Drugs Targeting Dopamine and Dopamine Receptors - PDF
Document Details
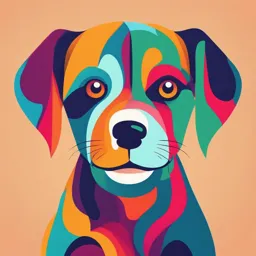
Uploaded by FruitfulIntegral
Wayne State University
Michael Bannon
Tags
Related
- Norepi, Serotonin, Dopamine PDF
- Central Nervous System (CNS) PDF
- Med Org Lec L4.2 PDF: Anti-Seizures and Antidepressants
- 2024 Synaptic Transmission & Neurotransmitters PDF
- Unit 3 - Organisation of the Nervous System: CNS & PNS (Structure and Functions) PDF
- Neuropharmacology Principles - Wong Chun Keung - 16 Sep 2024 PDF
Summary
This document contains lecture notes on drugs that target dopamine and dopamine receptors. Topics include the use of drugs in treating Parkinson's disease, psychosis, and attention deficit hyperactivity disorder. The document reviews the life cycle of dopamine, receptor interactions, and the mechanisms of different drugs.
Full Transcript
L40. Drugs targeting dopamine and dopamine receptors: use in Parkinson’s disease, psychosis, attention deficit hyperactivity disorder, and sleep disorders Michael Bannon PhD ([email protected]) Comments and questions via Canvas are preferred, for the benefit of your colleagues Learning objecti...
L40. Drugs targeting dopamine and dopamine receptors: use in Parkinson’s disease, psychosis, attention deficit hyperactivity disorder, and sleep disorders Michael Bannon PhD ([email protected]) Comments and questions via Canvas are preferred, for the benefit of your colleagues Learning objectives -Understand the basics of how DA is made, stored, and inactivated -Learn the classes of drugs that restore DA transmission, either directly or indirectly, in Parkinson’s disease -Understand the feature of antipsychotics most critical to therapeutic efficacy. Learn specific drugs mentioned. -Understand how amphetamines, methylphenidate, and cocaine augment DA transmission -Gain a broad understanding of the role of DA in reward and substance use disorder Lecture outline Life cycle of DA Synthesis and storage Means of inactivation DA receptors- families and their signaling Central DA pathways Mechanisms of DA drugs used in the treatment of Parkinson’s disease Drugs that affect DA levels Drugs that bypass DA to work on DA receptors Mechanisms of DA drugs used in the treatment of schizophrenia Role of D2 receptor blockade First vs second generation (i.e. atypical) antipsychotics Mechanisms of amphetamines in the treatment of attention deficit hyperactivity disorder and sleep disorders Mechanisms of action of amphetamines Use of amphetamines and other stimulants for sleepiness, cognitive enhancement, etc. Dopamine in reward mechanisms and substance use disorder Effects of drugs of abuse on DA neurotransmission Role of DA in reward and substance use disorders L40. Drugs targeting dopamine and dopamine receptors: use in Parkinson’s disease, psychosis, attention deficit hyperactivity disorder, and sleep disorders In the last two lectures, you’ve heard primarily about neurotransmitters that act via interaction with ionotropic receptors (i.e. fast glutamate- and GABA-mediated transmission). In the next few lectures, we’ll focus on neurotransmitters that are co-released with GABA or glutamate and serve as neuromodulators via their actions of metabotropic (G protein-coupled) receptors. Perhaps foremost among these modulatory neurotransmitters-- at least in terms of targeting by CNS drugs-- is dopamine (DA). DA and/or its receptors are targeted in the treatment of Parkinson’s disease, schizophrenia and other psychotic disorders, attention deficit hyperactivity disorder, excessive sleepiness, and other disorders of CNS function, accounting in total for about 1 in every 6 of CNS drug prescriptions. The DA system also mediates the properties of stimulants that underlie their abuse potential. In this lecture, we will briefly review the ‘life cycle’ of DA, its interaction with DA receptors, its regulation of physiological functions, and mechanisms of action of DA-based therapeutics. Life cycle of DA DA is a catecholamine neurotransmitter. It is converted to norepinephrine (NE) or epinephrine in some other cell types, but is the final neurotransmitter product in central DA neurons. Tyrosine hydroxylase is the first and rate-limiting enzyme in DA biosynthesis. Aromatic amino acid decarboxylase is more ubiquitous and the final biosynthetic step (Fig 1). Fig 1. DA biosynthesis Once made, DA is accumulated to very high local concentrations within storage vesicles via the vesicular monoamine transporter 2 (VMAT2; Fig 2). VMAT2 uses a vesicular proton gradient (attained by an ATPase) to counter-transport protons out of, and DA into, the vesicle. Upon depolarization of the DA nerve terminal, DA- containing vesicles release their content into the extracellular space. DA’s effects are terminated in large measure by an inward DA-sodium co-transport via the DA transporter (DAT), but also by metabolism (via monoamine oxidase [MAO] and catecholamine-O-methyltransferase [COMT]), and by diffusion (Figs 2,3). Recaptured DA can be reaccumulated by VMAT2 into vesicles for reuse. Fig 3. DA metabolism Fig 2. DA synapse As you will see in the next lecture, NE is derived from DA, and serotonin (5HT) is derived from another aromatic amino acid. There is high degree of relatedness in terms of the biosynthesis, storage, release, reuptake, and metabolism of these three monoamines, so it is unsurprising that many drugs that affect these processes in DA neurons can, to varying degrees, affect the same processes for the NE or 5HT neurons. Further, at a systems level, the major DA, NE, and 5HT projection neurons all originate in the midbrain or brainstem to innervate vast areas of the forebrain and have overlapping modulatory effects on brain function. DA receptors During its relatively brief life in the extracellular space, DA may interact with one or more of the five DA receptors (Fig 4). The family of D1 receptors (D1R) (i.e. D1 and D5) may be broadly thought of as ‘excitatory’ (increase in second messenger production) while the family of D2 receptors (D2R) (i.e. D2, D3, D4) may be thought of an ‘inhibitory’ (decrease cell excitability). D1R and D2R predominate in brain. The interest in the other DA receptors lies primarily in their rather unique distributions of CNS expression, which implies unique functional roles. Fig 4. DA receptors Central DA pathways Some DA cells and receptors occur within discrete cells in local circuits within the retina and olfactory bulb. There are also DA receptors in the area postrema outside the BBB (which mediate emesis). Short DA projections are found within various parts of the hypothalamus and are involved in regulation of hormones (e.g. prolactin and growth hormone). By far, the quantitatively and clinically most important group of DA cells originate within the midbrain (substantia nigra and adjacent ventral tegmental area) to send widespread innervations to forebrain regions such as the cortex (to modulate cognition), limbic areas (to regulate motivation, goal-directed thinking, affect, reward) and the basal ganglia (to modulate learning & execution of purposeful movement) (Fig 5). Fig 5. DA pathways It is important to remember that we do not have drugs that specifically affect DA or its receptors in any one particular DA system. When we target one of these DA pathways, we can predict that adverse side effects may arise from effects on the other DA systems. We will discuss this a bit more below. You will learn the pathophysiology of various CNS disorders later- here we provide only a brief overview of the mechanisms by which various drugs alter DA neurotransmission to affect the expression of disease symptoms. Mechanisms of DA drugs used in the treatment of Parkinson’s disease DA is robustly expressed in the basal ganglia, a brain region involved in motor control. Profound loss of the majority of DA cells innervating the basal ganglia underlies the symptoms of Parkinson’s disease (Fig 6). Fig 6. DA treatment of PD Fig 7. Dopa-related drugs Any treatment that can boost CNS DA levels or signaling an provide symptomatic treatment of Parkinson’s disease (Fig 6): L-dopa: Gets converted to DA in the CNS, especially when used in combination with carbidopa, a peripheral inhibitor of conversion to DA. One of earliest examples of rational drug development, it is now often reserved for use when other DA-based treatments are less effective. Dopa and related drugs are shown in Fig 7. COMT inhibitors: (e.g. Entacapone). Prevent peripheral metabolism of administered dopa. Used as an adjunct. MAO inhibitors: (e.g. Selegiline). Prevent breakdown of residual DA in the CNS. Used as an adjunct. D2R/D3R agonists: (e.g. ropinirole, pramipexole). Bypass DA biosynthesis to directly activate DA receptors. As shown in Fig 8, this drug category has become the first line treatment of choice (because of a reduced side effect profile compared to l-dopa). Based on the existence of other, non-basal ganglia DA systems, you might correctly predict that trying to replace basal ganglia DA with a DA-based drug therapy can lead to off-target adverse effects, such as cognitive changes (too much cortical DA), Fig 8. Drugs changes in mood and motivation (too much limbic DA), nausea used in PD and emesis (DA receptor stimulation in area postrema outside the BBB), etc. Mechanisms of DA drugs used in the treatment of schizophrenia Schizophrenia is a debilitating psychotic disorder(s) characterized by some combination of delusions, hallucinations, thought disorders, apathy, and emotional blunting. You will learn more about this topic later. Fig 9. Site of action of antipsychotics Evidence for involvement of DA systems in schizophrenia is nearly completely pharmacological in nature (Fig 9): -DA-releasing drugs such as amphetamines (see below) can exacerbate or elicit some schizophrenic symptoms, even in previously non-psychotic individuals. -All effective antipsychotic drugs share the property of being D2R antagonists (or, in the case of aripiprazole, a weak partial D2R agonist). Some newer (so called ‘atypical’ or ‘second generation’ antipsychotic drugs)(e.g. quetiapine, aripiprazole, risperidone) interact with other monoamine receptors (e.g. 5HT2A) in addition to D2R. These newer drugs now completely dominate the market, even though large clinical studies proved that none were more effective than the older, generic antipsychotic drugs (e.g. haloperidol). The exception is clozapine, the ‘original’ atypical antipsychotic that has unique therapeutic efficacy in treatment-resistant cases but also high toxicity, neither of which have been seen with subsequent ‘me-too’ atypical knock-offs. Although abnormalities of DA signaling are unlikely to be a primary cause of psychosis, DA receptor antagonists remain an important mainstay in the treatment of schizophrenia. Some atypical antipsychotics are also used as adjunctive treatments for bipolar disorder and other mood disorders. Again, you might not be surprised to learn that when trying to block DA signaling in higher brain areas, concurrent blockade of DA receptors in the basal ganglia can lead to a number of disturbances in movement (e.g. Parkinson’s-like symptoms early on and a type of dyskinesia after extended treatment). Finally, it is worth noting that, although D2R blockade is essentially immediate upon administration of these drugs (Fig 9), full therapeutic effects take weeks to months to achieve- obviously some downstream cellular and circuit changes must be involved in clinical responses. Mechanisms of amphetamines in the treatment of attention deficit hyperactivity disorder and sleep disorders Drugs that increase DA neurotransmission have been deployed in the treatment of a wide variety of symptoms or disorders, despite limited data suggesting a direct role of DA in underlying pathophysiology. A case in point is attention deficit hyperactivity disorder, a commonly diagnosed behavioral disorder marked by inattentiveness, impulsivity and, often, hyperactivity, for which an equivalent number of genetic and brain imaging studies have supported or refuted the involvement of DA function (and particularly the DAT). Fig 10. Amphetamine mechanisms of action This controversy about the role of DAT notwithstanding, DA-enhancing drugs such as various amphetamines and methylphenidate remain treatments of choice for attention deficit hyperactivity disorder. Amphetamines (see Fig 10) gain access to DA terminals via DAT transport (#2). Intracellular amphetamines then gain access to vesicles via VMAT2 transport, causing the release of vesicular DA stores into the DA terminal (#1). Free DA within the DA nerve endings can also be increased due to some MAO inhibition by amphetamines (#4). Reverse transport by the DAT then increases extracellular DA and accumulates up more amphetamine (#3). Methylphenidate works differently, increasing synaptic DA by blocking DAT-mediated DA reuptake. Structural similarities between amphetamines (and to a lesser extent, methylphenidate) and DA are shown in Fig 11. Fig 11. Structures of DA, amphetamines, and methylphenidate The well-known ability of DA-potentiating drugs (such as the amphetamines) to increase alertness, decrease fatigue, and suppress appetite has led to the prescribed or illicit use of these compounds for many decades. Amphetamines are still employed in the medical treatment of several sleep disorders, including narcolepsy and shift work sleep disorder. Modafinil is a newer stimulant which appears to work at least partially through a DA-augmenting mechanism and is used for similar indications. Stimulants in general, and modafinil in particular, have been touted as cognitive enhancers in healthy individuals, though the bulk of the evidence suggests that they may enhance performance (when used in low doses) only indirectly, by increasing alertness. The widespread use of DA-augmenting drugs for attention deficit hyperactivity and various other indications is such that stimulant drugs now account for nearly 1 in 10 CNS drug prescriptions, with amphetamine, methylphenidate, and their congeners accounting for about 90% of the market share (Fig 12). Fig 12. Stimulant drug prescriptions Dopamine in reward mechanisms and substance use disorder Drugs of abuse are both rewarding (i.e. they are interpreted by the brain as intrinsically positive) and reinforcing (i.e. they increase the probability that behaviors leading to drug access will be repeated). Since these drugs can overpower the brain’s response to natural reinforcers (e.g. food, water and sex), animals (including humans) will, under some conditions, self-administer drugs to the exclusion of eating and drinking, even to the point of death. Repeated drug use can thus lead to substance use disorder, i.e., compulsive drug seeking and consumption, in spite of diminished positive rewarding properties over time and the development of serious negative consequences. Fig 13. Relationship between brain Although drugs of abuse typically have multiple sites of drug levels and subjective effect action, recent evidence strongly suggests that all of them share a common action of augmenting DA release, particularly within the forebrain nucleus accumbens and caudate-putamen (see Fig 5). Cocaine directly blocks the DAT (similarly to methylphenidate), thereby increasing extracellular DA. As discussed above (Fig 10), amphetamines accumulate in DA nerve terminals, where they displace vesicular DA, and raise free intraneuronal DA, which is then released via reversal of the DAT. Other drugs of abuse as diverse as nicotine, ethanol, marijuana and heroin increase the rate of discharge of DA neurons through various synaptic inputs. Importantly, the rewarding properties of drugs are related in part to their rate of rise and decline within the CNS, which is thought to be why the oral use of stimulants for attention deficit disorder does not have the same abuse potential as intravenous, smoked, or snorted stimulants (Fig 13). Interestingly, DA neurons may play a role in obesity associated with food addiction. So DA neurons respond to acute exposure to drugs of abuse. But they also respond to anticipation of reward, and to differences between an anticipated reward and the reward actually received (i.e. reward-prediction error; Fig 14); this helps the organism to update estimates of future reward. Critically, DA cells respond to environmental cues that have become associated with past drug exposure (e.g. the sight of drug paraphernalia or drug-using associates). Ironically, responses to these cues strengthen over time, even as responses to the DA-augmenting drugs themselves attenuate. Drug addiction is now thought of as a form of learning and memory, with an increased focus on the long-term cellular and molecular changes that may underlie the critical problem of Fig 14. Relationship between conditioned relapse into drug use, seen even after prolonged drug abstinence. stimulus, reward/no reward and DA cell activity