Highway Midterm PDF
Document Details
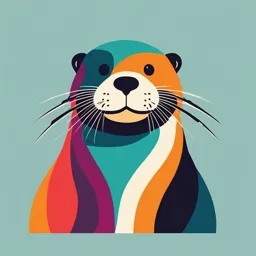
Uploaded by SumptuousBandura
Nikki Laurence Marquez, Francisc Ken Macagaling, John Carlo Francisco
Tags
Related
- Module 3 Design Criteria for Highways and Railways PDF
- Roles and Responsibilities of Transportation Engineer PDF
- Highway and Railway Geometric Design PDF
- CE331 - Design Criteria for Highways and Railways PDF
- Highway and Railway Design Criteria PDF
- Module 4: Geometric Criteria for Highways and Railways PDF
Summary
This document provides an overview of highway and railway design, focusing on various aspects, including cross-sections, horizontal and vertical alignments, super-elevation, earthworks, and components of a standard railway cross-section. It's suitable for undergraduate engineering studies.
Full Transcript
GEOMETRIC DESIGN OF HIGHWAYS AND RAILWAYS including cross sections, horizontal and vertical alignments, super- elevation and earthworks REPORTERS: NIKKI LAURENCE MARQUEZ FRANCISC KEN MACAGALING JOHN CARLO FRANCISCO Geometric Design of Railway Track should be such as to...
GEOMETRIC DESIGN OF HIGHWAYS AND RAILWAYS including cross sections, horizontal and vertical alignments, super- elevation and earthworks REPORTERS: NIKKI LAURENCE MARQUEZ FRANCISC KEN MACAGALING JOHN CARLO FRANCISCO Geometric Design of Railway Track should be such as to provide the maximum efficiency in the traffic operation with maximum safety at reasonable cost. Gradients any departure of track from the level is known as grade or gradient. The purpose of providing gradient is provide uniform rate of rise or fall, to reduce cost of earthwork, to reach different stations at different level. Types of Gradients: a. Ruling Gradient b. Momentum Gradient c. Pusher or Helper Gradient d. Gradient at station yards a. Ruling Gradient – the steepest gradient allowed on the track section b. Momentum Gradient – the gradient on a section which are steeper than the ruling gradient acquire sufficient momentum to negotiate. c. Pusher or Helper Gradient – the gradient where extra engine is required to push the train. d. Gradients at station yards – it is provided to prevent resistance due to grade on the starting vehicles. Components of a Standard Railway Cross Section Provides a side-on view of the various components that make up the track and surrounding structures. Here’s a breakdown of the key elements typically found in a railway cross section: Formation Layer Ballast Sleepers Shoulders Side Drainage FORMATION LAYER The base layer that supports the entire railway track. It consists of Subgrade and Blanket Layer. Subgrade is the natural ground on which the railway track is laid. It needs to be stable and compacted. Blanket Layer is often placed between the subgrade and the ballast to protect the subgrade from water and distribute loads. BALLAST The crushed stones or gravel that form the trackbed. They help to distribute the load from the sleepers, allow for water drainage, and hold the track in place. The appropriate thickness of a layer of track ballast depends on the size and spacing of the ties, the amount of traffic on the line, and various other factors. Track ballast should never be laid down less than 150 mm (6 inches) thick, and high-speed railway lines may require ballast up to 0.5 meters (20 inches) thick. Ballast less than 300 mm (12 inches) thick can lead to vibrations that damage nearby structures. However, increasing the depth beyond 300 mm (12 inches) confers no extra benefit in reducing vibration. SLEEPERS The rectangular supports that sit between the rails to hold them at the proper gauge (distance apart). Sleepers can be made of wood, concrete, or steel. The main function of sleepers in railway are: To keep the rails firmly in place also maintain a uniform gauge. These facilitate the transfer of wheel loads to the ballast. Rail sleepers lessen the vibrations emanating from the rails. These provide lateral and longitudinal stability. SHOULDERS The space between the rail and the edge of the ballast, ensuring lateral stability for the track structure. There are two types of rail shoulders available, these are: Cast-in Shoulder is the embedded part of the railway fastening system are pressed in concrete sleeper and work together with the ”E clip” to fasten the rail. The cast in shoulder is made by casting from iron. Weld-on Shoulder is used at the typical position of rail where the rail shoulder is weld on flat steel plate rather than the standard railroad tie plate. The materials for weld-on shoulders production can be low or mid carbon steel, based on the customer’s requirements. SIDE DRAINAGE Effective drainage on both sides of the railway track is essential for maintaining stability and preventing water accumulation. Side drains should be provided along the track cuttings and zero fill locations, where the cess level is not above the ground level. All side drains should be provided with concrete lining. CROSS-SECTION ELEMENTS Types of Railway Cross Sections Single Track Section Double Track Section Electrified Section Tunnel Cross Section Elevated Cross Section SINGLE TRACK SECTION A simple cross-section with a single track, common in less congested railway lines. Single tracks are common in rural or less congested areas where train traffic is infrequent, as it reduces construction and maintenance costs. Since trains travel in both directions on a single track, passing loops (also known as sidings) are often built at intervals to allow trains traveling in opposite directions to pass each other. DOUBLE TRACK SECTION Two tracks side by side, allowing for two-way travel, which is common in busy mainlines. Double track railways can handle more traffic than single tracks, making them ideal for busy routes, mainlines, or corridors with high train frequency. Since trains don’t have to wait for others to pass, double track systems allow for smoother, faster, and more reliable scheduling, reducing delays. ELECTRIFIED SECTION Includes overhead catenary wires and poles for powering electric trains. The cross section will also include details on clearance and placement of power systemics. Most high-speed trains operate on electrified tracks due to the higher efficiency, power, and performance of electric trains, which are essential for achieving speeds over 300 km/h (186 mph). TUNNEL CROSS SECTION A tunnel cross section is designed to fit within a subterranean or covered space, providing a clear profile of the tunnel's dimensions, shape, and structure. It accommodates the train and its necessary components like tracks, electrification, ventilation, and drainage. In tunnel cross sections, the track design may include adjustments for ventilation, tight curves, and the need for noise and vibration control, especially in urban areas where tunnels are close to residential or commercial buildings. ELEVATED CROSS SECTION It involves the railway tracks being supported on raised structures, such as piers, columns, or bridges, lifting the tracks above ground level to avoid interference with roads, rivers, or other infrastructure. The height of the elevated structure must provide adequate clearance for vehicles, pedestrians, or waterways underneath. This typically ranges from 5 to 10 meters depending on the purpose of the elevation. Geometric Design for Highways the geometric design of roads is the branch of highway engineering concerned with the positioning of the physical elements of the roadway according to standards and constraints. Relationship of Traffic to Highway Design The major traffic elements that influence highway design are: Average daily traffic (ADT) and annual average daily traffic (AADT) Design hour volume (DHV) Directional distribution (D) Percentage of trucks (T) Design speed (V) Directional distribution (D) - is the one-way volume in the predominant direction of travel, expressed as a proportion of the volume in the two-way design hour volume. - For rural two-lane roads, it ranges from 0.50 to 0.70 (50%-70% of traffic in one direction) and is typically assumed to be 0.60 (60%). Composition of traffic (T) - Usually expressed as the percentage of trucks (exclusive of light delivery trucks) present in the traffic flow during the design hour. - The percentage typically varies from about 5 to 19 percent In urban areas, the percentage of trucks traveling within the overall flow of traffic during peak hour tends to be considerably less than percentages on a daily basis. MAJOR OBJECTIVE IN HIGHWAY DESIGN Create a highway with dimensions and alignment that can serve the design service flow rate, which should be at least as great as the flow rate during the peak 15-minute period of the design hour, but not so great as to represent as extravagance in the design. Roadway Capacity The traffic volumes that can be served at each level of service are referred to as “service flow rate”. Design Speed The assumed design speed for a highway may be considered as “a selected speed used to determine the various geometric design features of the roadway”. Design speeds typically range from 15 to 75 mph (20 to 120 km/hr) The vehicle width The vehicle height naturally affects the affects the width of the traffic clearance of the lane. various structures. The vehicle length has a bearing on roadway capacity and affects the turning radius. The vehicle Design Vehicle weight affects the structural - The design of the motor design of the vehicles that will utilize the roadway. proposed facility also influence the design of a roadway project. American Association of Highway and Transportation Officials (AASHTO) recommends four design vehicle classes for geometric design of highway facility. 1. Passenger cars 2. Buses 3. Trucks 4. Recreational vehicles CROSS-SECTION ELEMENTS Highway Travel Lanes Roads presently in use have traditionally been separated into generalized categories that include: Two-lane highways Three-lane highways Multilane undivided highways Multilane divided highways Limited-access highways TWO-LANE HIGHWAYS It refers to roadways consisting of two lanes in the cross section, one for each direction of travel. Two-lane roads vary from low-type roads, which follow the natural ground surface, to high-speed primary highways with paved surfaces and stabilized shoulders. THREE-LANE HIGHWAYS The center lane is either used as two- way center left-turn lane or alternates in the uphill directions as a directional passing lane Left lane for passing lane Middle lane for travel or cruising lane Right lane for slower lane or merging lane FOUR-LANE OR WIDER HIGHWAYS On four-lane highways traffic flows in opposite directions on each pair of lanes, and passing is accomplished within the lanes of forward movement of traffic and not in the lanes of opposing traffic. A four-lane highway in the Philippines typically refers to a road that has two lanes in each direction, making a total of four lanes. These highways are designed to accommodate higher traffic volumes and allow for smoother and faster travel compared to roads with fewer lanes. DIVIDED HIGHWAYS Divided highways need not be of a constant cross section. The median strip may vary in width; the roads may be at difference elevations; and superelevation may be applied separately on each set of lanes. The width of the median strips varies from 4 -ft (1.2m) to 60-ft (18m). At urban and suburban intersections, a desirable median width of 18-ft (5.4m) accommodates a 12-ft (3.6m) turning lane and a 6-ft (1.8m) median separator. LIMITED-ACCESS HIGHWAYS A limited-access highway may be defined as a highway or street especially designed for through traffic, to which motorists and owners of abutting properties have only restricted right of access. In general, the desirable spacing of access points or interchanges along limited-access facilities is 1mi (1,500m) or greater in urban areas and 3 to 5 mi (4 to 7 km) in rural areas. The Veterans Memorial Parkway in London, Ontario is a modern at-grade limited-access road with intersections Pavement Crowns Another element of the highway cross section which is the raising of the centerline of the roadway above the elevation of the pavement edges. Crowns may be formed by intersecting tangent lines or by curved lines that emanate from the road centerline. It is important that the road be constructed with a crown or Curb cross slope to account for surface water drainage. Typically, a crown is placed in the center of the road and the pavement is sloped 2% in each direction. Curb Configurations Curbs help to control water runoff and, in conjunction with the crown in the roadway, direct rainwater to the side of the street where it will not impede traffic. Curbs at parking areas and adjacent to sidewalks should be 6 to 8 in. (150 to 200 mm) in height, with a curb face that is nearly vertical. Curb Typical highway curb sections. (Source: A Policy on Geometric Design of Highways and Streets, copyright 2001, American Association of State Highway and Transportation Officials, Washington, DC. Used by permission.) Shoulders For roads without curbs, it is necessary to provide shoulders for safe operation and to allow the development of full traffic capacity. Emergency vehicles such as ambulances, fire trucks and police cars may use the shoulder to bypass traffic congestion in some countries. A usable outside shoulder width of at least 10-ft (3m) and preferably 12-ft (3.6m) that is clear of all obstructions is desirable for all heavily traveled and high-speed highways. Guardrails A guardrail's purpose is to protect drivers who turn off of the road. The rail is used to prevent drivers from hitting obstacles such as steep embankments, hillsides, utility poles, retaining, bridge pillars, etc. It is safer to hit the guard rail than to hit these obstacles. Cable guardrail A guardrail should be provided where fills are over 8-ft (2.4 m) in height, when shoulder W-beam guardrail slopes are greater than 1V:4H (1 vertical unit for every 4 horizontal units), in locations where is sudden change in alignment, and where a greater reduction in speed is necessary. The most important types of guardrails are; 1. W-beam guardrail 2. Cable guardrail 3. Box beam guardrail Box beam guardrail Drainage Ditches - Drainage ditches should be located and shaped to avoid creating a hazard to traffic safety. - A drainage ditch is a depression in the land created to channel water. - Drainage ditches are typically formed around low-lying areas, roadsides or fields proximate to a water body or created to channel water from a more distant water source for the purpose of plant irrigation. Slopes - The graded area immediately adjacent to the graded roadway shoulder is the side-slope. - Foreslopes and backslopes may vary considerably depending on soil characteristics and the geographic location of the highway. - In general, 1V:2H is most commonly used for a backslope in order to remain within the right-of-way width and to reduce the impact on adjacent properties. Right-of-Way - The right-of-way width for a two-lane highway on secondary roads with an annual average daily traffic volume of 400 to 1,000 vehicles, as recommended by the American Association of State Highway and Transportation Officials, is 66-ft (20m) minimum and 80-ft (25m) desirable. - The right of way is the total land area acquired for the construction of the roadway. Roadway Alignment Horizontal and Vertical Alignment Roadway Alignment The designer must produce an alignment in which conditions are consistent and uniform to help reduce problems related to driver expectancy. Generally, the topography of the surrounding area is fitted into one of three classifications: 1. Level 2. Rolling 3. Mountainous Level Country – the alignment is in general limited by considerations other than grade Rolling Country – grade and curvature must be carefully considered and to a certain extent balanced. Mountainous Country – grades provide the greatest problem, and, in general, the horizontal alignment or curvature is controlled by maximum grade criteria. Roadway geometric design consists of the following fundamental three-dimensional features: Horizontal alignment - tangents and curves Vertical alignment - grades and vertical curves Cross section - lanes and shoulders, curbs, medians, roadside slopes and ditches, sidewalks Alignment - The position or the layout of the central line of the highway on the ground is called the alignment. Highway Alignment includes both a. Horizontal alignment includes straight and curved paths, the deviations and horizontal curves. b. Vertical alignment includes changes in level, gradients and vertical curves. HORIZONTAL ALIGNMENT VERTICAL ALIGNMENT - Corresponds to “x” and “z” - Corresponds to highway length and coordinates. “y” coordinate. - Plan view – Roughly - Presented in a profile view. -equivalent to perspective view of an - Give elevation of all points measured aerial photograph of highway. along of a highway. HORIZONTAL ALIGNMENT The roadway horizontal alignment is a series of horizontal tangents (straight roadway sections), circular curves, and spiral transitions. It shows the proposed roadway location in relation to the existing terrain and adjacent land conditions. HORIZONTAL ALIGNMENT The operational characteristics of a roadway are directly affected by its alignment. The alignment, in turn, affects vehicle operating speeds, sight distances, and highway capacity. The horizontal alignment is influenced by many factors including: Functional Terrain Design speed classification Right-of-way Environmental Traffic volume availability concerns Anticipated level of service Circular Curves - circular curve is an arc with a single constant radius connecting two tangents. Simple circular curve – the most common type of curve used in a horizontal alignment. Compound curve – composed of two or more adjoining circular arcs of different radii. Broken-back curve – the combination of a short length of tangent between two circular curves. Reverse curve – consists of two adjoining circular arcs with the arc centers located on opposite sides of the alignment. Compound and reverse curves are generally used only in specific design situations such as mountainous terrain. VERTICAL ALIGNMENT The vertical alignment of a roadway is controlled by design speed, topography, traffic volumes, highway functional classification, sight distance, horizontal alignment, vertical clearances, drainage, economics, and aesthetics. The vertical alignment (or profile) of the roadway is typically established at the point of rotation. Grades and Grade Control AASHTO recommendations make maximum grades dependent on design speed and the surrounding topography. Present design practice limits grades to 5 percent for a design speed of 70 mph (110 km/hr). For a design speed of 30 mph (50 km/hr), maximum grades typically range from 7 to 12 percent, depending on the roadway classification and the surrounding topography. Vertical Curves - are used to provide a smooth transition between roadway grades. - composed of a parabolic curve that provides a constant rate of change of grade. A parabolic curve “flattens” the curve at the top or bottom of a hill to maximize the driver’s sight distance. When a vertical curve connects a positive grade with a positive grade with a negative grade, it is referred to as a “crest curve”. When a vertical curve connects a negative grade with a positive grade, it is termed a “sag curve”. GEOMETRIC DESIGN OF HIGHWAYS AND RAILWAYS SUPER-ELEVATION AND EARTHWORKS JOHN CARLO FRANCISCO Super-elevation Super-elevation refers to the banking or tilting of the roadway or track on a curve, where the outer edge is raised above the inner edge. This design helps counteract the centrifugal forces that act on vehicles or trains as they navigate a curve, preventing them from skidding outward and improving safety and comfort. Super-elevation Super-elevation refers to the banking or tilting of the roadway or track on a curve, where the outer edge is raised above the inner edge. This design helps counteract the centrifugal forces that act on v ehi c l es or trai ns as they nav i g ate a c urv e, preventing them from skidding outward and improving safety and comfort. Super-elevation Super-elevation is essential in balancing the centrifugal forces that act on vehicles or trains when they travel through a curve. Without super- elevation, the centrifugal force would push the vehicle outward, potentially causing skidding, overturning, or discomfort for passengers Super-elevation e = super elevation formula v= velocity g= gravity (9.81 m/s²) R= radius of curvature f = design coefficient of lateral friction Super-elevation Recommended Values for Super-Elevation Based on Speed Limits: For low-speed roads, super-elevation can be minimal, around 2% to 4%, which is sufficient for gentle curves. For highways, where vehicles are traveling at much higher speeds, super-elevation values can reach up to 6% to 8% or even more, depending on curve sharpness and environmental conditions. Practical Limits: Super-elevation values above 10% are generally avoided to prevent issues such as drainage problems and difficulty for slow-moving or stopped vehicles to stay balanced. Super-elevation Railway Track Super-Elevation (Cant) Cant refers to raising the outer rail higher than the inner rail on curves, allowing trains to safely navigate curves at higher speeds by balancing the centrifugal force. Balancing Comfort, Safety, and Rail Wear Comfort: Reduces lateral forces felt by passengers, ensuring a smoother ride. Safety: Prevents derailment by balancing centrifugal forces that push the train outward on curves. Rail Wear: Evenly distributes forces across both rails, reducing wear and extending track life. Earthworks in Geometric Design Earthworks in Geometric Design Earthworks involve modifying the natural terrain through excavation or addition of soil to prepare the land for construction, ensuring proper alignment, drainage, and stability in highway or railway projects Earthworks in Geometric Design Cutting: Removing earth from higher areas to achieve the desired road or track level. Filling: Adding earth to lower areas to raise the road or railway to the required height. Grading: Smoothing and leveling the terrain to ensure proper drainage and a stable foundation. Embankment Creation: Constructing raised structures of soil to support roads or railways over uneven terrain or water bodies. Earthworks in Geometric Design Earthworks in Geometric Design Stable Foundation: Earthworks provide a stable base for infrastructure, preventing settlement or shifting. Drainage: Proper grading ensures effective water runoff, reducing the risk of erosion or damage to the road or railway. Durability: Well-executed earthworks contribute to the long-term durability and safety of transportation systems Cut and Fill Techniques for Highway Construction Cut and Fill refers to the earth-moving process used to create a flat and stable surface for highway construction by either removing (cutting) material from elevated areas or adding (filling) material to lower areas. The goal is to balance the amount of material cut and used for fill to minimize transportation costs and environmental impact. Consideration of Drainage, Slope Stability, and Erosion Control Drainage: Proper drainage systems are essential to avoid water accumulation, which can weaken the soil and compromise the stability of the highway. Techniques like culverts, ditches, and slope grading are used to channel water away from the road. Consideration of Drainage, Slope Stability, and Erosion Control Drainage: Slope Stability: Ensuring that both the cut and fill slopes are stable is critical to prevent landslides or soil erosion. This can involve: Benching: Creating horizontal steps in steep slopes to prevent large- scale sliding. Retaining Walls: Used in cut areas to hold back soil and provide additional stability. Earthworks for Railways Specific Challenges in Railway Earthworks Precision: Railway tracks require high levels of precision to ensure smooth alignment and stable operation. Even small deviations in the gradient or curvature can lead to discomfort for passengers or impact the stability of the trains, especially at high speeds. Load-bearing: Railways must support heavy, dynamic loads over long periods. Unlike roads, railways experience constant and concentrated load points from train wheels. This makes it crucial to design earthworks that can support significant weight without excessive settlement or deformation. Earthworks for Railways Subgrade Strength The subgrade must be strong to support these loads without excessive deformation: Compaction: Increases soil density and load-bearing capacity. Soil Stabilization: Uses materials like lime or cement to strengthen weak soils. Geosynthetics: Reinforces the subgrade to distribute loads evenly. Earthworks for Railways Trackbed Design The trackbed consists of multiple layers that distribute loads effectively: Ballast: Crushed stone supporting rails and ties, allowing for load distribution and drainage. Sub-ballast: Additional layer providing filtration and support to ballast. Subgrade: The foundation that must be prepared to handle the applied loads. Thank you CHAPTER 5 STRUCTURAL DESIGN OF RAILWAYS E n s u r i n g S a f e t y, E f f i c i e n c y and Sustainability Rabino, Shanley Joy S. Railways are a vital mode of transportation, facilitating the movement of goods and passengers over long distances. The structural design of railways encompasses various elements, including tracks, bridges, tunnels, and stations, all of which must be engineered for safety, efficiency, RAILWAY STRUCTURE and durability. - encompass a wide array of construction intended to support track itself or house railway operations. Components of Railway Structural Design 2.1 Railway Tracks a. Rails: Made from high-strength steel to withstand dynamic loads. Standard rail profiles (e.g., UIC60, UIC54) are used based on the anticipated traffic volume. b. Sleepers (Ties): Materials: Concrete, timber, or steel. Functions: Support and maintain gauge, distribute loads to ballast. c. Ballast: Composed of crushed stone, it provides stability, drainage, and facilitates track alignment. d. Subgrade: The natural ground layer that must be compacted and graded to support the railway structure. Components of Railway Structural Design 2.2 Bridges and Tunnels a. Bridges: Must support significant vertical and lateral loads from trains. Common designs include beam, arch, and suspension bridges, selected based on span and location. b. Tunnels: Design considerations include soil stability, water drainage, and ventilation. Materials often involve reinforced concrete and steel for durability. BRIDGE DECK - Portion of a railway bridge that supplies a means of carrying the track rails. 2 GENERAL TYPES OF BRIDGE DECK 1. Open Bridge Deck 2. Ballasted Bridge Deck 2 GENERAL TYPES OF BRIDGE DECK 1. Open Bridge Deck – the rails are anchored directly to timber bridge ties supported directly on the floor system of the superstructure. 2 GENERAL TYPES OF BRIDGE DECK 2. Ballasted Bridge Deck – the rails are anchored directly national to timber track ties supported in the ballast section. Purpose of BALLAST ❑ Holds the sleepers ❑ Load Distribution ❑ Vibration Dampening ❑ Drainage ❑ Prevent Vegetation around the Tracks ❑ Noise Absorption ❑ Minimizes Heat Expansion Structural Design Structural design is the methodical investigation of the stability, strength and rigidity of structures. A structural design project may be divided into three phases: ❖ Planning ❖ Design ❖ Construction A structural design project may be divided into three phases: ❖ Planning PLANNING ❖ Design - Involves the consideration of the ❖ Construction various requirements and factors affecting the general layout and dimensions of the structure and results in the choice of one or perhaps several alternative types of structure, which offer the best general solution. A structural design project may be divided into three phases: DESIGN ❖ Planning - Involves a detailed consideration of ❖ Design the alternative solutions defined in the ❖ Construction planning phase and results in the determination of the most suitable proportions, dimensions and details of the structural elements and connections for constructing each alternative structural arrangement being considered. A structural design project may be divided into three phases: CONSTRUCTION ❖ Planning - Involves mobilization of personnel; ❖ Design procurement of materials and ❖ Construction equipment, including their transportation to the site, and actual on-site erection. Importance of Proper Structural Design in Railways 1. Enhanced Safety Accident Prevention: Proper design minimizes risks such as derailments and collisions, ensuring passenger and freight safety. Resilience to Hazards: Structures are designed to withstand environmental factors like earthquakes, floods, and high winds. 2. User Comfort and Accessibility Improved Passenger Experience: Enhanced design reduces noise and vibrations, leading to a smoother ride. Inclusivity: Thoughtful design ensures facilities are accessible to all, including individuals with disabilities. 3. Operational Efficiency Smooth Operations: Well-designed tracks facilitate higher speeds and reliability, minimizing delays. Increased Capacity: Efficient designs accommodate more traffic and heavier loads without compromising safety. 4. Cost Effectiveness Reduced Maintenance Costs: Durable materials and designs lead to lower repair and maintenance needs. 5. Regulatory Compliance Adherence to Standards: Ensures compliance with safety and engineering regulations, fostering public trust and reliability. THANK YOU!!! “Life is like a train journey; you can’t always choose the stops, but you can choose how to enjoy the ride.” -Unknown The structural design of pavements involves determining the appropriate thickness and composition of pavement layers to ensure that they can carry traffic loads over time without excessive deterioration. Pavements are typically classified into two main types: flexible pavements and rigid pavements. Each type has different structural behavior and design principles. Pavements are typically classified into two main types 1. FLEXIBLE PAVEMENTS Flexible pavements distribute loads over the subgrade in a way that each layer deforms slightly, spreading the load over a larger area. They are composed of multiple layers of materials that provide support and protection from deformation. COMPONENTS OF FLEXIBLE PAVEMENTS: Surface Course: · The top layer, usually made of asphalt, designed to resist wear, provide a smooth driving surface, and withstand traffic loads. Binder Course: a critical layer in flexible pavements, positioned between the surface course and the base course. It plays an essential role in the pavement structure, providing load distribution and acting as a buffer between the wearing surface and the foundation layers. Base Course: · Provides structural support for the surface layer. It's typically made of crushed stone or gravel and can also be stabilized with cement or asphalt. Subbase Course: · An optional layer between the base course and the subgrade, which adds additional support and drainage. Subgrade: · The natural soil or compacted layer beneath the pavement structure. The quality and strength of the subgrade significantly influence pavement design. DESIGN CONSIDERATIONS FOR FLEXIBLE PAVEMENTS: Traffic Loads: · The expected volume and weight of traffic, usually expressed in equivalent single-axle loads (ESALs). Material Properties: · Strength, durability, and moisture susceptibility of asphalt, base, and subbase materials. Climate: · The effects of temperature variations, freeze-thaw cycles, and moisture on the pavement materials. Drainage: · Proper drainage design to avoid water infiltration, which can weaken the structure. The design of flexible pavements typically follows the empirical method (e.g., the AASHTO Guide for Design of Pavement Structures) or a mechanistic-empirical approach, which uses performance models based on laboratory and field data. Empirical Method Mechanistic-Empirical Approach This more advanced approach combines This method is based on past both mechanistic principles (like stress, performance and experience, with one of strain, and deformation responses of the most widely used guides being the pavement materials) and empirical data to AASHTO Guide for Design of Pavement predict pavement performance. By using Structures. This guide uses data collected performance models grounded in from field experiments to estimate how laboratory testing and real-world field pavements will perform under certain data, this method allows for a more traffic and environmental conditions. The tailored and accurate design. It considers empirical method is straightforward but various factors such as material may lack precision for unique or complex properties, traffic loads, and pavement conditions. environmental conditions to ensure better pavement longevity and efficiency. 2. RIGID PAVEMENTS: Rigid pavements are made of concrete and behave like a slab that spreads loads over a wide area. Unlike flexible pavements, they have high flexural strength and do not rely as much on the layers beneath them for load distribution. COMPONENTS OF RIGID PAVEMENTS: Surface (PCC Slab): Subgrade: · The main load-bearing layer, made of · The natural ground or compacted soil layer Portland Cement Concrete (PCC). It may under the pavement. Like flexible have reinforcing steel or dowel bars to help pavements, subgrade quality is crucial for with load transfer between joints. long-term performance. Base or Subbase Layer: · A granular or stabilized material placed between the slab and the subgrade. It helps improve drainage and provides a uniform support to reduce slab stresses. DESIGN CONSIDERATIONS FOR RIGID PAVEMENTS: Traffic Loads: · The number of ESALs expected during the pavement's service life. Concrete Strength: · The flexural strength of concrete (modulus of rupture) is a key parameter for designing the thickness of the slab. Load Transfer at Joints: · The use of dowels or aggregate interlocks to transfer loads between adjacent slabs, preventing differential settlement. Temperature Effects: · Proper drainage design to avoid water infiltration, which can weaken the structure. Subgrade and Support: : The strength and stability of the base, subbase, and subgrade. Rigid pavement design often follows a mechanistic-empirical approach, like the AASHTO Pavement Design Method, or other methods like the Portland Cement Association (PCA) design guide. AASHTO Pavement Design Portland Cement Association (PCA) Method Design Guide Like its flexible pavement counterpart, This guide offers an alternative, widely this method is based on both mechanistic accepted design approach for rigid principles and empirical data. It accounts pavements. It emphasizes the structural for factors such as traffic loads, material properties of concrete, including its properties, and environmental conditions, stiffness and ability to resist deformation but specifically tailored to rigid pavement under heavy loads. The PCA method structures (usually concrete). The method focuses on slab thickness design, joint models stresses and strains in the spacing, and reinforcement to optimize concrete slabs and joints to predict pavement durability and load-bearing performance and ensure longevity. capacity. 3. DESIGN Pavement design methods consider both traffic loads and environmental METHODS: factors. Common methods include: AASHTO Pavement Design Guide: · Widely used in the U.S., based on empirical data and performance studies. Mechanistic-Empirical Pavement Design Guide (MEPDG): · Combines mechanical modeling of pavement layers with empirical data to predict pavement performance under specific conditions. Other National Standards: · Many countries have their design codes or guidelines for pavement design, such as IRC in India or TRL in the UK. 4. PAVEMENT DESIGN PROCESS: TRAFFIC ANALYSIS: LAYER THICKNESS DESIGN: Determining the thickness of each layer to Estimating the number and type of ensure the pavement can distribute traffic vehicles that will use the pavement over loads without excessive deformation or its design life. failure. MATERIAL SELECTION: DRAINAGE DESIGN Choosing materials for the pavement Ensuring that water is properly managed layers based on load-bearing capacity, to prevent weakening of the pavement durability, and environmental conditions. structure. 5. PERFORMANCE Pavements are designed to resist various types of failures, such as: CONSIDERATIONS: Fatigue Cracking: Due to repeated traffic loads. Rutting: Permanent deformation in the wheel paths of flexible pavements. Thermal Cracking: : Due to temperature fluctuations. Faulting and Joint Deterioration: In rigid pavements due to poor load transfer at joints. Effective pavement design ensures a balance between initial construction costs and long-term maintenance, optimizing the pavement’s lifespan and performance. FOR YOUR ATTENTION