GEY 212.docx
Document Details
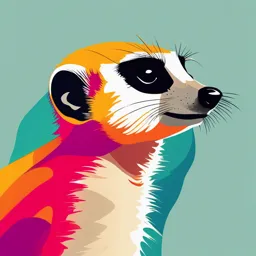
Uploaded by BrainyClover8124
Olabisi Onabanjo University
Tags
Full Transcript
**GEY 212** **STRATIGRAPHY (Concepts and Lithostratigraphy)** **GEOLOGICAL TIME** Time in geology is a bit like distance in astronomy: the numbers are so vast that it is difficult to make much sense of them. Periods of tens of years are easy to comprehend, because we experience them, and centurie...
**GEY 212** **STRATIGRAPHY (Concepts and Lithostratigraphy)** **GEOLOGICAL TIME** Time in geology is a bit like distance in astronomy: the numbers are so vast that it is difficult to make much sense of them. Periods of tens of years are easy to comprehend, because we experience them, and centuries are not so difficult, but once we start dealing with thousands of years our concept of the passage of these amounts of time becomes increasingly divorced from our life experience. So when a geologist refers to a million years, and then tens and hundreds of millions and ultimately billions of years, we have no reference points with which to gauge the passing of those lengths of time. However, a million years is a relatively short period in the history of the Earth, which is about 4.5 billion years. As we go further and further back in geological time, dating something to within a million years becomes more and more difficult. When considering a geological 'event', such as the position of a succession of sandstones or limestones, we may refer to it as having happened over, for example, 4 million years, but in doing so we are talking about something which occurred over a period which is longer than we can realistically imagine. The geologist therefore has to develop a peculiar sense of time, and may consider 100,000 years as a 'short' period, even though it is unimaginably long when compared with our everyday life. The passage of time since the formation of the Earth is divided into **geochronological units** and these are divisions of time that may be referred to in terms of years or by name. The Permian Period, for example, was the time between 299 and 251 million years before present. The abbreviations used for dates are 'Ma' for millions of years before present and 'ka' for thousands of years before present. The time thousands of millions of years before present is abbreviated to 'Ga' (Giga years). The North American Stratigraphic Code (North American Commission on Stratigraphic Nomenclature 1983) suggests that to express an interval of time of millions of years in the past abbreviations such as 'my', 'm.y.' or 'm.yr' could be used. This convention has the advantage of distinguishing 'dates' from 'intervals of time' but it is not universally applied. **Geological time units** It has commonly been the practice to distinguish between **geochronology**, which is concerned with geological time units and chronostratigraphy, which refers to material stratigraphic units. The difference between these is that the former is an interval of time that is expressed in years, whereas the latter is a unit of rock: for example, the Chalk strata in northwest Europe form a part of the Cretaceous System, a unit of rock, and they were deposited in shallow seas which existed in the area during a period of time that we call the Cretaceous Period, an interval of time. There is a hierarchical set of terms for geochronological units that has an exact parallel in chronographic units (Fig. 19.1), but the distinction between the two sets of terminologies is not made by all geologists and some (e.g. Zalasiewicz et al. 2004) question whether it is either useful or necessary to employ this dual stratigraphic terminology. The argument for maintaining both is that it provides a distinction between the physical reality of the strata themselves, the rocks of, say, the Silurian System, and the more abstract concept of the time interval during which they were deposited, which would be the Silurian Period. However, as Zalasiewicz et al. (2004) point out, the use of **'golden spikes'** (see below) for stratigraphic correlation means that the beginning and end of the period of time are now defined by a physical point in a succession of strata, and thus there is no real need to distinguish between the 'time unit' (geochronology) and the 'rock unit' (chronostratigraphy) as they amount to the same thing. The terms for the geochronological units are described below, with the equivalent chronostratigraphic units also noted where they are also in common use. C:\\Users\\DELL\\Pictures\\19.9.JPG **Eons** These are the longest periods of time within the history of the Earth, which are now commonly divided into three eons: the **Archaean Eon** up to 2.5 Ga, the **Proterozoic Eon** from 2.5 Ga to 542 Ma (together these constitute the **Precambrian**), and the **Phanerozoic Eon** from 542 Ma up to the present. **Eras** Eras are the three time divisions of the Phanerozoic: **The Palaeozoic Era** up to 251 Ma, the **Mesozoic Era** from then until 65.5 Ma and finally the **Cenozoic Era** up to the present. Precambrian eras have also been defined, for example dividing the Proterozoic into the Palaeoproterozoic, the Mesoproterozoic and the Neoproterozoic. **Periods/Systems** The basic unit of geological time is the period and these are the most commonly used terms when referring to Earth history. **The Mesozoic Era**, for example, is divided into three periods, the **Triassic Period, the Jurassic Period** and the **Cretaceous Period**. The term system is used for the rocks deposited in this time, e.g. the Jurassic System. **Epochs/Series** Epochs are the major divisions of periods: some have names, for example the Llandovery, Wenlock, Ludlow and Pridoli in the Silurian, while others are simply Early, (Mid-) and Late divisions of the period (e.g. Early Cretaceous and Late Cretaceous). The chronostratigraphic equivalent is the series, but it is important to note that the terms Lower, Middle and Upper are used instead of Early, Middle and Late. As an example, rocks that belong to the Lower Triassic Series were deposited in the Early Triassic Epoch. Logically a body of rock cannot be 'Early', nor can a period of time be considered 'Lower' so it is important to employ the correct adjective and use, for example, 'Early Jurassic' when referring to events which took place during that time interval. **Ages/Stages** The smallest commonly used divisions of geological time are ages, and the chronostratigraphic equivalent is the stage. They are typically a few million years in duration. For example, the Oligocene Epoch is divided into the **Rupelian** and **Chattian** Ages (the Rupelian and Chattian Stages of the Oligocene Series of rocks). Chrons are short periods of time that are sometimes determined from palaeomagnetic information, but these units do not have widespread usage outside of magnetostratigraphy (21.4). The Quaternary can be divided into short time units of only thousands to tens of thousands of years using a range of techniques available for dating the recent past, such as marine isotope stages (21.5). **19.1.2 Stratigraphic charts** The division of rocks into stratigraphic units had been carried out long before any method of determining the geological time periods had been developed. The main systems had been established and partly divided into series and stages by the beginning of the 20th century by using stratigraphic relations and biostratigraphic methods. Radiometric dating has provided a time scale for the chronostratigraphic division of rocks. The published geological time scales (Fig. 19.2) have been constructed by integrating information from biostratigraphy, magnetostratigraphy and data from radiometric dating to determine the chronostratigraphy of rock units throughout the Phanerozoic. A simplified version of the most recent version of the international stratigraphic chart published by Gradstein et al. (2004) is shown in Fig. 19.2 (Gradstein & Ogg 2004). This shows the names of the stratigraphic units that have been agreed by the International Commission on Stratigraphy and the ages, in millions of years, of the boundaries between each unit. The ages shown are based on the best available evidence and are not definitive. it is often difficult to directly measure the ages of a body of sedimentary rocks in terms of millions of years. Strata are normally defined stratigraphically as being, for example, 'Oxfordian' on the basis of the fossils that they contain the physical relationships that they have with other rock units. The time interval of the Oxfordian, 161.2 Ma to 155.0 Ma, that is shown on the chart is subject to change as new information from radiometric dating becomes available, or a recalibration is carried out. Older versions of these  Fig. 19.2 A stratigraphic chart with the ages of the different divisions of geological time. (Data from Gradstein et al. 2004). stratigraphic charts show different ages for boundaries, and no doubt future charts will also contain modifications to these dates. **Golden spikes** From the foregoing it should be clear that the Cambrian, for example, is not defined as the interval of time between 542 Ma and 488.3 Ma, but those numbers are the ages that are currently thought to be the times when the Cambrian Period started and ended. It is therefore necessary to have some other means of defining all of the divisions of the geological record, and the internationally accepted approach is to use the 'Global Standard Section and Point' (GSSP) scheme, otherwise known as the process of establishing 'golden spikes' Some of the periods of the Phanerozoic were originally named after the areas where the rocks were first described in the 18th and 19th centuries: the Cambrian from Wales (the Roman name of which was Cambria), the Devonian from Devon, England, the Permian after an area in Russia and the Jurassic from the Jura mountains of France. (Others were given names associated with a region, such as the Ordovician and Silurian Periods that have their origins in the names of ancient Welsh tribes, and some have names related to the character of the rocks, such as the Carboniferous, coal bearing, and Cretaceous, from the Latin for chalk). This effectively established the principle of a 'type area', a region where the rocks of that age occur that could act as a reference for other occurrences of similar rocks. It was, in fact, mainly the fossil content that provided the means of correlating: if strata from two different places contain the same fossils, they are considered to be from the same period -- this is the basis of biostratigraphic correlation. **STRATIGRAPHIC UNITS** The International Stratigraphic Chart and the Geologic Time Scale that it shows provides an overall framework within which we can place all the rocks on Earth and the events that have taken place since the planet formed. It is, however, of only limited relevance when faced with the practical problems of determining the stratigraphic relationships between rocks in the field. Strata do not have labels on them which immediately tell us that they were deposited in a particular epoch or period, but they do contain information that allows us to establish an order of formation of units and for us to work out where they fit in the overall scheme. There are a number of different approaches that can be used, each based on different aspects of the rocks, and each of which is of some value individually, but are most profitably used in combinations. First, a body of rock can be distinguished and defined by its lithological characteristics and its stratigraphic position relative to other bodies of rock: these are **lithostratigraphic units** and they can readily be defined in layered sedimentary rocks. Second, a body of rock can be defined and characterized by its fossil content, and this would be considered to be a **biostratigraphic unit**. Third, where the age of the rock can be directly or indirectly determined, a **chronostratigraphic unit** can be defined: chronostratigraphic units have upper and lower boundaries that are each **isochronous surfaces**, that is, a surface that formed at one time. The fourth type of stratigraphic unit is a **magnetostratigraphic unit**, a body of rock which exhibits magnetic properties that are different to adjacent bodies of rock in the stratigraphic succession (21.4.3). Finally, bodies of rock can be defined by their position relative to unconformities or other correlatable surfaces: these are sometimes called **allostratigraphic units**, but this approach is now generally referred to as 'Sequence Stratigraphy' **LITHOSTRATIGRAPHY** In lithostratigraphy rock units are considered in terms of the lithological characteristics of the strata and their relative stratigraphic positions. The relative stratigraphic positions of rock units can be determined by considering geometric and physical relationships that indicate which beds are older and which ones are younger. The units can be classified into a hierarchical system of members, formations and groups that provide a basis for categorizing and describing rocks in lithostratigraphic terms. **Lithostratigraphic units** There is a hierarchical framework of terms used for lithostratigraphic units, and from largest to smallest these are: 'Supergroup', 'Group', 'Formation', 'Member' and 'Bed'. The basic unit of lithostratigraphic division of rocks is the formation, which is a body of material that can be identified by its lithological characteristics and by its stratigraphic position. It must be traceable laterally, that is, it must be mappable at the surface or in the subsurface. A formation should have some degree of lithological homogeneity and its defining characteristics may include mineralogical composition, texture, primary sedimentary structures and fossil content in addition to the lithological composition. Note that the material does not necessarily have to be lithified and that all the discussion of terminology and stratigraphic relationships applies equally to unconsolidated sediment. A formation is not defined in terms of its age either by isotopic dating or in terms of biostratigraphy. Information about the fossil content of a mapping unit is useful in the description of a formation but the detailed taxonomy of the fossils that may define the relative age in biostratigraphic terms does not form part of the definition of a lithostratigraphic unit. A formation may be, and often is, a **diachronous unit**, that is, a deposit with the same lithological properties that was formed at different times in different places. A formation may be divided into smaller units in order to provide more detail of the distribution of lithologies. The term **member** is used for rock units that have limited lateral extent and are consistently related to a particular formation (or, rarely, more than one formation). An example would be a formation composed mainly of sandstone but which included beds of conglomerate in some parts of the area of outcrop. A number of members may be defined within a formation (or none at all) and the formation does not have to be completely subdivided in this way: some parts of a formation may not have a member status. Individual beds or sets of beds may be named if they are very distinctive by virtue of their lithology or fossil content. These beds may have economic significance or be useful in correlation because of their easily recognizable characteristics across an area. Where two or more formations are found associated with each other and share certain characteristics they are considered to form a group. **Groups** are commonly bound by unconformities which can be traced basin-wide. Unconformities that can be identified as major divisions in the stratigraphy over the area of a continent are sometimes considered to be the bounding surfaces of associations of two or more groups known as a **supergroup.** **Description of lithostratigraphic units** The formation is the fundamental lithostratigraphic unit and it is usual to follow a certain procedure in geological literature when describing a formation to ensure that most of the following issues are considered. Members and groups are usually described in a similar way. **Lithology and characteristics** The field characteristics of the rock, for example, an oolitic grainstone, interbedded coarse siltstone and claystone, a basaltic lithic tuff, and so on form the first part of the description. Although a formation will normally consist mainly of one lithology, combinations of two or more lithologies will often constitute a formation as interbedded or interfingering units. Sedimentary structures (ripple cross-laminations, normal grading, etc.), petrography (often determined from thin-section analysis) and fossil content (both body and trace fossils) should also be noted. **Definition of top and base** These are the criteria that are used to distinguish beds of this unit from those of underlying and overlying units; this is most commonly a change in lithology from, say, calcareous mudstone to coral boundstone. Where the boundary is not a sharp change from one formation to another, but is gradational, an arbitrary boundary must be placed within the transition. As an example, if the lower formation consists of mainly mudstone with thin sandstone beds, and the upper is mainly sandstone with subordinate mudstone, the boundary may be placed at the point where sandstone first makes up more than 50% of beds. A common convention is for only the base of a unit to be defined at the type section: the top is taken as the defined position of the base of the overlying unit. This convention is used because at another location there may be beds at the top of the lower unit that are not present at the type locality: these can be simply added to the top without a need for redefining the formation boundaries. **Type section** **A type section** is the location where the lithological characteristics are clear and, if possible, where the lower and upper boundaries of the formation can be seen. Sometimes it is necessary for a type section to be composite within a **type area**, with different sections described from different parts of the area. The type section will normally be presented as a graphic sedimentary log and this will form the **stratotype**. It must be precisely located (grid reference and/or GPS location) to make it possible for any other geologist to visit the type section and see the boundaries and the lithological characteristics described. **Thickness and extent** The thickness is measured in the type section, but variations in the thickness seen at other localities are also noted. The limits of the geographical area over which the unit is recognized should also be determined. There are no formal upper or lower limits to thickness and extent of rock units defined as a formation (or a member or group). The variability of rock types within an area will be the main constraint on the number and thickness of lithostratigraphic units that can be described and defined. Quality and quantity of exposure also play a role; as finer subdivision is possible in areas of good exposure. **Biostratigraphy** A biostratigraphic unit is a body of rock defined by its fossil content. It is therefore fundamentally different from a lithostratigraphic unit that is defined by the lithological properties of the rock. The fundamental unit of biostratigraphy is the biozone. **Biozones** are units of stratigraphy that are defined by the zone fossils (usually species or subspecies) that they contain. In theory they are independent of lithology, although environmental factors often have to be taken into consideration in the definition and interpretation of biozones. In the same way that formations in lithostratigraphy must be defined from a type section, there must also be a type section designated as a stratotype and described for each biozone. They are named from the characteristic or common taxon (or occasionally taxa) that defines the **biozone**. There are several different ways in which biozones can be designated in terms of the zone fossils that they contain. **Interval biozones** these are defined by the occurrences within a succession of one or two taxa. Where the first appearance and the disappearance of a single taxon is used as the definition, this is referred to as a **taxon-range biozone**. A second type is a **concurrent range biozone**, which uses two taxa with overlapping ranges, with the base defined by the appearance of one taxon and the top by the disappearance of the second one. A third possibility is a **partial range biozone**, which is based on two taxa that do not have overlapping ranges: once again, the base is defined by the appearance of one taxon and the top by the disappearance of a second. Where a taxon can be recognized as having followed another and preceding a third as part of a phyletic lineage the biozone defined by this taxon is called a **lineage biozone** (also called a **consecutive range biozone**). **Assemblage biozones** in this case the biozone is defined by at least three different taxa that may or may not be related. The presence and absence, appearance and disappearance of these taxa are all used to define a stratigraphic interval. **Assemblage biozones** are used in instances where there are no suitable taxa to define interval biozones and they may represent shorter time periods than those based on one or two taxa. **Acme biozones** the abundance of a particular taxon may vary through time, in which case an interval containing a statistically high proportion of this taxon may be used to define a biozone. This approach can be unreliable because the relative abundance is due to local environmental factors. The ideal zone fossil would be an organism that lived in all depositional environments all over the world and was abundant; it would have easily preserved hard parts and would be part of an evolutionary lineage that frequently developed new, distinct species. Not surprisingly, no such fossil taxon has ever existed and the choice of fossils used in biostratigraphy has been determined by a number of factors that are considered in the following sections. **Rate of speciation** The frequency with which new species evolve and replace former species in the same lineage determines the resolution that can be applied in biostratigraphy. Some organisms seem to have hardly evolved at all: the brachiopod Lingula seems to look exactly the same today as the fossils found in Lower Palaeozoic rocks and hence is of little biostratigraphic value. The groups that appear to display the highest rates of speciation are vertebrates, with mammals, reptiles and fish developing new species every 1 to 3 million years on average (Stanley 1985). However, the stratigraphic record of vertebrates is poor compared with marine molluscs, which are much more abundant as fossils, but have slower average speciation rates (around 10 million years). There are some groups that appear to have developed new forms regularly and at frequent intervals: new species of ammonites appear to have evolved every million years or so during the Jurassic and Cretaceous and in parts of the Cambrian some trilobite lineages appear to have developed new species at intervals of about a million years (Stanley 1985). By using more than one species to define them, biozones can commonly be established for time periods of about a million years, with higher resolution possible in certain parts of the stratigraphic record, especially in younger strata. **Depositional environment controls** The conditions vary so much between different depositional environments that no single species, genus or family can be expected to live in all of them. The adaptations required to live in a desert compared with a swamp, or a sandy coastline compared with a deep ocean, demand that the organisms that live in these environments are different. There is a strong environmental control on the distribution of taxa today and it is reasonable to assume that the nature of the environment strongly influenced the distribution of fossil groups as well. Some environments are more favourable to the preservation of body fossils than others: for example, preservation potential is lower on a high-energy beach than in a low-energy lagoon. There is a fundamental problem with correlation between continental and marine environments because very few animals or plants are found in both settings. In the marine environment the most widespread organisms are those that are planktonic (free floating) or animals that are nektonic (free-swimming lifestyle). Those that live on the sea bed, the benthonic or benthic creatures and plants, are normally found only in a certain water depth range and are hence not quite so useful. The rates of sedimentation in different depositional environments are also a factor in the preservation and distribution of stratigraphically useful fossils. Slow sedimentation rates commonly result in poor preservation because the remains of organism are left exposed on the land surface or sea floor where they are subject to biogenic degradation. On the other hand, with a slower rate of accumulation in a setting where organic material has a higher chance of preservation (e.g. in an anoxic environment), the higher concentration of fossils resulting from the reduced sediment supply can make the collecting of biostratigraphically useful material easier. It is also more likely that a first or last appearance datum will be identifiable in a single outcrop section because if sediment accumulation rates are high, hundreds of metres of strata may lie within a single biozone. **Mobility of organisms** The lifestyle of an organism not only determines its distribution in depositional environments, it also affects the rate at which an organism migrates from one area to another. If a new species evolves in one geographical location its value as a zone fossil in a regional or worldwide sense will depend on how quickly it migrates to occupy ecological niches elsewhere. Again, planktonic and nektonic organisms tend to be most useful in biostratigraphy because they move around relatively quickly. Some benthic organisms have a larval stage that is free-swimming and may therefore be spread around oceans relatively quickly. Organisms that do not move much (a sessile lifestyle) generally make poor fossils for biostratigraphic purposes. **Geographical distribution of organisms** Two environments may be almost identical in terms of physical conditions but if they are on opposite sides of the world they may be inhabited by quite different sets of animals and plants. The contrasts are greatest n continental environments where geographical isolation of communities due to tectonic plate movements has resulted in quite different families and orders. The mammal fauna of Australia are a striking example of geographical isolation resulting in the evolution of a group of animals that are quite distinct from animals living in similar environments in Europe or Asia. This geographical isolation of groups of organisms is called provincialism and it also occurs in marine organisms, particularly benthic forms, which cannot easily travel across oceans. Present or past oceans have been sufficiently separate to develop localised communities even though the depositional environments may have been similar. This faunal provincialism makes it necessary to develop different biostratigraphic schemes in different parts of the world. Abundance and size of fossils To be useful as a zone fossil a species must be sufficiently abundant to be found readily in sedimentary rocks. It must be possible for the geologist to be able to find representatives of the appropriate taxon without having to spend an undue amount of time looking. There is also a play-off between size and abundance. In general, smaller organisms are more numerous and hence the fossils of small organisms tend to be the most abundant. The problem with very small fossils is that they may be difficult to find and identify. The need for biostratigraphic schemes to be applicable to subsurface data from boreholes has led to an increased use of microfossils, fossils that are too small to be recognized in hand specimen, but which may be abundant and readily identified under the microscope (or electron microscope in some cases). Schemes based on microfossils have been developed in parallel to macrofossil schemes. Although a scheme based on ammonites may work very well in the field, the chances of finding a whole ammonite in the core of a borehole are remote. Microfossils are the only viable material for use in biostratigraphy where drilling does not recover core but only brings up pieces of the lithologies in the drilling mud. **Preservation potential** It is impossible to determine how many species or individuals have lived on Earth through geological time because very few are ever preserved as fossils. The fossil record represents a very small fraction of the biological history of the planet for a variety of reasons. First, some organisms do not possess the hard parts that can survive burial in sediments: we therefore have no idea how many types of worm may have existed in the past. Sites where there is exceptional preservation of the soft parts of fossils (lagerstatten) provide tantalising clues to the diversity of lifeforms that we know next to nothing about (Whittington & Conway-Morris 1985; Clarkson 1993). Second, the depositional environment may not be favourable to the preservation of remains: only the most resistant pieces of bone survive in the dry, oxidizing setting of deserts and almost all other material is destroyed. All organisms are part of a food chain and this means that their bodies are normally consumed, either by a predator or a scavenger. Preservation is therefore the exception for most animals and plants. Finally, the stratigraphic record is very incomplete, with only a fraction of the environmental niches that have existed preserved in sedimentary rocks. The low preservation potential severely limits the material available for biostratigraphic purposes, restricting it to those taxa that had hard parts and existed in appropriate depositional environments. **TAXA USED IN BIOSTRATIGRAPHY** No single group of organisms fulfils all the criteria for the ideal zone fossil and a number of different groups of taxa have been used for defining biozones through the stratigraphic record (Clarkson 1993). Some, such as the graptolites in the Ordovician and Silurian, are used for worldwide correlation; others are restricted in use to certain facies in a particular succession, for example corals in the Carboniferous of northwest Europe. Some examples of taxonomic groups used in biostratigraphy are outlined below. **Marine macrofossils**: Brachiopods, Ammonoids, Gastropods, Corals and Echinoderms **Marine microfossils:** Foraminifera, Radiolaria, Calcareous nannofossils, other microfossil includes Ostracods, Diatoms, Conodonts, Acritarchs, and dinoflagellates. **Terrestrial fossil groups used in biostratigraphy:** palynomorphs includes pollen grains, seeds and spores.