Glutamatergic & GABAergic Pharmacology 10-2024 PDF
Document Details
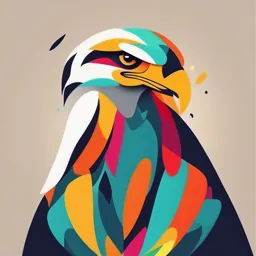
Uploaded by GaloreRhodium8872
University of Kansas
2024
Liqin Zhao, PhD
Tags
Related
- Pharmacology Unit 4 lecture 4.pdf
- Pharmacology of the Central Nervous System PDF
- Corrected_Final_20231106_Glutamatergic and GABAergic Neurotransmission_Sedatives_Hypnotics.pdf
- CNS Pharmacology III - PDF
- Pharmacology of Dopamine week 8 PDF
- Pharmacology of Serotonergic and Central Adrenergic Neurotransmission PDF
Summary
These are lecture notes on Glutamatergic and GABAergic Pharmacology from Fall 2024 at the University of Kansas.
Full Transcript
Glutamatergic & GABAergic Pharmacology LIQIN ZHAO, PHD Malott 2046 [email protected] Fall 2024 CNS Pharmacology Nearly all CNS drugs produce their therapeutic effects by modifying certain steps in neurotransmission ...
Glutamatergic & GABAergic Pharmacology LIQIN ZHAO, PHD Malott 2046 [email protected] Fall 2024 CNS Pharmacology Nearly all CNS drugs produce their therapeutic effects by modifying certain steps in neurotransmission CNS Pharmacology 1. action potential 2. affect synthesis 3. storage in vesicle 4. inhibit metabolism 5. affect release 6. affect uptake (into terminal or glia) 7. inhibit degradation 8. affect receptor 9. receptor influences ion flow 10. retrograde signaling CNS Pharmacology Glutamate GABA Dopamine Serotonin Norepinephrine Acetylcholine Anti‐ND Sedative‐ Anti‐PD drugs Antidepressants Anti‐AD drugs drugs Hypnotics Antipsychotics Anti‐BD drugs Anti‐ADHD drugs AEDs Drugs of abuse Anti‐OCD drugs Anesthetics Anti‐migraine drugs CNS Pharmacology Ion Channels (Na+, K+, Ca2+) AEDs Analgesics/Opioids Anesthetics Glutamatergic & GABAergic Pharmacology Physiologic / pathophysiologic effects and pharmacologic modulation of neurotransmission mediated by Glutamate / GABA Study Objectives 1. Physiology of glutamatergic neurotransmission 2. Pathophysiology and pharmacology of glutamatergic neurotransmission 3. Physiology and pathophysiology of GABAergic neurotransmission 4. Drugs affecting GABAergic neurotransmission Glutamate Metabolism Synthesis: In glutamatergic nerve terminals, -ketoglutarate glutamate by GABA transaminase (GABA-T) Storage: Packaged into synaptic vesicles by H+-ATPase/vesicular glutamate transporter (VGLUT) Glutamate Metabolism Release: In response to an action potential [Ca2+]i exocytosis Glutamate Metabolism Deactivation: - Reuptake into presynaptic nerve terminals by neuronal Gt(n) transporters - Uptake into glial cells by glial Gt(g) transporters - In glial cells, Glutamate (Glu) glutamine (Gln) by glutamine synthetase; - Gln transferred to presynaptic nerve terminals by Gln transporters; - Gln Glu by mitochondria- associated glutaminase Glutamate Receptors Ionotropic glutamate receptors – 3 Subtypes: AMPA, kainate, NMDA – Glutamate-gated Na+, K+, Ca2+ channels – Fast response; excitatory Metabotropic glutamate receptors (mGluRs) – 8 Subtypes: Group I (2), Group II (2), Group III (4) – Affect ion channels through second messengers – Slow response; final effects depend on the type of ion channels Ionotropic Glutamate Receptors Ionotropic Glutamate Receptors Glutamate Kainate AMPA NMDA (-amino-3-hydroxy-5-methyl-4- (N-methyl-D-aspartate) isoxazole propionic acid) Ionotropic Glutamate Receptors Subtype Location Subunits Agonists Actions AMPA-R Throughout the GluR1 Glutamate; Na+ influx CNS, particularly GluR2 AMPA (major effect) hippocampus and GluR3 K+ efflux cerebral cortex GluR4 Kainate-R Throughout the GluR5 Glutamate; Na+ influx CNS, particularly GluR6 kainate (major effect) hippocampus and GluR7 K+ efflux cerebellum KA1 KA2 NMDA-R Primarily NR1 Glutamate; Ca2+ influx hippocampus, NR2A NMDA; (major effect) cerebral cortex, NR2B + Glycine K+ efflux spinal cord NR2C + membrane NR2D depolarization Metabotropic Glutamate Receptors Metabotropic Glutamate Receptors Gs – Activates adenylyl cyclase (AC) cAMP PKA Gi – Inhibits adenylyl cyclase (AC) cAMP PKA Gq – Activates phospholipase C (PLC) IP3 and DAG [Ca2+]i and PKC cAMP: cyclic adenosine monophosphate PKA: protein kinase A PIP2: phosphatidylinositol‐4,5‐bisphosphate IP3: inositol‐1,4,5‐trisphosphate DAG: diacylglycerol PKC: protein kinase C Metabotropic Glutamate Receptors Postsynaptic modulation: subunit AC / PLC subunits K+ efflux / Ca2+ influx Metabotropic Glutamate Receptors Presynaptic negative feedback modulation: [Ca2+]i glutamate release Metabotropic Glutamate Receptors Group Subtype Actions I mGluR1 Postsynaptic: mGluR5 Activate AC cAMP PKA neuronal activity Activate PLC IP3 and DAG [Ca2+]i and PKC neuronal activity Inhibit K+ channels K+ efflux neuronal excitability II mGluR2 Postsynaptic: mGluR3 Inhibit AC cAMP PKA neuronal activity Activate K+ channels K+ efflux neuronal excitability Presynaptic: Inhibit AC cAMP PKA neuronal activity Inhibit Ca2+ channels Ca2+ influx glutamate release neuronal excitability III mGluR4 Presynaptic: mGluR6 Inhibit AC cAMP PKA neuronal activity mGluR7 Inhibit Ca2+ channels Ca2+ influx glutamate release neuronal excitability mGluR8 Glutamatergic Neurotransmission Study Objectives 1. Physiology of glutamatergic neurotransmission 2. Pathophysiology and pharmacology of glutamatergic neurotransmission 3. Physiology and pathophysiology of GABAergic neurotransmission 4. Drugs affecting GABAergic neurotransmission Pathophysiology of Glutamatergic Neurotransmission Excitotoxicity Glutamate release or glutamate uptake synaptic glutamate [Ca2+]i Ca2+-dependent degradation enzymes & mitochondrial damage cell death Neurodegenerative diseases [e.g., Alzheimer’s disease (AD), Parkinson’s disease (PD), Huntington’s disease (HD), amyotrophic lateral sclerosis (ALS)] Stroke and trauma Hyperanalgesia Epilepsy Pharmacology of Glutamatergic Neurotransmission Clinical applications remain limited Riluzole – ALS Voltage-gated Na+ channel blocker Na+ conductance action potential glutamate release Memantine – AD NMDA receptor antagonist Ca2+ influx Amantadine – PD NMDA receptor antagonist Ca2+ influx; used in combination with levodopa reduces the severity of dyskinesia in PD by 60% Lamotrigine – refractory seizures Stabilizes the inactivated state of voltage-gated Na+ channel action potential glutamate release Felbamate – refractory seizures NMDA receptor antagonist Ca2+ influx Pharmacology of Glutamatergic Neurotransmission 1, 2, 4, 5 = acetylcholinesterase (AChE) inhibitors FDA Recently Approved Anti-AD Immunotherapies July 6, 2023: Lecanemab (Eisai/Biogen), an anti-amyloid monoclonal antibody, received an accelerated approval in January 2023, followed by a traditional approval in July 2023 by the FDA, for treating patients with mild cognitive impairment or mild dementia stage of AD, and with confirmed presence of amyloid pathology. Administered intravenously every two weeks Boxed warning: amyloid-related imaging abnormalities (ARIA) FDA Recently Approved Anti-AD Immunotherapies July 2, 2024: Donanemab (Eli Lilly), the second anti-amyloid monoclonal antibody, was approved by the FDA for treating patients with mild cognitive impairment or mild dementia stage of AD, and with confirmed presence of amyloid pathology. Administered intravenously every four weeks. Boxed warning: amyloid-related imaging abnormalities (ARIA) Study Objectives 1. Physiology of glutamatergic neurotransmission 2. Pathophysiology and pharmacology of glutamatergic neurotransmission 3. Physiology and pathophysiology of GABAergic neurotransmission 4. Drugs affecting GABAergic neurotransmission GABA Metabolism Synthesis: In GABAergic nerve terminals, glutamate GABA by glutamic acid decarboxylase (GAD) Storage: Packaged into synaptic vesicles by H+-ATPase /vesicular GABA transporter (VGAT) Release: In response to an action potential [Ca2+]i exocytosis GABA Metabolism Deactivation: System A transporter (SA) - Reuptake into presynaptic System N transporter (SN) nerve terminals - Uptake into glial cells by GABA transporters (GAT) In glial cells: GABA succinic semialdehyde (GABA transaminase; GABA-T) succinic acid (SSA dehydrogenase) Krebs cycle -ketoglutarate glutamate (GABA-T) glutamine presynaptic nerve terminals GABA Receptors Ionotropic GABA receptors – GABAA and GABAC – GABA-gated Cl- channels – Fast response, inhibitory Metabotropic GABA receptors – GABAB – Affect ion currents through second messengers – Slow response, mixed actions GABAA Receptors The most abundant GABA receptors in the CNS Pentamers / 16 subunits (1- 6, 1-3, 1-3, , , , ) / 20 subunit combinations Synaptic GABAA receptors: 2 , 2 , and 1 subunit Extrasynaptic GABAA receptors: 2 , 2 , and 1 or (instead of ) subunit GABAA Receptors GABA binding sites: 2 extracellular at interface between and subunits 2 x GABA bind to GABA binding sites [Cl-]i hyperpolarization / inhibitory postsynaptic potential Modulatory sites: endogenous ligands / drugs / do not directly regulate the gating of the receptor channel GABAA Receptors Inhibition of GABAA receptors seizures Mutations in GABAA receptor subunits inherited human epilepsies Enhanced GABAA receptor activity neuronal excitability impairment of CNS functions GABAC Receptors Pentameric Cl- channels Subunits 1-3 (not found in GABAA receptors) Primarily localized in the retina GABAB Receptors GPCRs Primarily localized in the spinal cord Expressed both presynaptically and postsynaptically Presynaptic negative feedback modulation: [Ca2+]i GABA release postsynaptic neuronal excitability GABAB Receptors Postsynaptic inhibitory modulation: subunit inhibits AC / PLC neuronal activity subunits K+ efflux / Ca2+ influx neuronal excitability GABAergic Neurotransmission Study Objectives 1. Physiology of glutamatergic neurotransmission 2. Pathophysiology and pharmacology of glutamatergic neurotransmission 3. Physiology and pathophysiology of GABAergic neurotransmission 4. Drugs affecting GABAergic neurotransmission GABAergic Drugs Inhibitors of GABA metabolism GABAA receptor agonists and antagonists GABAA receptor modulators GABAB receptor agonists and antagonists Nonprescription uses of agents that affect GABA physiology GABAergic Drugs Clinical applications: Sedation (anxiolysis) Hypnosis (sleep induction, general anesthesia) Control of seizures Neuroprotection following stroke or head trauma Others Muscle spasm Alcohol withdrawal syndrome Inhibitors of GABA Metabolism Tiagabine Inhibits GABA transporter, GAT-1 Blocks GABA reuptake synaptic GABA GABAergic transmission Used in the treatment of epilepsy Inhibitors of GABA Metabolism Vigabatrin (-vinyl GABA) Inhibits GABA transaminase, GABA-T Blocks GABA to succinate semialdehyde vesicular GABA GABAergic transmission Used in the treatment of epilepsy GABAA Receptor Agonists and Antagonists GABAA Receptor Agonists and Antagonists Muscimol Found in hallucinogenic Amanita muscaria Full agonist at many GABAA receptor subtypes *Bicuculline Competitive antagonist at GABA binding sites *Picrotoxin Noncompetitive inhibitor that blocks the Cl- channel pore *Induce seizures and used only for research GABAA Receptor Modulators Benzodiazepines Chlordiazepoxide – discovered by Leo Sternbach (American chemist) in 1955; marketed by Roche in 1960 Diazepam – marketed by Roche in 1963 Benzodiazepines Pharmacologic effects Bind at the interface of and subunits on GABAA receptors containing 1, 2, 3, or 5 subunits (requiring a histidine residue) – “benzodiazepine receptors” Enhance GABAA receptor Cl- channel gating in response to GABA Cl- conductance postsynaptic neuronal excitability Potentiate the inhibitory effects of GABA Benzodiazepines Pharmacologic effects Enhance maximal activation by GABAA partial agonists Directly activate certain mutant GABAA receptors – weak agonist Benzodiazepines Clinical applications Sleep enhancers Anxiolytics Sedatives Antiepileptics Muscle relaxants Treatment of alcohol withdrawal symptoms In the short term: well-tolerated, relatively safe and effective In the long-term: potential for development of tolerance, dependence, addiction Benzodiazepines Drug Clinical Uses Duration of Action Clorazepate Anxiety Short-acting Seizure disorders (3-8 hrs) Alcohol withdrawal syndrome Midazolam Anxiety Short-acting Sedation (general anesthetic) (3-8 hrs) Alprazolam Anxiety Intermediate-acting (Xanax) Panic disorder (11-20 hrs) Lorazepam Anxiety Intermediate-acting (Ativan) Insomnia (11-20 hrs) Seizure disorders Premedication for anesthetic procedure Benzodiazepines Drug Clinical Uses Duration of Action Chlordiazepoxide Anxiety Long-acting (1-3 days) (Librium) Alcohol withdrawal syndrome Clonazepam Panic disorder Long-acting (1-3 days) (Klonopin) Seizure disorders Diazepam Anxiety Long-acting (1-3 days) (Valium) Sedation (general anesthetic) Seizure disorders Muscle spasm Alcohol withdrawal syndrome Clobazam Anxiety Long-acting (1-3 days) Lennox-Gastaut syndrome Less sedating and fewer negative effects on cognition Benzodiazepines Drug Clinical uses Duration of action Triazolam Insomnia Short-acting (3-8 hrs) Estazolam Insomnia Intermediate-acting (11-20 hrs) Temazepam Insomnia Intermediate-acting (11-20 hrs) Flurazepam Insomnia Long-acting (1-3 days) Quazepam Insomnia Long-acting (1-3 days) Benzodiazepines Flumazenil Benzodiazepine antagonist – treats benzodiazepine overdose In patients with benzodiazepine dependence – induces severe withdrawal syndrome Does not block the effects of barbiturates or ethanol Benzodiazepines Metabolized by hepatic P450 enzymes, especially CYP3A4 Many metabolites remain pharmacologically active *active metabolites Benzodiazepines Z-Drugs (Zolpidem (Ambien), Zaleplon, Eszopiclone) Treatment of insomnia Bind to the benzodiazepine binding site on GABAA receptors containing 1 subunit -- Diazepam overdose can be reversed by flumazenil Technically not benzodiazepines (structural difference) Clinical applications not as diverse as classical benzodiazepines Tolerance and amnesia still an issue Zolpidem Benzodiazepines Data suggest that for patients with zolpidem blood levels of about 50 ng/mL, driving skills may be sufficiently impaired to cause an accident Recent pharmacokinetic trials of the drug, which included 250 men and 250 women, found that 15% of women and 3% of men had zolpidem concentrations higher than 50 ng/mL about 8 hours after taking a 10-mg dose; concentrations exceeded 90 ng/mL in some women and at least 1 man Women eliminate zolpidem more slowly than men Extended-release formulations of zolpidem increased risk of having levels of the drug after 8 hours that are potentially impairing 8 hours after a 12.5-mg extended-release dose, 33% of women and 25% of men had concentrations greater than 50 ng/mL and 5% of patients had levels of 100 ng/mL or higher Risk was less (but not zero) among individuals taking a 6.25-mg dose of extended- release zolpidem, with 15% of women and 5% of men 10% of elderly having suprathreshold blood concentrations of the drug 8 hours after taking the drug Recommended doses for women are being lowered from 10 mg to 5 mg for immediate-release zolpidem and from 12.5 mg to 6.25 mg for extended-release formulations Source: Bridget M. Kuehn, MSJ JAMA 2013;309(7):645-646 Barbiturates Barbiturates Barbituric acid – synthesized by German chemist Adolf von Baeyer in 1864; no direct effect on the CNS Barbital – marketed by Bayer in Barbituric acid Barbital 1904; used as a sleeping aid until mid-1950s Phenobarbital – marketed by Bayer in 1912 >2500 derivatives with pharmacologically active properties have been made Phenobarbital Diazepam Barbiturates Pharmacologic effects Widespread effects in the CNS Enhance the inhibitory efficacy of GABA Not limited to GABAA receptors with specific subunit combinations In the brainstem, suppress reticular activating system sedation, amnesia, loss of consciousness In the spinal cord, relax muscles and suppress reflexes Barbiturates Pharmacologic effects Anesthetic barbiturates (e.g., pentobarbital): enhancers of GABAA responses to GABA agonists at GABAA receptors Anticonvulsant barbiturates (e.g., phenobarbital) far less direct agonism on GABAA receptors At clinically relevant concentrations: enhancement of GABA-induced agonism > direct GABAA agonism Barbiturates Pharmacologic effects Affect receptors involved in excitatory neurotransmission AMPA activation by glutamate neuronal excitability At anesthetic concentrations, pentobarbital decreases the activity of voltage-gated Na+ channels, inhibiting high-frequency neuronal firing neuronal excitability Barbiturates Pharmacologic effects Enhancement of GABA- induced agonism: efficacy: barbiturates benzodiazepines potency: benzodiazepines > barbiturates Overdoses of benzodiazepines are deeply sedating but rarely life-threatening Overdoses of barbiturates may produce profound hypnosis, respiratory depression, coma, and death Barbiturates Clinical applications General anesthesia Antiepileptics Neuroprotection Barbiturates Metabolized by hepatic P450 enzymes (CYP3A4, CYP3A5, CYP3A7) – upregulation by chronic use – tolerance and cross-tolerance Reversal of overdose (e.g., phenobarbital ) – IV NaHCO3 increases clearance Synergistic potentiation – concomitant use of barbiturates and other CNS depressants (e.g., ethanol) Physical dependence – barbiturate withdrawal syndromes (e.g., tremors, anxiety, insomnia, CNS excitability) – life threatening Barbiturates Drug Clinical uses Duration of action Methohexital Anesthesia induction and short-term Ultrashort-acting maintenance (5-15 mins) Thiopental Anesthesia induction and short-term Ultrashort-acting maintenance, (5-15 mins) emergency seizure treatment Amobarbital Preoperative sedation, Short-acting emergency seizure treatment (3-8 hrs) Pentobarbital Preoperative sedation, Short-acting emergency seizure treatment (3-8 hrs) Secobarbital Preoperative sedation, Short-acting emergency seizure treatment (3-8 hrs) Phenobarbital Treatment of seizures: the oldest still Long-acting commonly used anti-seizure drug – for (53-118 hrs) all types except absence seizures –– first-line treatment of neonatal seizures Other GABAA Receptor Modulators Etomidate / Propofol Act selectively at GABAA receptors containing 2 and 3 subunits At low concentrations – potentiate GABAA receptor Etomidate activation by GABA At high concentrations – GABAA agonists Used for rapid induction of IV general anesthesia Propofol GABAB Receptor Agonists Baclofen GABAB receptor activation postsynaptic K+ efflux Used in the treatment of spasticity associated with motor neuron diseases (e.g., multiple sclerosis) or spinal cord injury Overdose: hypotension, cardiac and respiratory depression, and coma Withdrawal syndromes: treated with benzodiazepines, propofol Other Agents that Affect GABA Physiology Ethanol Acts on multiple targets including GABAA and glutamate receptors GABAA-mediated Cl- influx NMDAR-mediated excitatory effects Synergistic inhibition (with other sedatives, hypnotics, antidepressants, anxiolytics, anticonvulsants, and opioids) Tolerance – changes in GABAA receptor function Alcohol withdrawal syndromes – treated with benzodiazepines GABAergic Sedative-Hypnotic Drugs GABAergic Sedative-Hypnotic Drugs GABAergic Sedative-Hypnotic Drugs https://drugabuse.com/benzo‐epidemic‐a‐killer‐hiding‐in‐the‐shadow‐of‐opioids/ Conclusions and Future Directions GABA and glutamate represent the major inhibitory and excitatory neurotransmitter in the CNS, respectively. Most drugs that act on GABAergic neurotransmission enhance GABAergic activity and thereby depress CNS functions. Modulation of GABAergic transmission can occur either presynaptically or postsynaptically. Drugs acting at presynaptic sites primarily target GABA synthesis, degradation, and reuptake. Drugs acting postsynaptically affect GABA receptors directly: – By occupying the GABA binding sites (agonists/antagonists) – By an allosteric mechanism (modulators) Conclusions and Future Directions Each of the three main GABA receptor types has a distinct pharmacology: – GABAA receptors are targeted by the largest number of drugs, including GABA binding site agonists, benzodiazepines, barbiturates, general anesthetics, and neuroactive steroids. – GABAB receptors are currently targeted by only a few therapeutic agents, which are used to treat spasticity. – GABAC receptors have not yet been developed as a target of pharmacologic agents. To improve safety and reduce adverse effects, including ataxia, tolerance, and physical dependence, development of new anxiolytics and sedatives has aimed for low-efficacy compounds (e.g., benzodiazepines) as well as compounds with selective activity at GABAA receptor subtypes. Conclusions and Future Directions Animal models with selectively mutated GABAA receptor subunits have revealed that sedation/hypnosis is produced by enhancing the activity of receptors containing 1 subunit. In contrast, anxiolysis is produced by modulation of 2- or 3-containing receptors, and amnesia is associated with 5-containing receptors. There is also evidence for distinct pharmacology and physiology of synaptic GABAA receptors containing different subunits. In the future, highly specific antagonists for glutamate receptor subtypes could potentially protect the CNS in stroke, prevent hyperalgesia and tissue trauma, and treat epileptic seizures.