HAP The Muscular System PDF
Document Details
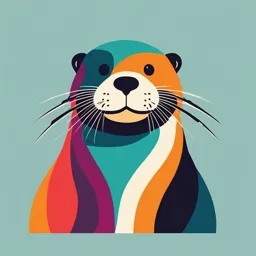
Uploaded by CoolestMood
Centro Escolar University
Tags
Related
- Essentials of Human Anatomy & Physiology PDF
- Essentials of Human Anatomy & Physiology Chapter 6 PDF
- Essentials of Human Anatomy & Physiology Chapter 6 PDF
- Human Anatomy and Physiology: The Muscular System PDF
- Human Anatomy and Physiology - Chapter 09 - Muscles and Muscle Tissue PDF
- Muscles PDF - General Human Anatomy
Summary
This document provides a detailed overview of the human muscular system, encompassing different muscle types (skeletal, cardiac, smooth), their functions, and the associated anatomy. It also covers the mechanical characteristics and properties of muscles.
Full Transcript
Types of Muscles Skeletal attached to bones striated voluntarily controlled Cardiac located in the heart striated involuntarily controlled Smooth Loca...
Types of Muscles Skeletal attached to bones striated voluntarily controlled Cardiac located in the heart striated involuntarily controlled Smooth Located in blood vessels, hollow organs Non-striated involuntarily controlled 1 The Muscular System Functions 1. Movement 2. Maintain posture 3. Respiration 4. Production of body heat 5. Communication 6. Constriction of organs and vessels 7. Contraction of the heart Figure 7.1 2 Functional Properties of Muscles Contractility - the ability of muscle to shorten forcefully, or contract Excitability - the capacity of muscle to respond to a stimulus Extensibility - the ability to be stretched beyond its normal resting length and still be able to contract Elasticity - the ability of the muscle to recoil to its original resting length after it has been stretched 3 Whole Skeletal Muscle Anatomy 1 Skeletal muscle, or striated muscle, with its associated connective tissue, constitutes approximately 40% of body weight. Also called striated muscle because transverse bands, or striations, can be seen in the muscle under the microscope. Individual skeletal muscles, such as the biceps brachii, are complete organs, as a result of being comprised of several tissues: muscle, nerve, and connective tissue. 4 Organization of Skeletal Muscle Sarcomere: Fascicle: units of Muscle Muscle myofibrils belly a bundle of Fiber: responsible for muscle fibers muscle cell the striated appearance Myofibrils: Myosin: Myofilament: structures thick protein that make filaments filaments that up a muscle make up a Actin: thin fiber sarcomere filaments Muscle fiber = muscle cell Skeletal Muscle Fiber Anatomy 1 A muscle fiber is a large cell, with several hundred nuclei located at its periphery. Muscle fibers range in length 1 mm to 30 cm. Alternating light and dark bands give muscle fibers a striated appearance. The number of muscle fibers remains constant after birth so enlargement of muscles results from an increase in the size of muscle fibers, not an increase in fiber number. 7 Connective Tissue Coverings Each skeletal muscle is surrounded by a connective tissue sheath called the epimysium. A skeletal muscle is subdivided into groups of muscle cells, termed fascicles. Each fascicle is surrounded by a connective tissue covering, termed the perimysium. Each skeletal muscle cell (fiber) is surrounded by a connective tissue covering, termed the endomysium. 8 Electrical Component Structures 1 The sarcolemma (cell membrane) has many tubelike inward folds, called transverse tubules, or T tubules. T tubules occur at regular intervals along the muscle fiber and extend into the center of the muscle fiber. The T tubules are associated with enlarged portions of the smooth endoplasmic reticulum called the sarcoplasmic reticulum. 9 Electrical Component Structures of the Muscle 2 The enlarged portions are called terminal cisternae. Two terminal cisternae and their associated T tubule form a muscle triad. The sarcoplasmic reticulum has a relatively high concentration of Ca2+, which plays a major role in muscle contraction. The cytoplasm of a muscle fiber is called the sarcoplasm, which contains many bundles of protein filaments. 10 Structure of Skeletal Muscle Figure Access the text alternative for slide images 7.2 11 Mechanical Component Structures 12 Mechanical Component Structures Bundles of protein filaments are called myofibrils. Myofibrils consist of two types of myofilaments, actin (thin filaments) and myosin (thick filaments). Actin and myosin are arranged into repeating units called sarcomeres. Actin + myosin = sarcomeres The myofilaments in the sarcomere provide for the mechanical aspect of muscle contraction. 13 Sarcomere: The Breakdown Sarcomere is the basic structural unit of skeletal muscle. Sarcomeres join end to end to create myofibrils. Z disks are network of protein fibers that serve as an anchor for actin myofilaments and separate one sarcomere from the next. A sarcomere extends from one Z disk to the next Z disk. 14 Sarcomere: The Breakdown Sarcomere: The Breakdown of Striations Main Protein Filaments THICK FILAMENTS: MYOSIN Composed of myosin proteins. Myosin has a head and a tail. Head = binds to actin Tail = aggregates to form the thick filament. THIN FILAMENTS: ACTIN Primarily composed of actin proteins. Actin filaments are intertwined with each other (g-actin and f-actin) to form a double-stranded helix. When relaxed, the myosin binding site within actin are blocked by tropomyosin. 17 Myofilament Structure: The Actin Actin myofilaments (thin) are made up of 3 components: 1. Actin 2. Troponin 3. Tropomyosin. Troponin- binds with actin molecules have binding sites for Ca2+ tropomyosin filaments block the myosin myofilament binding sites on the actin myofilaments. 18 Myofilament Structure The Myosin Myosin myofilaments, or thick myofilaments, resemble bundles of tiny golf clubs. Myosin heads have ATP binding sites, ATPase and attachment spots for actin. 19 Skeletal Muscle Fiber (a) SPL/Getty Images Figure Access the text alternative for slide images 7.3 20 Now that we know all the structures involved, how do the muscles move? Where do we start? 21 It all starts with the motor neuron! Then continues through the neuromuscular junction! Then finally in the myofibrils! Where the following happens: 1. Excitation of muscle – Action Potential 2. Neuromuscular junction – Synapses 3. Muscle Contraction- Sliding Filament Theory 22 Excitation of Muscle Fibers: Muscles get excited too! What is a Nerve Impulse? Also known as an action potential, is a rapid electrical signal that travels along the length of a neuron (nerve cell), then to the muscle fiber. The fundamental way that information is transmitted throughout the nervous system. 23 Understanding the Resting Membrane Potential and Action Potential Imagine a cell as a battery. Just like a battery has a positive and negative terminal, a cell has a difference in charge across its membrane. The inside of the cell is generally negatively charged compared to the outside. Why does this charge difference exist? 1. Ion Distribution: The cell has different amounts of ions (charged particles) inside and outside. More potassium (K+) inside More sodium (Na+) outside. 2. Membrane Permeability: The cell membrane is more permeable to potassium than sodium. This means potassium can move in and out more easily. 3. Negative Charges: Proteins and other molecules inside the cell are negatively charged and can't easily leave. 24 The Resting Membrane Potential: The Default State This charge difference is called the resting membrane potential. It's like the battery's voltage. It's maintained by the sodium-potassium pump, which works to keep potassium inside and sodium outside. 25 The Action Potential: A Temporary Change A stimulus (nerve signal), can cause the membrane to become more permeable to sodium. Sodium rushes in, making the inside more positive. This is called depolarization. To restore the balance, potassium channels open, and potassium flows out. The membrane potential returns to its resting state. This is called repolarization. Think of it like a domino effect: One change triggers a chain of events that eventually brings the cell back to its starting point. 26 The Action Potential: A Temporary Change A stimulus (nerve signal), can cause the membrane to become more permeable to sodium. Sodium rushes in, making the inside more positive. This is called depolarization. To restore the balance, potassium channels open, and potassium flows out. The membrane potential returns to its resting state. This is called repolarization. Think of it like a domino effect: One change triggers a chain of events that eventually brings the cell back to its starting point. 27 The Action Potential: A Temporary Change TO UNDERSTAND MORE, YOU CAN WATCH THIS VIDEO: https://www.youtube.com/watch?app=desktop&v=iBDXOt_uHTQ&fbcli d=IwY2xjawF6liFleHRuA2FlbQIxMAABHRKrS3DaNqO3oQayZ4lDBSbSEE A2k9VChrOYRZhzG3CCyDUQOkrSAlIzow_aem_pcuGa6y8hOQcOhfCBra abw 28 The Action Potential: A Temporary Change 29 Now, we proceed with the Neuromuscular Junction! Neuromuscular Junction: A Bridge Between Nerve and Muscle The specialized synapse where a motor neuron transmits a signal to a muscle fiber, initiating muscle contraction. The point of communication between the nervous system and the muscular system. 30 The Structure of the Neuromuscular Junction Motor neuron - a nerve cell that stimulates muscle cells. Neuromuscular junction - a synapse where a neuron connects with a muscle fiber. Synapse - the cell-to-cell junction between a nerve cell and either another nerve cell or an effector cell, such as in a muscle or a gland. Motor unit - a group of muscle fibers that a single motor neuron stimulates. 31 The Structure of the Neuromuscular Junction Presynaptic membrane – the end of a neuron cell axon fiber. Synaptic cleft - the space between the presynaptic terminal and postsynaptic membrane. Postsynaptic membrane - the muscle fiber membrane (sarcolemma). Synaptic vesicle - a vesicle in the presynaptic terminal that stores and releases neurotransmitter chemicals. 32 The Structure of the Neuromuscular Junction Neurotransmitters are chemicals that stimulate or inhibit postsynaptic cells. Acetylcholine is the neurotransmitter that stimulates skeletal muscles. 33 How Does Neuromuscular Junction Work? 1. In the motor neuron: When the action potential arrives, voltage-gated sodium channels open, allowing sodium ions (Na+) to rush into the cell. This influx of positive ions depolarizes the neuron. 2. At the neuromuscular junction: When the action potential reaches the terminal end of the motor neuron, it triggers the opening of voltage- gated calcium channels, allowing calcium ions (Ca2+) to enter the cell. This influx of calcium ions stimulates the release of acetylcholine from the synaptic vesicles into the synaptic cleft. 34 How Does Neuromuscular Junction Work? 3. Acetylcholine Binding: Acetylcholine diffuses across the synaptic cleft and binds to nicotinic receptors. 4. Muscle Action Potential: This binding causes ion channels to open, allowing sodium ions to enter and potassium ions to leave the muscle fiber, generating an electrical signal. 5. Muscle Contraction: This muscle action potential travels along the muscle fiber, triggering the release of calcium ions from intracellular stores and ultimately leading to muscle contraction. 35 The Action Potential: A Temporary Change TO UNDERSTAND MORE, YOU CAN WATCH THIS VIDEO: https://www.youtube.com/watch?v=zbo0i 1r1pXA 36 Now, let’s proceed to the Myofibril: The Sliding Filament Model 1. Nerve Impulse: A nerve impulse travels down a motor neuron to the neuromuscular junction, where it stimulates the release of acetylcholine (ACh). ACh binds to receptors on the muscle fiber's sarcolemma, causing an action potential to propagate across the membrane and into the T-tubules. 2. Calcium Release: The action potential triggers the release of calcium ions (Ca2+) from the sarcoplasmic reticulum (SR) into the sarcoplasm. 37 Sliding Filament Model 3. Muscle Contraction: 3.1 Exposure of Actin Binding Sites: Calcium ions bind to troponin, a protein complex associated with actin filaments. This binding causes tropomyosin, another protein that covers the actin binding sites, to move away, exposing the sites. 3.2 Myosin Head Attachment: Myosin heads, which are energized by ATP hydrolysis, bind to the exposed actin binding sites, forming cross-bridges. Energy Note: ATP Hydrolysis: ATP is broken down into ADP (adenosine diphosphate) and inorganic phosphate (Pi) by the myosin head. This reaction releases energy. 38 Sliding Filament Model 3.3 Power Stroke: The myosin heads then exert a force, pulling the actin filaments towards the center of the sarcomere. This movement is known as the power stroke. 3.4 ATP Binding and Detachment: ATP binds to the myosin head, causing it to detach from the actin filament. The myosin head is now ready to bind to another actin binding site. 3.5 Cycle Repetition: This process repeats, with the myosin heads continuously binding, pulling, detaching, and rebinding, causing the actin filaments to slide over the myosin filaments and shorten the sarcomere. 39 Sliding Filament Model 4. Muscle Relaxation: When the nerve impulse ceases, calcium ions are pumped back into the SR. With the removal of calcium ions, troponin and tropomyosin return to their original positions, covering the actin binding sites. Myosin heads detach from the actin filaments, and the muscle relaxes. 40 Sliding Filament Model: The Summary 41 Overall Summary of the Muscle Contraction 42 Sliding Filament Model 43