Geometrical Optics PDF
Document Details
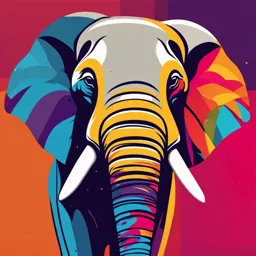
Uploaded by BreathtakingBinary924
European University Cyprus
Dr Irene Polycarpou
Tags
Summary
This document is a lecture or presentation on the topic of geometrical optics, providing an overview of key concepts such as the interaction of light with matter, the human eye, and various measurement techniques. It contains information about optical instruments.
Full Transcript
1 Optics Physics for Biomedical Sciences Dr Irene Polycarpou Microscopes revealed a micro-universe Optics, light & vision has been vital for human survival...
1 Optics Physics for Biomedical Sciences Dr Irene Polycarpou Microscopes revealed a micro-universe Optics, light & vision has been vital for human survival Cross section of a skeletal muscle Telescope observations forged our under the light understanding of the Universe microscope. Today, Optics remain a key scientific diagnostic technique (e.g. imaging) and may be also used during a surgery. Optics in Medicine Optics can provide improved illumination, magnification, and access to small or internal body cavities for visual examination and diagnosis of patients. Optics can exploit the interaction of light with biological cells, tissues, and organs for probing, imaging, or inducing changes for therapeutic purposes. Optics can enable novel devices, software, and methods that can characterize cancer tumors, track or guide therapies, or diagnose diseases using visible and near-infrared light. Corrective laser 3-D laser eye surgery imaging of cell The Human Eye The human eye can only detect frequencies in the visible spectrum. The quantity of light entering the eye is controlled by the size of the pupil, but it is the cells in the retina that detect the amount of light and the color. The retina consists of two types of light- sensitive (photoreceptor) cells called rods and cones. These cells react to the different light frequencies, producing different photochemical reactions that send electrical signals to the brain. The Human Eye Rods can distinguish between different intensities of light , but not between light of different frequencies. Cones allow us to see color. Photoreceptor cells Photometry Photometry is the science of measurement of light, in terms of its perceived brightness to the human eye. The human eye is not equally sensitive to all wavelengths of light. Photometry attempts to account for this by weighting the measured power at each wavelength with a factor that represents how sensitive the eye is at that wavelength. Photometry gives us information on: ✓ Luminous intensity ✓ Luminous flux ✓ Luminance ✓ Illuminance Luminous Intensity & Luminance Luminous intensity It is a measure of the power emitted by a light source in particular direction. The unit of measurement is candela (cd). Luminance: It is the luminous intensity emitted by a unit area of a light source. It is a measure of how bright the surface will appear to the eye from a particular angle. The units of luminance is the candela/m2 Luminous Flux or Luminous Power (Φ) It is energy per unit time (dQ/dt) that is radiated from a source over visible wavelengths. More specifically, it is energy radiated over wavelengths sensitive to the human eye, from about 330 nm to 780 nm. It is the measure of the perceived power of light. It differs from radiant flux, the measure of the total power of electromagnetic radiation, in that luminous flux is adjusted to reflect the varying sensitivity of the human eye to different wavelengths of light. Thus, luminous flux is a weighted average of the radiant flux in the visible spectrum. It is a weighted average because the human eye does not respond equally to all visible wavelengths. The SI unit of luminous flux is the lumen (lm). The lumen is defined in relation to the candela which is the unit of luminous intensity as: 1 lm = 1 cd ⋅ sr Illuminance (E) It is the total luminous flux of light incident per unit area. In other words, luminous flux represents the total amount of light emitted by the source, while illuminance refers to the total amount of light received by an object. The relationship between illuminance and luminous flux is similar to that between irradiance and radiation flux, that is, the radiation flux received per unit area. However, the illuminance is weighted according to the sensitivity of human eyes to light of different wavelengths, which represents the light intensity perceived by human eyes. The SI unit of illuminance is the lux (lx). It is equal to one lumen per square meter. 1 lx = 1 lm/m2 = 1 cd·sr/m2. Illuminance (E) Illuminance (E) or illumination is the total luminous flux falling on a unit area of a surface. It is a measure of how brightly a surface is lit. The unit of illuminance is lux (lx), which is the same as lumens per square meter (lm/m2) If 1 lumen (lm) of luminous flux uniformly lights a surface of area 1m2, the illuminance of the surface will be 1 lux. Lux vs. Lumen The lux takes into account the area over which the luminous flux is spread. For example, 100 lumens, concentrated into an area of one square meter, will light up that square meter with an illuminance of 100 lux. The same 100 lumens, spread out over a hundred square meters will produce a dimmer illuminance of only 1 lux. Candela, Lumen and Lux Candela measures the brightness of a light source in a specific direction → luminous intensity Lumens measures the total amount of light emitted by a light source in all directions → luminous flux Lux measures the amount of light that falls on a surface → illuminance Measurement of Light Effect of Distance on Luminous Intensity The distance from the light source has no influence on the amount of candela. On a distance of 1 meter, the amount of candela of a light source is equal to the candela on a distance of 2 meters Effect of Distance on Illuminance Illuminance obeys the inverse-square law, which states “… as a light source is moved away from a surface, the illuminance decreases by a factor that is inversely proportional to the square of the distance” Thus, the illuminance of a surface by a source of light 2m away, is ¼ of the illuminance at 1m from the source. Effect of Distance on Illuminance For two light sources, one at 1m from a surface and the other at 2 m, to give the same illuminance to the surface It would be necessary for the source at 2m to be 4 times as bright as the source at 1m. The Effect of Distance Lumen is independent of distance, as it measures the total light output from a source in all directions. The lumen value of a light source remains constant regardless of how far it is from an object or a surface. The Effect of Distance Lumen is independent of distance, as it measures the total light output from a source in all directions (luminous flux). Lux is inversely proportional to the square of distance, as it measures the amount of light that falls on a surface per unit area (illuminance). Candela is independent of distance, as it measures the light intensity in a specific direction or angle (luminous intensity). Fundamentals of Optics Reflection: Bouncing back of light from a surface or a boundary. Refraction: Bending of light as it passes from one medium to another with different optical density. Imaging: Formation of a representation of an object by light rays. Diffraction: Spreading of light as it encounters an obstacle or a slit. Interference: Superposition of two or more light waves resulting in a new wave pattern. Polarisation: Orientation of the electric field vector of a light wave in a specific direction. Reflection Real Image ✓ Image is made from “real” light rays that converge at a real focal point ✓ Can be projected onto a screen because it is formed by the actual convergence of light rays from an object ✓ Always inverted Virtual Image ✓ An image that is formed on a screen or a retina by a second lens or lens system ✓ The result of the further convergence of light rays from a real image ✓ Image only seems to be there! Virtual Images in Plane Mirrors A virtual image is an image that is formed in locations where light does not actually reach. It cannot be projected onto a screen, but it appears to the observer as though the light is coming from behind the mirror. A virtual image formed by a plane mirror is upright, same size and same shape as the object, and has an apparent left- right reversal. The image distance is equal to the object distance from the mirror. Reflection In order to reflect light waves with high efficiency, the surface of a mirror must be perfectly smooth over a long range, with imperfections that are much smaller than the wavelength of light being reflected. This requirement applies regardless of the shape of the mirror, which can be irregular or curved. There are two types of reflection: 1. Diffuse, where the angle of reflection is random, and does not have anything to do with the angle of incidence. 2. Specular, this is what you see in metals, mirrors, where the angle of reflection is equal to the angle of incidence, and, the relative angle of the photons is kept, that is how a mirror image is built. Curved Mirrors What if the mirror isn’t flat? Light still follows the same rules, with local surface normal. Parabolic mirrors have exact focus ✓Used in telescopes, backyard satellite dishes, etc. ✓Also forms virtual images. When parallel light rays hit a parabolic mirror, they all reflect to the same point — the focal point. Properties of a Mirror Focal length f is the distance from a lens or mirror to its image plane. It affects the image size and magnification. Focal point F is where light rays meet or seem to meet after passing through a lens or mirror. It is the image of an object at infinity. Radius of curvature R is the distance from a surface vertex to the center of curvature C, which is the center of the sphere that the surface is part of. It affects the light bending by the surface. Parabolic vs. Spherical Mirrors Parabolic mirrors are shaped to reflect all incoming parallel light rays to a single focal point, resulting in a sharp and focused image. Spherical mirrors have a constant curvature and reflect parallel light rays to a focal volume, causing spherical aberration and a blurry image. A sharp and focused image from a spherical mirror can only be obtained if it has a small surface. Therefore, spherical mirrors are often used for low-power or short-focal- length applications, while parabolic mirrors are preferred for high-power or long-focal-length applications. Parabolic Mirror When parallel light rays hit a parabolic mirror, they all reflect to the same point—the focal point. The location of this focal point depends on the curvature of the mirror. Spherical Mirror There are two types of spherical mirrors: concave and convex, depending on whether the reflecting surface is the inner or outer side of the sphere. The image formation by spherical mirrors depends on the size of the mirror relative to its radius of curvature. ✓ For small spherical mirrors, the image is formed by the intersection of the reflected rays. ✓ For large spherical mirrors, the image is formed by the intersection of the extended reflected rays. Types of Curved Mirrors Concave mirror ✓Reflective surface on the inner side of the sphere. ✓Magnify or reduce the size of the image, and invert or upright the image. Convex mirror ✓Reflective surface on the outer side of the sphere. ✓Has a negative focal length and a negative magnification, and it always forms the image behind the mirror. Concave vs. Convex Mirror Concave vs. Convex Mirror Inner side of the spoon ✓ Concave mirror ✓ See an inverted image of yourself that is larger than your actual size if you are close to the spoon, or a smaller and upright image if you are far from the spoon. Outer side of the spoon ✓ Convex mirror ✓ See a smaller and upright image of yourself that is always behind the spoon. This is because the concave mirror can focus the light rays reflected from your face, while the convex mirror can only diverge them. Object Position and Image Formation For a real object between f and the mirror, a virtual image is formed behind the mirror. The image is upright and larger than the object. Object Position and Image Formation For a real object between C and f, a real image is formed outside of C. The image is inverted and larger than the object. Object Position and Image Formation For a real object at C, the real image is formed at C. The image is inverted and the same size as the object. Object Position and Image Formation For a real object close to the mirror but outside of C, the real image is formed between C and f. The image is inverted and smaller than the object. Object Position and Image Formation What size image is formed if the real object is placed at the focal point f? For a real object at f, no image is formed. The reflected rays are parallel and never converge. Lateral Magnification Lateral magnification is the ratio of the image size to the object size measured in planes perpendicular to the optical axis. It indicates how much the image is magnified or reduced by an optical system. Can be positive or negative, depending on the orientation of the image and the object. ✓Positive magnification means the image and the object have the same orientation ✓Negative magnification means the image is inverted relative to the object Refraction Speed of light in vacuum: Light also goes through some things c = 2.9979x108 m/s (just use 3x108 m/s) ✓ Glass, water, eyeball, air The presence of material slows light’s progress ✓ Interactions with electrical properties of atoms Light rays change direction (are “refracted”) when they move from one medium to another. Refraction takes place because light travels with different speeds in different media. Refraction In medium, light moves at speed v, slower than in vacuum Index of refraction of a material is defined by c n = v Recall: wave speed 𝑣 = λ𝑓 Speed and wavelength change when light passes from one medium to another, frequency stays the same. c v= and n =. The “light slowing factor” is called the index of refraction. n n ❖ glass has n = 1.52, meaning that light travels about 1.5 times slower in glass than in vacuum ❖ water has n = 1.33 ❖ air has n = 1.00028 ❖ vacuum is n = 1.00000 (speed of light at full capacity) Refraction at Plane Surface Light bends at interface between refractive indices ✓bends more the larger the difference in refractive index A n1 = 1.0 n2 = 1.5 B Snell’s Law Snell's law, in optics, is a relationship between the path taken by a ray of light in crossing the boundary or surface of separation between two contacting substances and the refractive index of each. Snell’s Law governs the relationship between the angles of incidence and refraction and the indices of refraction of the two media. It applies to the refraction of light in any situation, regardless of type of media. Refraction When light travels from a faster medium (such as air) to a slower medium (such as glass), it bends towards the normal line because it slows down due to the higher optical density of the slower medium. Refraction and vision When we see in air, the light rays from the objects are refracted by the cornea and the lens of the eye to form a sharp image on the retina. When we see in water, the light rays from the objects are refracted less by the cornea and the lens of the eye because water has a higher optical density than air. This causes the image to be blurry or distorted on the retina, unless we wear goggles or contact lenses to correct the refraction. Refraction – Human Eye Refraction is the bending of light rays as they pass through different media, such as air and water. The eye uses refraction to focus light on the retina, where images are formed. Refraction – Human Eye Light rays from distance objects are parallel, so do not need much refraction to focus properly. Light rays from closer objects diverge, and need much refraction to focus properly. Reflection vs. Refraction Reflection is the bouncing back of light from a surface, while refraction is the bending of light as it passes from one medium to another. The angle of reflection is equal to the angle of incidence, while the angle of refraction depends on the ratio of the refractive indices of the two media. Lenses Lenses are transparent pieces of glass or plastic that bend light rays to form images. Spherical lenses: Lenses whose surfaces have spherical curvature. Each surface of a spherical lens can be convex (bulging outwards from the lens), concave (depressed into the lens), or planar (flat). Types of Lenses Lenses and Light Converging: rays meet at point Diverging: rays spread apart Collimated: rays parallel, no bending Positive or Converging Lens If the lens is biconvex, a collimated or parallel beam of light travelling parallel to the lens axis and passing through the lens will be converged (or focused) to a spot on the axis, at a certain distance behind the lens (known as the focal length). In this case the lens is called a positive or converging lens. Positive or Converging Lens If the lens is biconcave, a collimated or parallel beam of light travelling parallel to the lens axis and passing through the lens will be diverged (or spread). In this case the lens is called a negative or diverging lens. The beam after passing through the lens appears to be originating from a particular point on the axis in the front of the lens. The distance from this point to the lens is now as the focal length. Concave vs. Convex Lens Lens Maker’s Equation Lens Maker’s equation is a formula that relates the focal length of a lens to the refractive index of its material and the radii of curvature of its two surfaces. It can be used to design lenses with desired focal lengths and magnifications. Imaging Properties A positive or converging lens in air focuses a collimated beam travelling along the lens axis to a spot (known as the focal point) at a distance f from the lens. Conversely, a point source of light placed at the focal point is converted into a collimated beam by the lens. This leads to image formation in lenses. Lens formula Lens Formula Object distance Distance of object from optical center of lens. Denoted by u. Image distance Distance of image from optical center of the lens. Denoted by v. Focal length Distance of principal focus from the optical center of the lens. Denoted by f. Converging Lenses Object beyond 2F: image between F and 2F, real, inverted, diminished Object at 2F: image at 2F, real, inverted, same size Object between F and 2F: image beyond 2F, real, inverted, magnified Object at F: image at infinity, real, inverted, very large Object between F and O: image behind object, virtual, upright, magnified Diverging Lenses Object anywhere: image between F and O, virtual, upright, diminished. How Vision Works When you look at an object, light rays are reflected from the object to the cornea. The light rays are bent, refracted and focused by the cornea, lens and vitreous. The lens job is to make sure the rays come to a sharp focus on the retina. The resulting image on the retina is upside down. At the retina the light rays are converted to electrical impulses which are then transmitted through the optic nerve, to the brain, where the image is translated and perceived in an upright position. Eye as a Camera Diaphragm: this controls the amount of light rays entering. In the eye, it is the pupil while in the camera, it is the shutter. Lens: both the human eye and the camera have a lens that focus the light rays. However, the eyes has other structures that help in light refraction - cornea, aqueous humor, and vitreous humor. Image Sensor: for the eye, it is the rods and cones in the retina which converts electromagnetic energy to electrical and then sends the electrical impulses to the brain for interpretation through the optic nerve. In the camera, it's the film (at least in old cameras). How Vision Works https://www.youtube.com/watch? v=gBdyU1b0ADQ Optical Lenses and Devices Optical lenses and devices enable the detection, diagnosis and treatment of various medical conditions by using light and its interaction with living tissues. Optical lenses and devices can be used for various purposes, such as magnification, correction, image formation, illumination, spectroscopy, microscopy, endoscopy, laser delivery, and surgical instruments. Use of lenses in medicine: ✓As prosthetic for the correction of visual impairments such as myopia, hyperopia, presbyopia and astigmatism. ✓In imaging systems such as microscope. Comon Vision Defects Nearsightedness (myopia): eye too long, light focused in front of retina. Farsightedness (hyperopia): eye too short, light focused behind retina Astigmatism: cornea not spherical, light focused irregularly. Correction of Common Vision Defects Myopia: diverging lens, thinner in center, moves focal point back, aligns image with retina Hyperopia: converging lens, thicker in center, moves focal point forward, aligns image with retina Astigmatism: cylindrical lens, curved in one direction, corrects refractive error, creates uniform focus Myopia Myopia (nearsightedness) occurs when light rays are focused in front of the retina. Close objects can be seen clearly but objects at a distance are out of focus. Lenses are thinner in the center than on the edges (concave) and increase the focal length. The eye works by light being focused onto the retina by the cornea and lens. Hyperopia Hyperopia (farsightedness) is a condition in which light rays are focused behind the retina. Distant objects can be seen clearly, but close objects are out of focus. Lenses that are thicker in the center than on the edges (convex) decrease the focal length. Astigmatism Astigmatism is a condition in which the cornea or the lens has an irregular shape, causing light rays to focus unevenly on the retina. Objects at any distance can appear blurry or distorted, depending on the degree and direction of the astigmatism. Lenses that are curved in one direction (cylindrical) correct the refractive error, creating a uniform focus on the retina. Optical Fibers (Fiber Optics) Optical fibres are narrow tubes of glass fibres with a plastic coating that carry light from one end to the other. The light bounces off the walls of the fibre and can even bounce around corners. Light rays use total internal reflection to travel along the fibres. In order for this to be achieved, the light ray must hit the walls of the fibre at a minimum angle of 82°, which is the critical angle for light travelling from glass to plastic. Since the fibres are very narrow, this is usually not a problem. Optical Fibers Light travels through the core of a fiber optic by continually reflecting off of the cladding. Due to total internal reflection, the cladding does not absorb any of the light, allowing the light to travel over great distances. Some of the light signal will degrade over time due to impurities in the glass. There are two types of optical fibers: Single-mode fibers- transmit one signal per fiber (used in cable TV and telephones). Multi-mode fibers- transmit multiple signals per fiber (used in computer networks). The Use of Optical Fibers The properties of optical fibres make them useful for a wide range of applications including: ✓Medical - to transmit pictures of organs and arteries ✓Biological and genetics research ✓Industrial - to transmit pictures of the inside of complex machinery ✓Communications - to transmit data over long distances without transmission loss Today, Optics remain a key diagnostic technique (e.g. imaging). A new revolution in Optics has emerged with the birth of the laser, fibre optics, integration of optics and electronics, etc.. Endoscope An endoscope is a flexible tube with a light and camera that allows doctors to inspect and observe the inside of the body without major surgery. An endoscope can be used for various purposes, such as diagnosis, treatment, biopsy, or removal of polyps, stones, or foreign bodies in the digestive, respiratory, urinary, or reproductive systems. An endoscope works by using optics, electronics, and software engineering to transmit illumination and high-resolution images in real time from the target organ to a monitor. Endoscope Control section: the part that the doctor holds.. Insertion section: flexible tube that is inserted into the body of the patient. The lens or lens system is the optical component that captures the images of the internal organs and tissues. The light transmitting system is the component that provides illumination for the lens. The working channel is the hollow passage that allows the insertion of instruments, such as biopsy forceps, snares, or catheters, for diagnostic or therapeutic purposes. The distal end is the tip of the insertion section that contains the lens, the light source, and the opening of the working channel. Connector section: connects the endoscope to the external devices, such as the monitor, the light source, the insufflator, or the pump. Endoscope DISTAL END OF THE INSERTION SECTION Air/water nozzle: sprays air or water Illumination fibre optic: delivers light to lens Biopsy/suction channel: allows tissue sampling or suction Viewing fibre optics: transmits image to eyepiece Endoscope Endoscope Two of the main fibre optic features used in an endoscope are cables that light up (illuminate) the inside of the stomach. The other main feature is a fibre optic viewing cable that allows you to see what the problem is: These fibre optic cables rely on total internal reflection. Endoscope The endoscope gives visual evidence of the problem, and it can be used to collect a sample of tissue. An endoscope is a thin, flexible telescope. It is about as thick as a little finger. An endoscope can be passed through the mouth, into the oesophagus and down towards the stomach and duodenum. Or, it can be inserted into the rectum and through the colon. Endoscopy is a procedure used to examine internal organs. How does Endoscopy Work? 1. One of the two main endoscope cables carries light from a bright lamp in the operating room into the body, illuminating the cavity where the endoscope has been inserted. 2. The light bounces along the walls of the cable into the patient's body cavity. 3. The diseased or injured part of the patient's body is illuminated. 4. Light reflected off the body part travels back up a separate fiber-optic cable, bouncing off the glass walls as it goes. 5. The light shines into the physician's eyepiece so they can see what's happening inside the patient's body. Sometimes the fiber-optic cable is directed into a video camera (which displays what's happening on a television monitor) or a CCD (which can capture images like a digital camera or feed them into a computer for various kinds of image enhancement). Use of Endoscopes Arthroscopy The endoscope is inserted through an incision in the skin near a joint under investigation. This can be used to look at the joint and preform operations such as removing torn tissues. Bronchoscopy The endoscope is inserted through bronchial tubes within the lungs in order to look at the airway and to remove any objects blocking the airway. Endoscope Biopsy The endoscope is inserted through an incision or opening in the body that leads to the area under investigation. Biopsy forceps are then used to take a sample of tissue. Gastroscopy The endoscope is inserted down the throat to look for problems with the oesophagus, stomach and duodenum such as bleeding or ulcers. Laparoscopy The endoscope is inserted through an incision in the abdomen. How does Endoscopy Work? Bronchoscopy Bronchoscopy is a procedure that lets doctors observe patient’s lungs and air passages. During bronchoscopy, a thin tube (bronchoscope) is passed through the nose or mouth, down the throat and into the lungs. Bronchoscopy is most commonly performed using a flexible bronchoscope. However, in certain situations, such as a lot of bleeding in the lungs or a large object is stuck in the airway, a rigid bronchoscope may be needed. Endoscopic Ultrasound (EUS) In EUS procedure, the transducer is attached at the tip of the endoscope. Since the transducer is closer to the organ being inspected, it gives better and clearer images of the organs. Endoscopic ultrasound is used in evaluating cancer stages, pancreatitis, tumors, bowel leakage and more. Moreover, lymph nodes and blood vessels can also be examined along with the traditional digestive tract organs through the EUS procedure. Endoscopic Ultrasound (EUS) Ultrasound probe: emits and receives sound waves Needle: allows fine-needle aspiration or biopsy Camera: captures images of internal organs Microscope A microscope is an instrument for viewing objects that are too small to be seen by the naked or unaided eye. Classes of microscopes: Optical theory (optical microscopes AND electron microscopes): Function through the optical theory of lenses in order to magnify the image generated by the passage of a wave through the sample. The waves used are either electromagnetic in optical microscopes or electron beams in electron microscopes. Scanning probe Compound Electron vs. Optical Microscope Electron microscopes differ produce an image of a specimen by using a beam of electrons rather than a beam of light. Electrons have much a shorter wavelength than visible light, and this allows electron microscopes to produce higher-resolution images than standard light / optical microscopes. One limitation, however, is that electron microscopy samples must be placed under vacuum in electron microscopy. This means that live cells cannot be imaged. Electron vs. Optical Microscope Electron Microscope SCANNING ELECTRON MICROSCOPY (SEM) A beam of electrons moves back and forth across the surface of a cell or tissue, creating a detailed image of the 3D surface. Electron Microscope TRANSMISSION ELECTRON MICROSCOPY (TEM) The sample is cut into extremely thin slices (for instance, using a diamond cutting edge) before imaging, and the electron beam passes through the slice rather than skimming over its surface. TEM is often used to obtain detailed images of the internal structures of cells. SEM vs. TEM Transmission microscopy uses a beam of electrons that passes through a thin sample and forms an image on a detector. Scanning microscopy uses a beam of electrons that scans the surface of a sample and collects the scattered or emitted electrons. Compound Microscope A compound microscope uses a compound lens system (multiple lenses). Higher magnification is achieved by using two lenses rather than just a single magnifying lens. While the eyepieces and the objective lenses create high magnification, a condenser beneath the stage focuses the light directly into the sample. Types of Microscopes Electron vs. Optical Microscope Revision Revision Revision Revision Revision Revision Revision Revision