Glycolysis_TCA 2022 Past Paper PDF
Document Details
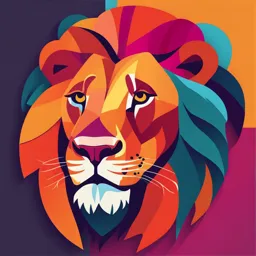
Uploaded by SumptuousSugilite7063
RCSI Medical University of Bahrain
2022
Royal College of Surgeons in Ireland
Salim Fredericks
Tags
Summary
This Royal College of Surgeons in Ireland, Medical University of Bahrain 2022 biochemistry past paper covers glycolysis and the tricarboxylic acid cycle. The paper includes learning objectives, diagrams, and questions related to energy generation in aerobic and anaerobic settings. The document covers glucose metabolism, and the roles of important enzymes in these processes.
Full Transcript
Royal College of Surgeons in Ireland Medical University of Bahrain Biochem - Glycolysis and the Tricarboxylic Acid Cycle Salim Fredericks LEARNING OBJECTIVES 1. Outline Glycolysis and TCA Cycle in the overall map of metabolism 2. Describe glucose entry into cells and metabolism of gluco...
Royal College of Surgeons in Ireland Medical University of Bahrain Biochem - Glycolysis and the Tricarboxylic Acid Cycle Salim Fredericks LEARNING OBJECTIVES 1. Outline Glycolysis and TCA Cycle in the overall map of metabolism 2. Describe glucose entry into cells and metabolism of glucose. 3. Describe the alternative metabolic uses of pyruvate 4. Describe the significance of the pyruvate dehydrogenase complex in health and disease 5. Describe the TCA cycle’s reaction sequence 6. Describe the regulation of glycolysis the TCA cycle 7. Identify the steps at which chemical energy is captured in glycolysis and TCA cycle 8. Outline the energy yield (as ATP) from the catabolism of glucose under aerobic & anaerobic conditions. Relate glycolysis and TCA cycle to the overall map of metabolism Why is glucose metabolism important? Glucose & related sugars are important components of the diet e.g. breakdown product of carbohydrates such as starch Some tissues can only use glucose as a metabolic fuel e.g. brain, erythrocytes, renal medulla, cornea, testes, exercising muscle Therefore, the body has to maintain constant glucose levels in circulation (4.5 - 5.6 mmol/L; fasting) The context of glycolysis and TCA Overview of catabolism Lippencott’s 8.2 Major Pathways in Carbohydrate Metabolism Major Pathways in Carbohydrate Metabolism Describe glucose entry into cells Glucose uptake into cells Glucose is a polar molecule, therefore it requires transport proteins to facilitate its movement through membranes. There are two classes of transporter proteins : facilitated diffusion via GLUT Carriers - facilitated diffusion (ATP independent) - GLUT 1-4 - expressed on most cells Cotransporters - secondary active transport - expressed only in intestinal and kidney epithelial cells secondary active transport via sodium-glucose symporter http://www.ncbi.nlm.nih.gov/bookshelf/picrender.fcgi?book=cooper&part=A1986&blobname=ch12f17.jpg http://www.apsu.edu/thompsOnj/Anatomy%20&%20Physiology/2020/2020%20Exam%20Reviews/Exam%204/pumpanim.gif Glucose uptake into cells Distribution Name Notes Facilitative bidirectional transporters GLUT1 all cells; (especially RBCs and Basal uptake brain) renal tubular cells, intestinal high capacity, low affinity; GLUT2 epithelial cells, liver cells, pancreatic Post-prandial uptake and β cells sensing GLUT3 neurons high-affinity regulated by insulin; GLUT4 adipose tissues, striated muscle responsible for insulin- (skeletal muscle, cardiac muscle). regulated glucose storage. Sodium-dependent unidirectional transporter Active uptake of glucose SGLT 1 Small intestine and kidney against a concentration gradient Retention of glucose in cells Once inside, Glucose is trapped inside the cell by phosphorylation, converting it to Glucose-6-phosphate (there are no cell membrane transporters for phosphorylated sugars) Phosphorylation is catalyzed by kinases: hexokinase – in all cells glucokinase – liver parenchymal cells, pancreatic islet cells Hexokinase vs glucokinase enzymatic properties Hexokinase -low Km : high affinity for glucose even at low concentrations -low Vmax : limited capacity Glucokinase (hexokinase –D) -high Km : only operates efficiently at high glucose concentrations -high Vmax : high capacity, allowing it to handle the high glucose levels occurring post- prandially Metabolism of glycose Stages of glycolysis Phosph’n of glucose. 2 x C3 from 1 x C6 ATP generation Still a C6 molecule glucose is trapped and destabilized glucose → fructose 1,6 bisphosphate by: A phosphorylation An isomerisation Another phosphorylation This is readily split into two C3 molecules two inter-convertible C3 from a C6 Triose phosphate isomerase readily converts: Dihydorxyacetone phsophate (DHAP) Glyceraldehyde-3-P Generation of ATP Glycer-3-P → 1,3-BPG: Glycer-3-P-DH NAD → NADH 1,3-BPG → 3-PG: BP-Kinase ADP → ATP PEP → pyruvate: pyruvate-Kinase ADP → ATP Describe the alternative metabolic uses of pyruvate Other metabolic fates of pyruvate - Conversion to Acetyl-CoA which can enter the TCA Cycle or serve as a precursor for Fatty-acid synthesis [Pyruvate Dehydrogenase complex] - Conversion to Oxaloacetate which can enter the TCA Cycle or serve as a precursor for Gluconeogenesis [Pyruvate Carboxylase] - Reduction to Ethanol [Pyruvate Decarboxylase] Identify the reaction steps at which regulatory processes occur in glycolysis Regulatory enzymes act as metabolic “valves” Irreversible reactions are: Hexokinase Phospho- fructokinase Pyruvate kinase Control of glycolysis - Hexokinase This catalysers the first reaction of glycolysis: This reaction regulates entry into the glycolysis pathway and in effect entry into to the cell High conc. of G-6-P signals to the cell that more glucose is not needed Glycolysis regulation Glucose + ATP glucose-6-phosphate Hexokinase irreversible Inhibited by its reaction product, glucose-6-P Low Km for glucose (high affinity) – operates efficiently at low glucose conc Glucokinase (hexokinase D) irreversible Only in liver and pancreatic islet cells low affinity for glucose (high Km)* ; operates best at higher glucose concentration Not inhibited by G-6-P Functions to sequester glucose post-prandially *In fact, does not follow michaelis-menton kinetics so it is strictly incorrect to talk about Km in the case of glucokinase Control of glycolysis - phosphofructokinase Phosphofructokinase is the most important control element in mammalian glycolysis: High levels of ATP allosterically inhibit PFK Increased AMP (ie lower ATP) increases the activity of PFK Glycolysis regulation Fructose-6-P fructose-1,6-bisphosphate Phosphofructokinase (PFK1) Irreversible Most important control point, rate limiting step Allosteric inhibition by ATP, allosteric activation by AMP Allosteric inhibition by citrate Hormonal: PFK1 Activated by fructose 2,6-bisphosphate Levels of F-2,6-BP , increased by insulin, decreased by glucagon F-2,6-BP also acts as an inhibitor of gluconeogenesis (prevents ‘futile cycling’) Glycolysis regulation Phosphoenol pyruvate + ADP pyruvate + ATP Pyruvate kinase (physiologically irreversible) Activated by fructose-1,6-bisphosphate (an earlier glycolytic intermediate) – feed forward regulation Inactivated by glucagon mediated phosphorylation Significance of the pyruvate dehydrogenase complex in health and Pyruvate dehydrogenase Pyruvate dehydrogenase Localization Glycolysis occurs in the cytoplasm. The TCA Cycle occurs in the mitochondrion. The reaction cycle occurs in the mitochondrial matrix. The mitochondrial membrane is impermeable to charged molecules. How does pyruvate enter the mitochondria? Pyruvate dehydrogenase A specific Pyruvate Transporter exists in the inner mitochondrial membrane. In the matrix: Pyruvate Acetyl-CoA by Pyruvate Dehydrogenase Complex - a multi-enzyme complex - contains three distinct enzyme activities Pyruvate decarboxylase (“dehydrogenase”) Dihydrolipoyl transacetylase Dihydrolipoyl dehydrogenase - contains 5 coenzymes Pyruvate dehydrogenase An irreversible reaction glucose cannot be made from Acetyl-CoA Allosterically inhibited by Acetyl-CoA NADH Pyruvate dehydrogenase complex The association of the three enzymes means that the intermediates are passed from one active-site to the next without being released Pyruvate dehydrogenase requires coenzymes Pyruvate Dehydrogenase & clinical problems? [a] Pyruvate Dehydrogenase Deficiency An inherited (X-linked) genetic defect which results in congenital lactic acidosis. Pyruvate converted to Lactic-acid, NOT Acetyl-CoA Symptoms: - developmental defects (especially of the CNS) - muscular spacticity - early death X No effective therapy exists Pyruvate Dehydrogenase & clinical problems? [b] Arsenic poisoning Arsenic is an inhibitor of enzymes that use lipoic-acid as a cofactor Therefore, it will inhibit pyruvate dehydrogenase Symptoms: - neurological disturbances life123.com - death Pyruvate Dehydrogenase & clinical problems? [c] Vitamin deficiencies Panthotenic acid Coenzymes require vitamins for their synthesis: CoA Panthotenic acid (B5) NAD Niacin (B3) Niacin FAD Riboflavin (B2) TPP Thiamine (B1) Deficiency in any of these vitamins impair pyruvate dehydrogenase function Riboflavin Symptoms: Pyruvate, lactate & alanine levels (pyruvate reduced to lactate or transaminated to alanine) Severe lethargy & fatigue Complications affecting the cardiovascular, nervous, Thiamine muscular, & gastrointestinal systems. TPP = Thiamine pyrophosphate The Tricarboxylic Acid Cycle Cellular respiration - overview Dietary NADH carbohydrates are broken down to glucose monomers NADH other monosaccharides PDH are converted to glucose, or enter cellular respiration at different points Fats are broken down to acetyl CoA in the mitochondrion cellular respiration involves the controlled release of energy stored in carbohydrate and fat The reactions of Tricarboxylic Acid Cycle in sequence The Tricarboxylic Acid (TCA) cycle Main functions: Energy yield Metabolic intermediates Location: The TCA cycle occurs in the mitochondrial matrix (mostly) TCA reactions “Tricarboxylic acid” Citrate Synthase Acetyl CoA + oxaloacetate citrate Activated by Ca2+, ADP Inhibited by ATP, NADH, succinyl CoA Aconitase Citrate isocitrate TCA reactions Isocitrate dehydrogenase Energy! Isocitrate α-ketoglutarate (+ CO2 + NADH) Oxidative decarboxylation of isocitrate. A rate limiting step of the TCA Activated by ADP, Ca2+ Inhibited by ATP, NADH TCA reactions Energy! α-ketoglutarate dehydrogenase complex α-ketoglutarate succinyl CoA (+ CO2 + NADH) Oxidative decarboxylation of α-ketoglutarate 3 enzyme complex Coenzymes: thiamine pyrophosphate, lipoic acid, FAD, NAD+, CoA – Analogous to PDH, with which it shares a subunit Activated by Ca2+ Inhibited by ATP, NADH, GTP, Succinyl CoA TCA reactions Succinate Thiokinase Energy! Succinyl CoA Succinate (+ GTP) substrate level phosphorylation coupled to production of one ATP Succinate dehydrogenase Energy! Succinate fumarate (+ FADH2) – This enzyme is embedded in the inner mitochondrial membrane and is part of the electron transport chain. The FADH2 produced directly enters the ETC, passing its electrons to CoQ TCA reactions Fumarase Fumarate malate Energy! Malate dehydrogenase Malate oxaloacetete (+ NADH) Pyruvate Enzymes of dehydrogenase the TCA Citrate synthase Malate aconitase dehydrogenase fumarase Isocitrate dehydrogenase Succinate dehydrogenase α-ketoglutarate Succinate dehydrogenase complex thiokinase Energy generation in TCA Overall yield and energetics of the TCA cycle 2 carbons enter as Acetyl CoA and two (different ones) leave as CO2 One molecule of GTP is formed directly Four electron pairs are transferred from the cycle to generate 3 NADH and 1 FADH2 – The electrons will ultimately generate ATP through the process of oxidative phosphorylation Direct yield per Theoretical* ATP Acetyl CoA yield 3 NADH 9 1 FADH 2 1 GTP 1 total 12 Regulation of TCA Regulation of the TCA cycle inhibitors activators NADH citrate synthase ATP ADP succinyl CoA isocitrate NADH dehydrogenase ATP ADP, Ca++ NADH αKG succinyl CoA dehydrogenase ATP Ca++ complex GTP Outline the energy yield (as ATP) from the catabolism of glucose under aerobic & anaerobic With regards to energy generation and consumption glycolysis should be seen as having two stages Aerobic vs. Anaerobic Aerobic metabolism is much more efficient than anaerobic metabolism This is because it allows the complete oxidation of fuel molecules to CO2 and H2O Anaerobic metabolism: glucose pyruvate Aerobic metabolism: glucose CO2 + H2O ATP production ATP is produced in two ways: [a] directly – substrate-level phosphorylation (ATP generation) eg: phosphoglycerate kinase reaction in glycolysis [b] indirectly – oxidative phosphorylation (NADH+H+ & FADH2 generation) they act as carriers of energy in the form of ‘reducing power’ (electrons) Conversion in the electron transport chain to ATP: NADH +H+ 3 ATP FADH2 2 ATP Aerobic vs. anaerobic glycolysis Figure 8.9 Lippincott: Biochemistry Energy from aerobic glycolysis ATP ATP 2x 2x 2x ATP 2x ATP Energy from anaerobic glycolysis ATP ATP 2x 2x ATP lactate 2x ATP ATP production Anaerobic metabolism Glucose Glycolysis Pyruvate 1 glucose 2 pyruvate = 8 ATP 2 pyruvate 2 lactate (-6 ATP) Lactate 1 Glucose2 lactate =2 ATP Aerobic metabolism Glucose Glycolysis Pyruvate TCA Cycle & 1 glucose Oxidative produces a Phosphorylation max. of 38 ATP CO2 + H2O Energy Yield from cellular respiration Anaerobic glycolysis yields 2 ATP per glucose (the NADH is used up converting pyruvate to lactate, but this recycles the NAD) Aerobic glycolysis yields 2 ATP and 2 NADH per glucose Pyruvate Dehydrogenase: 2 NADH per glucose TCA cycle yields 6 NADH, 2 FADH2 and 2 GTP per glucose Oxidative phosphorylation yields 2.5 ATP per NADH, 2ATP per FADH2 Overall yield from 1 glucose for aerobic respiration : 33 ATP* (30-38)