or elongated structure, usually in the center of the cell (Figure.1A). Its main components are the nuclear envelope, chromatin, nucleolus, and nuclear matrix (Figure.1B). The size and morphological features of nuclei in a specific normal tissue tend to be uniform. In common hematoxylin and eosin-stained preparations; the nucleus, however, appears intensely stained dark blue or black. (Why?) Ultrastructure of the nucleus Three components are recognized: 1. Nuclear Envelope Electron microscopy shows that the nucleus is surrounded by two parallel membranes separated by a narrow space called the perinuclear cisterna. Together, the paired membranes and the intervening space make up the nuclear envelope. (Fig 1B) Polyribosomes are attached to the outer membrane, showing that the nuclear envelope is a in continuity with the endoplasmic reticulum. (Why?) At sites at which the inner and outer membranes of the nuclear envelope fuse, there are gaps, the nuclear pores (Figure 1B), that provide controlled pathways between the nucleus and the cytoplasm. Because the nuclear envelope is impermeable to ions and molecules of all sizes, the exchange of substances between the nucleus and the cytoplasm is made only through the nuclear pores. Ions and Page 1 of 5 molecules with a diameter up to 9 nm pass freely through the nuclear pore without consuming energy. But molecules and molecular complexes larger than 9 nm are transported by an active process, mediated by receptors, which uses energy from adenosine triphosphate (ATP). 2. Chromatin o Chromatin is composed mainly of coiled strands DNA bound to basic proteins (histones). o The basic structural unit of chromatin is the nucleosome (Figure 2), which consists of a core of four types of histones, wrapped around DNA base pairs. (What is the role of histones?) o Linker DNA; An additional DNA segment forms a link between adjacent nucleosomes, and another type of histone is bound to this DNA. This organization of chromatin has been referred to as "beads-on-a-string." Nonhistone proteins are also associated with chromatin, but their arrangement is less well understood. o Functional forms of Chromatin; in nondividing nuclei, is in fact the chromosomes in a different degree of uncoiling. According to the degree of chromosome condensation, two types of chromatin can be distinguished with both the light and electron microscopes (Figure 3). Heterochromatin (Gr. heteros, other, + chroma, color), which is electron dense, appears as coarse granules in the electron microscope and as basophilic clumps in the light microscope. It represents the inactive form of chromatin and acts as a reserve in less active cells. Euchromatin is the less coiled portion of the chromosomes, visible as a finely dispersed granular material in the electron microscope and as lightly stained basophilic areas in the light microscope. It represents the active form of chromatin and more abundant in active cells. The proportion of heterochromatin to euchromatin accounts for the light-to-dark appearance of nuclei 0in tissue sections as seen in light and electron microscopes. The intensity of nuclear staining of the chromatin is frequently used interpret the functional state of the nucleus. (How?) Figure 2 Nucleosome structure Page 2 of 5 Figure 3 Electron micrograph of a nucleus showing the heterochromatin (HC) and euchromatin (EC). Unlabeled arrows indicate the nucleolus-associated chromatin around the nucleolus (NU). Arrowheads indicate the perinuclear cisterna. Underneath the cisterna is a layer of heterochromatin, the main component of the so-called nuclear membrane seen under the light microscope. X 26,000. Careful study of the chromatin of mammalian cell nuclei reveals a heterochromatin mass that is frequently observed in female cells but not in male cells. This chromatin clump is the sex chromatin and is one of the two X chromosomes present in female cells. The X chromosome that constitutes the sex chromatin remains tightly coiled and visible, whereas the other X chromosome is uncoiled and not visible. Evidence suggests that the sex chromatin is genetically inactive. The male has one X chromosome and one Y chromosome as sex determinants; the X chromosome is uncoiled, and therefore no sex chromatin is visible. In human epithelial cells, sex chromatin appears as a small granule attached to the nuclear envelope. The cells lining the internal surface of the cheek are frequently used to study sex chromatin. Blood smears are also often used, in which case the sex chromatin appears as a drumstick-like appendage to the nuclei of the neutrophilic leukocytes. 3. Nucleolus o The nucleolus is a spherical structure (Figure 17-5) that is rich in rRNA and protein. It is usually basophilic when stained with hematoxylin and eosin. o Significance of the nucleolus; it contains DNA that codes for rRNA (type of RNA present inside ribosomes). The nucleolus is the site of synthesis of ribosomal subunits (to be explained later). Ribosomal proteins, synthesized in the cytoplasm, become associated with rRNAs in the nucleolus; ribosome subunits then migrate into the cytoplasm. Heterochromatin is often attached to the nucleolus (nucleolus-associated chromatin), but the functional significance of the association is not known. The rRNAs are synthesized and modified inside the nucleus. In the nucleolus they receive proteins and are organized into small and large ribosomal subunits, which migrate to the cytoplasm through the nuclear pores. (Figure 17-4) Page 3 of 5 4. Nucleoplasm: The protoplasm within the nucleus, consisting of a fluid portion, a proteinaceous matrix, and various ribonucleoproteins particles. Nuclear & cellular changes during cell cycle: Cell cycle The cell cycle is a series of events within the cell that prepare the cell for dividing into two daughter cells. Phases of the cell cycle: I- Interphase; long period of time during which the cell increases its size and content and replicates its genetic material. It includes three stages: a) G1 (gap) phase, synthesis of macromolecules essential for DNA duplication begins & cell growth. b) S (synthetic) phase, DNA is duplicated. c) G2 phase, the cell undergoes preparations for mitosis. II- Mitosis, a shorter period of time during which the cell divides its nucleus and cytoplasm, giving rise to two daughter cells. The cell cycle may be thought of as beginning at the conclusion of the telophase stage in mitosis, after which the cell enters interphase. (Figure 6) The interphase Gap 1 Daughter cells formed during mitosis enter the G1 phase. During this phase, the cells synthesize RNA, regulatory proteins essential to DNA replication, and enzymes necessary to carry out these synthetic activities. Thus, cell growth occurs restoring cell size to normal. The centrioles (a cell organelle involved in cell division) begin to duplicate themselves, a process that is completed by the G2 phase. S Phase During the S phase, the synthetic phase of the cell cycle, the genome is duplicated. The cell now contains twice the normal complement of its DNA. Autosomal cells contain the diploid amount of DNA before the synthetic (S) phase of the cell cycle that becomes doubled after S phase in preparation for cell division. Page 4 of 5 G2 Phase The gap 2 phase (G2 phase) is the period between the end of DNA synthesis and the beginning of mitosis. During the G2 phase, the RNA and proteins essential to cell division are synthesized, the energy for mitosis is stored. Duplication of centrioles and formation of the needed microtubules are completed. DNA replication is analyzed for possible errors, and any of these errors is corrected. Cells that become highly differentiated (What is meant by differentiation?) after the last mitotic event may stop to undergo mitosis either permanently (e.g., neurons, muscle cells) or temporarily (e.g., peripheral lymphocytes) and return to the cell cycle at a later time. Cells that have left the cell cycle are said to be in a resting stage, the G0 (outside) phase, or the stable phase. Figure 6. The cell cycle in actively dividing cells. Nondividing cells, such as neurons, leave the cycle to enter the G0 phase (resting stage). Other cells, such as lymphocytes, may return to the cell cycle. Page 5 of 5 13 Cytoskeletal microtubules & Microfilaments & motility ILOs By the end of this lecture, students will be able to 1. Correlate the molecular organization of microtubules to its dynamic nature. 2. Interpret structural adaptation of MT to their function. 3. Correlate the relevant motor proteins in cell trafficking to MT. 4. Appraise the importance of microtubules as a target for drug action. 5. Correlate molecular structure of actin to its function. Cytoskeleton The cytoplasm of animal cells contains a cytoskeleton, an intricate three-dimensional meshwork of protein filaments that are responsible for the maintenance of cell shape (Fig 1). Additionally, the cytoskeleton is an active participant in cellular motion, whether of organelles or vesicles within the cytoplasm, regions of the cell, or the entire cell. The cytoskeleton has three components: thin filaments (microfilaments), intermediate filaments, and microtubules. Fig. 1 The cytoskeleton Microtubules Microtubules (MTs) are long, straight, hollow structures that act as intracellular pathways. The centrosome is a region close to the nucleus that houses the centrioles, as well as several hundred ring-shaped γ-tubulin ring complex molecules that act as nucleation sites for microtubules (fig. 2). Microtubules are dynamic structures that frequently change their length by undergoing growth spurts and then becoming shorter; both processes occur at the plus ends (oriented away from the nucleus), so that the average half-life of a microtubule is only about 10 minutes (fig. 2-B). Molecular structure of MTS Each microtubule consists of 13 parallel protofilaments composed of heterodimers of the globular 1 polypeptide α- and β-tubulin subunits. Fig. 2 Molecular structure of MTs A B Functions of MTs Provide rigidity and maintain cell shape. Regulate intracellular movement of organelles and vesicles. Establish intracellular compartments. Provide the capability of ciliary and flagellar (tail of the sperm) motion. During cell division, rapid polymerization of existing, as well as, new microtubules is responsible for the formation of the mitotic spindle. Clinical correlation The dynamic process of microtubule formation is disrupted by some drugs which can have different clinical effects on cellular functions e.g. Colchicine: By binding to the tubulin molecules in leukocytes, colchicine prevents its polymerization into microtubules. It thus interferes with leukocyte migration, phagocytosis and further release of inflammatory mediators, and this is the basis for its anti-inflammatory effect in acute gouty arthritis (Joint inflammation due to precipitation of urate crystals). Microtubule-associated proteins Microtubule-associated proteins are motor proteins that assist in the translocation of organelles and vesicles inside the cell. 2 Their primary functions are to prevent depolymerization of microtubules and to assist in the intracellular movement of organelles and vesicles. Movement along a microtubule occurs in both directions and is toward both the plus end and the minus end. The two major families of microtubule motor proteins, the MAPs dynein and kinesin, bind to the microtubule as well as to vesicles and organelles In the presence of ATP, dynein moves the vesicle toward the minus end of the microtubule. Kinesin effects vesicular (and organelle) transport in the opposite direction, toward the plus end. Centrioles Centrioles are small, cylindrical structures composed of nine microtubule triplets; they constitute the core of the microtubule organizing center or the centrosome. They are paired structures, arranged perpendicular to each other, and are located in the microtubule organizing center, the centrosome, in the vicinity of the Golgi apparatus. Functions of the centriole The centrosome assists in the formation and organization of microtubules as well as in its self- duplication before cell division. During cell division, centrioles are responsible for the formation of the spindle apparatus. Additionally, centrioles are the basal bodies that guide the formation of cilia and flagella (motile cell processes). 3 Fig. 5 Cilia & Flagellum Actin Filaments (microfilaments) Thin filaments (microfilaments) are composed of two chains of globular subunits (G-actin) coiled around each other to form a filamentous protein, F-actin. Thin filaments are 6-nm thick and possess a faster-growing plus end and a slower-growing minus end. Functional forms of actin 1. Contractile bundles: Their actin filaments are arranged loosely, parallel to each other, with the plus and minus ends alternating in direction. They form cleavage furrows (contractile rings) during mitotic division. Movement of organelles and vesicles within the cell. Cellular activities, such as exocytosis and endocytosis, as well as the extension of filopodia and cell migration (fig. 6-D). 2. Gel-like networks: provide the structural foundation of much of the cell cortex (fig. 6-C). 3. Bundles: form the core of microvilli (apical cell projections) (fig. 6-A). 4. Focal points: points of contact between the cell and the extracellular matrix (fig 6-B). 4 Fig. 6- Functional forms of actin A B Focal points F C D 5 14 Cell Cycle control & Mitosis ILOs By the end of this lecture, students will be able to 1. Interpret the significance of different phases of the cell cycle. 2. Correlate the phases of mitosis to normal and abnormal division outcomes. Cell cycle control The capability of the cell to begin and advance through the cell cycle is governed by the presence and interactions of a group of related proteins known as cyclins, with specific cyclin-dependent kinases (CDKs). Thus: Cyclin D, synthesized during early G1 phase, binds to CDK4 as well as to CDK6. Additionally, in the late G1 phase cyclin E is synthesized and binds to CDK2. These three complexes, through other intermediaries, permit the cell to enter and progress through the S phase. Cyclin A binds to CDK2 and CDK1 and these complexes permit the cell to leave the S phase and enter the G2 phase and induce the formation of cyclin B. Cyclin B binds to CDK1, and this complex allows the cell to leave the G2 phase and enter the M phase.(Figure 23-2) Fig 23-2. Control of the cell cycle 1 Fate of cyclins Once the cyclins have performed their specific functions, they enter the ubiquitin- proteasome pathway, where they are degraded into their component molecules. Cell cycle check points The cell also employs quality control mechanisms, known as checkpoints, to safeguard against early transition between the phases. These checkpoints ensure the meticulous completion of essential events, such as adequate cell growth, correct DNA synthesis, and proper chromosome segregation, before permitting the cell to leave its current phase of the cell cycle. The cell accomplishes such delays in the progression through the cell cycle by activating inhibitory pathways and/or by suppressing activating pathways. (check the details in Figure 23-3) Factors stimulating the cell to enter the cycle: The triggers inducing the cell to enter the cell cycle may be: (1) A mechanical force (e.g., stretching of smooth muscle), (2) Injury to the tissue (e.g., ischemia), and (3) Cell death. All of these incidents cause the release of ligands by signaling cells in the involved tissue. Frequently, these ligands are growth factors that indirectly induce the expression of proto-oncogenes, genes that are responsible for controlling the proliferative pathways of the cell. Clinical Hint Oncogenes and cancer: Normal cell proliferation and differentiation are controlled by a group of genes called protooncogenes; altering the structure or expression of these genes promotes the production of tumours. The expression of proto-oncogenes must be very strictly regulated to prevent unwanted and uncontrolled cell proliferation. Mutations in proto-oncogenes that enable the cell to escape control and divide in an uncontrolled way are responsible for many cancers. Such mutated proto-oncogenes are known as oncogenes. Altered oncogene activity can be induced by a change in the DNA sequence (mutation), an increase in the number of genes (gene amplification), or gene rearrangement. The Chromosomes Chromosomes are chromatin fibers that become so condensed and tightly coiled during mitosis and meiosis so that they are visible with the light microscope. 2 Each chromosome is formed of two sister chromatids attached at a point called the centromere. As the cell leaves the interphase stage and prepares to undergo mitotic or meiotic activity, the chromatin fibers are extensively condensed to form chromosomes, carrying the duplicated DNA (In which phase of the cell cycle?) Fig. 23-3. Structure of the chromosome 3 Types of cells in the human body 1. Somatic cells They are the body cells that are produced by mitosis and contain 46 chromosomes (diploid number), representing 23 homologous pairs of chromosomes. One member of each of the chromosome pairs is derived from the maternal parent; the other comes from the paternal parent. Of the 23 pairs, 22 are called autosomes; the remaining pair, which determines gender, are the sex chromosomes. The sex chromosomes of the female are two X chromosomes (XX); those of the male are the X and Y chromosomes (XY). Only one of the two X chromosomes in female somatic cells is transcriptionally active. The inactive X chromosome, randomly determined early in development, remains inactive throughout the life of that individual. The number of chromosomes in somatic cells is specific for the species and is called the genome, the total genetic makeup. 2. Germ cells They are either the sperm in male or ovum in the female and produced by meiosis. They contain 23 chromosomes (haploid number) and one sex chromosome either X or Y. Mitosis (M) occurs at the conclusion of the G2 phase and thus completes the cell cycle. Mitosis is the process whereby the cytoplasm and the nucleus of the cell are divided equally into two identical daughter cells. First, the nuclear material is divided in a process called karyokinesis, followed by division of the cytoplasm, called cytokinesis. The process of mitosis is divided into four distinct stages: prophase, metaphase, anaphase, and telophase Mitosis Mitosis is the process of cell division that results in the formation of two identical daughter cells. I- Prophase During prophase, the chromosomes condense, and the nucleolus disappears. Each chromosome consists of two parallel sister chromatids. As chromosomes condense, the nucleolus disappears. The centrosome also divides into two regions, each half containing a pair of centrioles and a microtubule-organizing center (MTOC), which migrate away from each other to opposite poles of the cell. 4 Fig. 23-4 Centrosome structure & prophase From each MTOC, astral rays and spindle fibers develop, giving rise to the mitotic spindle apparatus. It is thought that the astral rays (microtubules that radiate out from the pole of the spindle) may assist in orienting the MTOC at the pole of the cell. Those microtubules that attach to the centromere region of the chromosome are the spindle fibers, which assist in directing the chromosome alignment on cell equator and later migration of chromatids to the cell pole. In the absence of centrioles, the microtubule-nucleating material is dispersed within the cytoplasm with the result that astral rays and spindle fibers do not form properly, and mitosis does not proceed in the appropriate manner. (How is related to the cell cycle?) Spindle fibers bind to the kinetochore in preparation for chromatid migration to effect karyokinesis. II- Metaphase The nuclear envelope disappears early at this phase. The newly duplicated chromosomes align themselves on the equator of the mitotic spindle, being directed by the spindle fibers, and become maximally condensed. Each chromatid is oriented parallel to the equator, and spindle microtubules are attached to its kinetochore (a protein on the centromere that helps in movement of chromatids), radiating to the spindle pole (fig. 22-5). 5 Fig. 23-5. Metaphase & kinetochore III- Anaphase During anaphase, the sister chromatids separate and begin to migrate to opposite poles of the cell, and a cleavage furrow begins to develop (fig. 22-6). The spindle/kinetochore attachment site leads the way, with the arms of the chromatids simply moving along, contributing nothing to the migration or its pathway. In late anaphase, a cleavage furrow begins to form at the plasmalemma, indicating the region where the cell will be divided during cytokinesis. Figure 23-6 Anaphase & telophase IV- Telophase Telophase, the terminal phase of mitosis, is characterized by cytokinesis, reconstitution of the nucleus and nuclear envelope, disappearance of the mitotic spindle, and unwinding of the chromosomes into chromatin. 6. The chromosomes uncoil and become organized into heterochromatin and euchromatin of the interphase cell. Nucleolus reappears. Cytokinesis is the division of the cytoplasm into two equal parts during mitosis. The cleavage furrow continues to deepen (fig. 22-6). The remaining microtubules are surrounded by a contractile ring, composed of actin and myosin filaments attached to the plasma membrane. Constriction of the ring helps in separation of the two new cells. Each daughter cell resulting from mitosis is identical in every respect, including the entire genome, and each daughter cell possesses a diploid (2n) number of chromosomes. Clinical correlations Understanding of mitosis and the cell cycle has greatly aided cancer chemotherapy, making it possible to use drugs at the time when the cells are in a particular stage of the cell cycle. For example, vincristine and similar drugs disrupt the mitotic spindle, arresting the cell in mitosis. Colchicine , another plant alkaloid that produces the same effect, has been used extensively in studies of individual chromosomes and karyotyping. 7 17 GENE EXPRESSION 2. RNA TRANSLATION AND GENETIC CODE ILOs By the end of this lecture, students will be able to 1. Discuss the rules of genetic code 2. Correlate the function of different RNAs to the process of translation 3. Describe the process of translation 4. Interpret role of translation and post translational modification in health and disease What is translation? It is the translation of the nucleotide sequence of a mRNA (Codons) into an amino acid sequence of a protein, in order to synthesize proteins. Each codon consists of a sequence of 3 nucleotides i.e. it is a triplet code. Collection of these codons makes up the genetic code. Protein biosynthesis is called translation because it involves translation of information from the 4- letter language and structure of nucleic acid into the 20-letter language and structure of proteins Requirements of translational process m RNA as a carrier of genetic information. tRNA as an adapter molecule, which recognizes an amino acid on one end and its corresponding codon on the other end. At least one specific type of tRNA is required for each amino acid. Ribosomes as the molecular machine coordinating the interaction between mRNA, tRNA, the enzymes and the protein factors required for protein synthesis. Genetic code (Figure 1) It is the relationship between nucleotide sequence in DNA or mRNA AND amino acids in a polypeptide chain. Each amino acid can be specified by more than one codon. There is one start codon: AUG (METHIONINE) There are 3 STOP codons (UAA, UAG, UGA) The genetic information along mRNA is read from 5’ to 3’ direction. Figure 1.genetic code 1 Characteristics of genetic code 1- Genetic code is degenerate i.e. multiple codons can code for the same amino acid except tryptophan and methionine.(both are coded by only one codon) Wobble theory: The 3rd nucleotide in a codon is less important than the other two in determining the specific amino acid to be incorporated. (i.e if an amino acid has several codons, all such cosons will usually have the first 2 letters in common but the third is different, which means the 3 rd is of less importance) 2- Genetic code is unambiguous i.e. each codon specifies no more than one amino acid. 3- Genetic code is non-overlapping and Commaless meaning that the code is read from a fixed starting point as a continuous sequence of bases, taken three at a time without any punctuation between codons. For example, AGCUGGAUACAU is read as AGC UGG AUA CAU. 4-Genetic code is universal i.e. the same code words are used in all organisms (pro- and eukaryotes) Protein Biosynthesis stages 1- Initiation 2- Elongation 3- Termination Stage 1: Initiation For initiation of protein biosynthesis, there must be:- - tRNA - rRNA - mRNA - Eukaryotic initiation factors (eIFs). - GTP, ATP and different amino acids. In this stage, The 80 S eukaryotic ribosome is dissociated into 40 S and 60 S subunits. eIF – 3 and eIF-1 bind to 40 S subunit thus preventing re-association between the 2 subunits. GTP and eIF-2 bind, in addition to binding of mRNA (accompanied by hydrolysis of ATP to ADP+Pi)and Methionine– tRNA (a tRNA specifically involved in binding to the initiation codon AUG). This This is followed by re-association of both ribosomal units, with dissociation of initiation factors and hydrolysis of GTP. This is termed the initiation complex. (Can you enumerate its components?) (Figure2) Figure 2. Initiation of translation 2 N.B: t RNA charging (Figure 3) It means recognition and attachment of the specific amino acid to the 3` hydroxyl adenosine terminus (to the sugar) of tRNA in an ester linkage. Figure 3.tRNA charging Stage 2: Elongation It is a cyclic process involving 3 steps I. Binding of aminoacyl – tRNA to the A site The ribosome has three binding sites for tRNA molecules: the A, P, and E sites.(Figure 4) In the complete 80S ribosome subunit, A site is free (N.B. A=aminoacyl binding site) Binding of aminoacyl t-RNA to A site needs activation of aminoacyl tRNA by binding of eukaryote elongation factor - 1 (e EF-1) and GTP. When aminoacyl tRNA binds to A site, GTP is hydrolysed and e EF-1 is released. Figure 4..Binding sites of tRNA in ribosome N.B: Anticodon: Each tRNA molecule contains a three-base nucleotide sequence, the anticodon, which pairs with a specific codon on the mRNA within the ribosome. This codon specifies which aminoacid will be inserted in the growing peptide sequence. The first codon on mRNA always codes for methionine. II. Peptide bond formation (Figure 5) The alpha amino group of the new amino acid carried by t-RNA in the A site attacks the carboxylic group of the peptidyl-tRNA in the P site. This reaction needs peptidyl transferase enzyme (RIBOZYME) The reaction results in attachment of the growing peptide chain to the tRNA in the A site. III. Translocation (Figure 5) Upon removal of the peptide from t-RNA in the P site, the discharged t-RNA quickly dissociates. eEF-2 and GTP are responsible for translocation of the newly formed peptidy t-RNA from A site to P site 3 The A site is now free to receive a new aminoacyl-RNA Stage 3: Termination (Figure 6) After many cycles of elongation, the non-sense or stop codon of mRNA (UAA, UAG or UGA) appears in the A site. Normally, there is no tRNA with an anticodon capable of recognizing such a termination signal. Releasing factors (eRFS) can recognize the termination signals in the A site Releasing factors(eRFs), GTP and peptidyl transferase promote the hydrolysis of the bond between the peptide chain and t-RNA at P site 80S subunit dissociates and all the factors , tRNA , mRNA, GDP and Pi are released Figure 5. Process of elongation Figure 6.Termination of translation N.B. The formation of one peptide bond requires energy resulting from hydrolysis of 4 high energy phosphate bonds:- Charging of tRNA with amino acyl moiety requires hydrolysis of an ATP to an AMP. (2 high energy bonds) The entry of amino tRNA into the A site requires one GTP hydrolysis to GDP. The translocation of the newly formed peptidyl – tRNA in the A site into the P site results in hydrolysis of one GTP to GDP N.B. Errors in translation will result in faulty proteins, which will be either targeted for degradation in proteasome or will be non- functioning or abnormally functioning. Protein maturation Aim: Activation of protein to a functional form 4 Localization in subcellular compartment Secretion from the cell Protein maturation involves the following: I- Protein folding: Folding to 3D structures, aided by molecular chaperone. (Refer to protein structure lecture). Misfolded proteins are targeted for destruction II- Post – translational processing: a- Proteolysis: It means removal of amino terminal, carboxy terminal or internal sequences. Examples: 1-Removal of amino terminal methionine residues 2-Removal of signal peptides by signal peptidases (signal peptides help translocate the proteins to its final destination and hence are removed after protein transport) (figure 7) b- Modifications of individual amino acids Figure 7.Proteolysis Hydroxylation: of proline and lysine for collagen synthesis Phosphorylation of serine, threonine or tyrosine (Important in cell signaling) γ-carboxylation of glutamic acid in prothrombin and osteocalcin (this helps Ca 2+ binding which is necessary for blood clotting and bone ossification in both proteins respectively) c- Addition of certain groups Glycosylation: Attachment of CHO side chain to form glycoproteins. Glycosylation can help stabilize glycoproteins against degradation or provide proper conformation for protein function. CHO can be either N linked( to asparagine), or O-linked( to serine and threonine) Acylation: Addition of fatty acids to various amino acid side chains. Acylation has several functional effects on proteins, especially to help anchor them to membranes. Clinical implications: I) Many antibiotic work by altering the translation of bacterial DNA and are generally classified as bacterial protein synthesis inhibitors as Tetracyclines and Macrolides like Erythromycin. II) Some toxins can cause death by inhibiting eukaryotic translation: Shiga toxin / ricin (produced by E-coli bacteria and causes bloody diarrhea) inhibits tRNA binding by acting on 60S subunit Diphtheria toxin (produced by bacteria and causes difficult breathing, heart failure, paralysis, and even death) inhibits translocation through binding to Eukaryotic- EF-2 5 21 Autophagy, Lysosomes, Peroxisomes & cell inclusions ILOs By the end of this lecture, students will be able to 1. Explain the role of autophagy as a cellular sink 2. Describe the origin of lysosomal enzymes and their function in health and disease. 3. Predict the role especially of autolysosome and phagolysosome in physiological conditions 4. Connect the structure of proteasome to its degrative function. 5. Predict the role of peroxisomes in cell adaptation to patterns of stress. 6. Correlate the types of cytoplasmic inclusions to patterns of cell activity 7. Justify the impact of its derangement on cellular health. 1. Lysosomes Lysosomes are membrane bounded cell organelles that have an acidic pH and contain hydrolytic enzymes. It contains at least 40 different types of acid hydrolases, such as sulfatases, proteases, nucleases, lipases that are active in acidic pH. These enzymes are manufactured in the same steps of protein synthesis following the same steps in rER, packed in Golgi complex and released in vesicles from trans Golgi network. Lysosomes receive contents to be digested from late endosomes. Lysosomes aid in digesting phagocytosed microorganisms, cellular debris, and cells but also excess or senescent organelles, such as mitochondria and RER. The various enzymes digest the engulfed material into small, soluble end products that are transported by carrier proteins in the lysosomal membrane from the lysosomes into the cytosol andare either reused by the cell or exported from the cell into the extracellular space. Transport of Substances into Lysosomes Substances destined for degradation within lysosomes reach these organelles in one of three ways: through phagosomes, pinocytotic vesicles, or autophagosomes. 1- Phagosomes: Phagocytosed material, contained within phagosomes, moves toward the interior of the cell. The phagosome joins either a lysosome or a late endosome. The hydrolytic enzymes digest most of the contents of the phagosome, especially the protein and carbohydrate components. Lipids, however, are more resistant to complete digestion, and they remain enclosed within the spent lysosome, now referred to as a residual body. (Fig. 1) 1 Fg 1. Pathways of intracellular digestion by lysosomes 2- Autophagy: The term autophagy is derived from the Greek word meaning 'self- devouring'. Senescent organelles such as mitochondria or the RER, need to be degraded. The organelles in question become surrounded by elements of the endoplasmic reticulum and are enclosed in vesicles called autophagosomes. Fate of autophagosomes: These structures fuse either with late endosomes or with lysosomes and share the same subsequent fate as the phagosome. (Fig. 1) Autophagy is a self-digesting mechanism responsible for removal of long-lived proteins, damaged organelles, and malformed proteins during biosynthesis by lysosome. Significance of autophagy Regulation of diverse cellular functions including growth, differentiation, response to nutrient deficit and oxidative stress, cell death, and macromolecule and organelle turnover. Mechanism Autophagosome formation is regulated by dozens of “autophagy-related genes” called Atgs. Mutation leads to formation of a double-membrane vesicle, which encapsulates cytoplasm, malformed proteins, long-lived proteins, and organelles and then fuses with lysosomes for degradation. 2 Autophagy Regulation Autophagy is activated in response to diverse stress and physiological conditions. For example, food deprivation, hyperthermia, and hypoxia, which are known as major environmental modulators of ageing, are also conditions that induce autophagy. Figure 2 - Stages of autophagy Autophagy and Diseases Autophagy is important in normal development and responds to changing environmental stimuli. On starvation, autophagy is greatly increased, allowing the cell to degrade proteins and organelles and thus obtain a source of macromolecular precursors, such as amino acids, fatty acids, and nucleotides, which would not be available otherwise. Autophagy roles in cancer are a topic of intense debate. In one hand, autophagy has an anticancer role. On the other hand, when tumor cells are starved due to limited angiogenesis, autophagy stops them from dying. Autophagy is important in numerous diseases, including bacterial and viral infections, neurodegenerative disorders, several myopathies, and cardiovascular diseases. 3 Autophagy and weight loss A type of intermittent fasting is used to stimulate autophagy and to 'trick' one's metabolism into working longer hours and burning more fat. Notably, pharmacological stimulation of autophagy can reduce both weight gain and obesity-associated alterations upon hypercaloric regimens usage. Proteasomes Proteasomes are small organelles composed of protein complexes (proteases) that are responsible for proteolysis (protein breakdown) of malformed and ubiquitin-tagged proteins. Proteasomes monitor the protein content of the cell to ensure degradation of unwanted proteins, such as excess enzymes and other proteins that become unnecessary to the cell after they perform their normal functions, and malformed proteins. Protein encoded by virus should also be destroyed. The process of cytosolic proteolysis is carefully controlled by the cell, and it requires that the protein be recognized as a potential candidate for degradation. This recognition involves ubiquination, a process whereby several ubiquitin molecules (a 76-amino acid long polypeptide chain) are attached to the candidate protein using ATP. Once a protein has been marked, it is degraded by proteasomes. (Fig 2) During proteolysis, the ubiquitin molecules are released and re-enter the cytosolic pool to be re used. Fig. 3. The structure and function of the proteasome 4 Protein degradation by proteasomes in health and disease Proteins destined for degradation are labeled with ubiquitin through covalent attachment to a lysine side chain. The amino acid composition at the amino terminus determines how quickly the protein will be ubiquinated and thus the half-life of the protein. Some proteins have very long half-lives, such as the crystallins in the lens of the eye; these proteins do not turn over significantly during the human life span. Because they were synthesized largely in utero, about half the crystallins in the adult lens are older than the person. Other proteins have half-lives of 4 months (proteins such as hemoglobin that last as long as the red blood cell), or the half-life can be very short, such as for ornithine decarboxylase, which has a half-life of 11 minutes. The half-lives of proteins is influenced by the amino (N)-terminal residue, the so- called N-end rule. Destabilizing N-terminal amino acids (causing short half-life) include arginine and acetylated alanine. In contrast, serine is a stabilizing amino acid. Additionally, proteins rich in sequences containing proline, glutamate, serine, and threonine (called PEST sequences) are rapidly ubiquinated and degraded and, therefore, have short half-lives Poly-ubiquination, which increases the rate of turnover/degradation of a protein, occurs by successive addition of free ubiquitin to that which is already bound to the protein. Failure of degradation of misfolded proteins by proteasomes, can lead to accumulation of abnormal proteins and development of certain diseases such as Alzheimer’s disease and Creutzfeldt–Jakob disease (Mad-cow disease). Peroxisomes Peroxisomes are small membrane bounded, self-replicating organelles. They contain more than 40 oxidative enzymes, especially urate oxidase, and D- amino acid oxidase that contain oxidative enzymes. Peroxisomes function in the catabolism of long-chained fatty acids (beta oxidation), forming acetyl coenzyme A (CoA) as well as hydrogen peroxide (H2O2) by combining hydrogen from the fatty acid with molecular oxygen. Similar to mitochondria, peroxisomes increase in size and undergo fission to form new peroxisomes; however, they possess no genetic material of their own. Inclusions Inclusions are non-living components of the cell that do not possess metabolic activity and are not bounded by membranes. The most common inclusions are glycogen, lipid droplets, pigments, and crystals. 5 1. Glycogen Glycogen is the most common storage form of glucose in human and is especially abundant in cells of muscle and liver. It appears in electron micrographs as clusters, or rosettes, of β particles (and larger α particles in the liver) that resemble ribosomes, located in the vicinity of the SER. On demand, enzymes responsible for glycogenolysis degrade glycogen into individual molecules of glucose. 2. Lipids Lipids, triglycerides in storage form, not only are stored in specialized cells (adipocytes) but also are located as individual droplets in various cell types, especially hepatocytes. Lipids are considered as potential source of energy within the cells. 3. Pigments It could be natural pigments as haemoglobin of red blood cells, melanin in the skin and hair and a yellow-to-brown pigment, lipofuscin in the long-lived cells, such as neurons and cardiac muscle. Tattoos is the injection of ink intracellular that could be phagocytosed by macrophages leading to its permanent effect. Fig. 4 Types of inclusions A. TEM of glycogen ganules in rosettes B. Lipid droplets in fat cell Clinical hint: abnormal accumulations I. Lipids 1-Steatosis (Fatty Change) Means excessive, abnormal accumulations of triglycerides within parenchymal cells due to alcohol abuse, diabetes mellitus, obesity, toxins, protein malnutrition, and anoxia 2- Cholesterol and Cholesterol Esters as in atherosclerosis. 6 I. Proteins as inAlzheimer disease. II. Glycogenas in Diabetes mellitus and Glycogen storage diseases. III. Pigments; Exogenous Carbon (coal dust),The most common air pollutant in urban areas. Its accumulation could lead to Anthracosis occurs in heavy smokers, and coal mines workers with accumulation of carbon pigment within lungs and regional lymph nodes. Endogenous Pigments Lipofuscin Patients with severe malnutrition& Cancer cachexia. Melanin: Hyperpigmentation generalized due to excessive sun exposure or localized as in benign (nevus) and malignant cutaneous tumors. Hypopigmentation Generalized as in albinism or localized as in vitiligo (autoimmune disorder). 7 26 Cytoskeleton & intercellular junctions ILOs By the end of this lecture, students will be able to 1. Correlate stable nature of IM to its supportive role in the cell. 2. Discuss the significance of cellular specificity of the IM in diagnosis of tumors origin. 3. Deduce structural adaptation of the cell junction to its function. 4. Interpret the impact of molecular structure abnormality on tissue integrity. Intermediated Filaments ⮚ These rope-like intermediate filaments are constructed of tetramers of rod-like proteins that are tightly bundled into long helical arrays (Fig 1). Fig 1. Molecular structure and organization of Intermediated filaments ⮚ The individual subunit of each tetramer differs considerably for each type of intermediate filament. The categories of intermediate filaments include keratins, desmin, vimentin, glial fibrillary acidic protein, neurofilaments, and nuclear lamins. Page 1 of 4 Examples of Intermediate Filaments Found in Eukaryotic Cells. Filament Type Cell Type Examples Keratins Epithelium Both keratinizing and nonkeratinizing epithelial cells Desmin Muscle Striated and smooth muscle (except vascular smooth muscle) Gilial fibrillary acidic Glial cells Astrocytes proteins Neurofilaments Neurons Nerve cell body and processes The intercellular junctions These are specialized attachment areas (cell junctions) present on cells that are in close contact with each other. Morphologically, they are classified into three types: a) Zonula (belt-like) junctions completely encircle the cells. b) Fascia (sheet- like) junctions form broad areas of contact between cells. c) Macula (disc-like) junctions are like spot welds on the cell surface. According to their function, the common cell junctions are: 1. Occluding junctions: function in joining cells to form an impermeable barrier, preventing material from taking an intercellular route in passing across the cellular sheath. Page 2 of 4 2. Anchoring junctions (zonula and macula adherens) function in maintaining cell-to-cell or cell-to-basal lamina adherence, thus provide mechanical stability in tissues subjected to mechanical pressure. 3. Communicating junctions: function in permitting movement of ions or signaling molecules between cells, thus coupling adjacent cells both electrically and metabolically. According to their molecular structure, they include: I- Zonula occludens (tight junctions): They form a "belt-like" junction that encircles the entire circumference of the cell. It is established by fusion of the outer layers of the cell membranes of the two cells in an interrupted pattern, where fusion is represented by focal points. (Fig. 1) At the fusion sites, transmembrane junctional proteins called claudins and occludins bind to each other, thus forming a seal occluding the intercellular space. A third transmembrane protein called cadherin reinforces the other two types. (Fig 2) II- Zonula adherentes The intercellular space of 15 to 20 nm between the outer leaflets of the two adjacent cell membranes is occupied by the extracellular moieties of cadherins. (Fig 2) These Ca2+-dependent integral proteins of the cell membrane are transmembrane linker proteins. Their intracytoplasmic aspect binds to a specialized region of the cell web, specifically a bundle of actin filaments. III- Desmosomes (macula adherentes) These "spot weld"-like junctions that are randomly distributed along the lateral cell membranes of adjacent cells. (Fig 1) Disk-shaped attachment plaques are located opposite each other on the cytoplasmic aspects of the plasma membranes of adjacent epithelial cells. (Fig 2) Each plaque is composed of a series of attachment proteins. Intermediate filaments of cytokeratin are observed to insert into the plaque, where they make a hairpin turn, then extend back out into the cytoplasm. IV- Gap junctions (Nexus) Gap junctions are built by six closely packed transmembrane channel-forming proteins (connexins) that assemble to form channel-structures called connexons, aqueous pores through the plasma membrane that juts out about 1.5 nm into the intercellular space. (Fig 2A) Page 3 of 4 When a connexon of one plasma membrane is in register with its counterpart of the adjacent plasma membrane, the two connexons fuse, forming a functional intercellular hydrophilic communication channel. Page 4 of 4 32 Meiosis ILOs By the end of this lecture, students will be able to 1. Correlate chromosomal changes to different phases of meiosis. 2. Interpret role of crossing over in genetic variations. 3. Interpret the different types of chromosomal abnormalities. 4. Differentiate between aneuploidy and polyploidy. 5. Differentiate between balanced and unbalanced karyotypes. 6. Correlate the phenotypic outcome with types of chromosomal aberrations. Meiosis Meiosis is a special type of cell division resulting in the formation of gametes (spermatozoa or ova) whose chromosome number has been reduced from the diploid (2n) to the haploid (1n) number. Meiosis begins at the conclusion of interphase in the cell cycle. It produces the germ cells- the ova and the spermatozoa. This process has two crucial results: 1. Reduction in the number of chromosomes from the diploid (2n) to the haploid (1n) number, ensuring that each gamete carries the haploid amount of DNA and the haploid number of chromosomes. 2. Recombination of genes, ensuring genetic variability and diversity of the gene pool Meiosis is divided into two phases: I- Meiosis I, or reductional division (first event): Homologous pairs of chromosomes line up, members of each pair separate and go to opposite poles, and the cell divides; thus, each daughter cell receives half the number of chromosomes (haploid number). II- Meiosis II, or equatorial division (second event): The two chromatids of each chromosome are separated, as in mitosis, followed by migration of the chromatids to opposite poles and the formation of two daughter cells. These two events produce four cells (gametes), each with the haploid number of chromosomes and haploid DNA content. Page 1 of 3 In gametogenesis, when the germ cells are in the S phase of the cell cycle preceding meiosis, the amount of DNA is doubled to 4n but the chromosome number remains at 2n (46 chromosomes). Meiosis I 1. Prophase I: It begins after the DNA has been doubled to 4n in the S phase. Prophase of meiosis I lasts a long time. Homologous pairs of chromosomes approximate each other and condense. The most significant event in prophase I is formation of chiasmata (crossing over sites) as random exchange of genetic material occurs between homologous chromosomes. 2. Metaphase I: is characterized by lining up of homologous pairs of chromosomes, each composed of two chromatids, on the equatorial plate of the meiotic spindle. 3. Anaphase I: Homologous chromosomes migrate away from each other, going to opposite poles. 4. Telophase I: The chromosomes reach the opposing poles, nuclei are re-formed and cytokinesis occurs, giving rise to two daughter cells. Each cell possesses 23 chromosomes, the haploid (1n) number, but because each chromosome is composed of two chromatids, the DNA content is still diploid. Figure 2. Phases of meiosis d; double amount of DNA, s; haploid amount of DNA Page 2 of 3 Meiosis II (equatorial division) occurs without DNA synthesis and proceeds rapidly through four phases and cytokinesis to form four daughter cells each with the haploid chromosome number It is subdivided into prophase II, metaphase II, anaphase II, telophase II, and cytokinesis The chromosomes line up on the equator, the kinetochores attach to spindle fibers, followed by the chromatids migrating to opposite poles, and cytokinesis divides each of the two cells. Outcome of meiosis II: 1. Results in a total of four daughter cells from the original diploid germ cell. Each of the four cells contains a haploid amount of DNA and a haploid chromosome number. 2. The cells are genetically distinct because of reshuffling of the chromosomes and crossing over. Thus, every gamete contains its own unique genetic complement. Page 3 of 3