GEOL 312 - Geochemistry PDF
Document Details
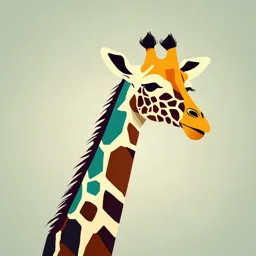
Uploaded by PropitiousCentaur7805
Swapan Kumar Haldar
Tags
Related
- Electromagnetic Acquisition & Conductivity PDF
- Metalliferous Ore Deposits Lecture Notes PDF
- RSG 504: Remote Sensing Applications in Mineral Exploration PDF
- Lecture 17 - General Procedures of Geochemical Exploration (Part 1) PDF
- Hindi PDF 1-31 dQÀfa¶fSXX, 2023
- Techniques d'exploration des substances utiles PDF
Summary
This document provides a detailed overview of mineral exploration principles and applications. It covers various methods, such as analytical techniques, and data interpretation approaches in relation to geochemical surveys.
Full Transcript
Mineral Exploration: Principles and Applications; By Swapan Kumar Haldar 1. DEFINITION................................................................................................................ 2 1.1 Elemental Dispersion...........................................................................
Mineral Exploration: Principles and Applications; By Swapan Kumar Haldar 1. DEFINITION................................................................................................................ 2 1.1 Elemental Dispersion....................................................................................................................... 2 1.2 Pathfinder Elements......................................................................................................................... 2 1.3 Background and Threshold Value................................................................................................... 3 1.4 Orientation Survey........................................................................................................................... 3 1.5. Regional, District and Local Scale Geochemistry.......................................................................... 5 2. FIELD PROCEDURE.................................................................................................. 5 3. ANALYTICAL METHODS........................................................................................ 6 3.1. Visual Colorimeter.......................................................................................................................... 6 3.2. Emission Spectrograph................................................................................................................... 6 3.3. Atomic Absorption Spectrometry................................................................................................... 6 3.4. X-ray Fluorescence......................................................................................................................... 7 3.5. Inductively Coupled Plasma-Atomic Emission Spectrometry....................................................... 7 3.6. Instrumental Neutron Activation Analysis..................................................................................... 7 3.7. Scanning/Transmission Electron Microscope................................................................................ 7 3.8. Electron Microprobe and Secondary Ion Mass Spectrometers....................................................... 8 3.9. Choice of Analysis.......................................................................................................................... 8 4. DATA INTERPRETATION....................................................................................... 8 5. GEOCHEMICAL METHODS................................................................................... 9 5.1 Pedo-Geochemical................................................................................................................... 10 5.2 Consolidated Weathered Cover..................................................................................................... 10 5.2.1. Calcrete.................................................................................................................................. 11 5.2.2 Silcrete.................................................................................................................................... 11 5.2.3 Laterite.................................................................................................................................... 11 5.2.4 Gossans................................................................................................................................... 11 5.3 Litho-geochemical Survey............................................................................................................. 14 5.4 Drift or Till Geochemical Survey.................................................................................................. 15 5.5 Stream Sediment Survey................................................................................................................ 16 5.6 Hydro-geochemical Survey........................................................................................................... 17 5.7 Vegetation Survey.......................................................................................................................... 17 5.7.1 Geobotany............................................................................................................................... 17 5.7.2 Biogeochemical...................................................................................................................... 18 5.8 Geo-zoological Survey.................................................................................................................. 19 5.9 Vapour Survey............................................................................................................................... 19 5.10 Electro-geochemical Survey........................................................................................................ 20 5.11 Radiogenic Isotope Geochemistry............................................................................................... 21 5.12 Heavy Mineral Survey................................................................................................................. 22 6. REVIEW......................................................................................................................23 1 GEOL 312 - GEOCHEMISTRY 1. DEFINITION Exploration geochemistry fundamentally deals with the enrichment or depletion of certain chemical elements in the vicinity of mineral deposits apart from barren regions. Geochemical prospecting is performed by systematic measurements of one or more chemical parameters, usually in traces, of naturally occurring material in the Earth’s crust. The samples are collected from rocks, debris, soil, gossan, stream or lake sediments, groundwater, vapour, vegetation and living things. The results of samples, at times, may show abnormal chemical pattern over the regional properties. This abnormality is defined as geochemical anomaly which may end up with discovery of near-surface or deep-seated hidden mineral deposits. 1.1 Elemental Dispersion Traces of metallic elements are usually found in soil, rocks, and groundwater in the proximity of an ore body. The fundamental dispersion pattern can be due to distribution of elements disseminated in rocks, which occurred during ore forming process. It can also be formed by migration and redistribution of elements in solution during oxidation, weathering and erosion of mineral deposit. The process usually occurs around the surface environment. The degree of concentration of specific elements logarithmically diminishes away from the deposit till it reaches to the background value of the enclosing rock. The geochemical envelope, which is an expression of alteration and zoning conditions surrounding metalliferous deposit, is called “primary dispersion halo.” The formation of primary halo is synchronous to the mineralization. It is essentially identical with geochemistry of unweathered rocks and minerals, irrespective to how and where the ore body itself was formed. The halos are either enriched or depleted in several elements as a result of introduction or redistribution related to ore- forming phenomena. The shape and size of the halo is exceptionally variable due to diverse mobility characteristics of the elements in solution and microstructures in the rocks. “Secondary dispersion halo” is the dispersed remnants of mineralization caused by surface processes of chemical and physical weathering and redistribution of primary patterns. The halo can be recognized in samples taken from the soil, rocks, sediments, groundwater and volatile matters at a distance of meters to tens of kilometres. Minerals are often unstable in secondary environment. Chemical weathering involves elemental breakdown of rocks and minerals in abundance of water, oxygen and carbon dioxide. It can move considerable distances from the source. Physical weathering implies disintegration of rocks and minerals with little or no chemical and mineralogical changes. It may often involve long distance transportation from the source. These minerals are chemically resistant. They are oxides (cassiterite, rutile, magnetite, chromite) and native form of gold, platinum, diamond, etc. The example of physical weathering in geochemical exploration is found in “till” and “glacial” deposits. 1.2 Pathfinder Elements “Pathfinder” or “indicator” elements are characteristic parameter in geochemical prospecting. These are relatively mobile elements due to physicochemical conditions of the solutions in which they are found or in volatile state (gaseous). They occur invariably in close geochemical association of the primary minerals being searched. These elements can be more easily found either because they form a broader halo around the deposit or because they can be detected more easily by simpler and less expensive analytical methods. Pathfinder elements play great role in locating concealed deposits due to these special properties. The choice of pathfinder necessitates that the elements used must occur in the 2 primary association with the element being sought, for example copper, nickel and chromium as pathfinders for deposits containing Platinum Group (Platinum, Palladium, Osmium, Iridium, Rhodium and Ruthenium) of Elements (PGE) (Bushveld chromium-PGE deposit, South Africa, Sudbury nickel- PGE deposit, Canada and Nausahi chromium PGE deposit, India), zinc for lead-silver-zinc deposits of Rajasthan and scheelite in Kolar gold field, Karnataka, India. The elements can also be derived from its parent by radioactive decay, such as the use of radon as a pathfinder for uranium deposits. Some common pathfinder elements are listed at Table 1. Table 1 Common Pathfinder Elements in Geochemical Exploration Type of deposits Pathfinder elements Gold, silver, gold-silver-copper-cobalt-zinc and complex sulphide ores As Copper-zinc-lead-silver and complex sulphide deposits Hg, Zn Wolframite-tin deposits Mo Porphyry copper, barium-silver deposits Mn, Mo, Au, Te Platinum-Palladium in mafic-ultramafic rocks Cu-Ni-Cr-Pd Uranium (all types) Rn, Cu, Bi, As Sulphide deposits SO4 1.3 Background and Threshold Value “Background” values are characterized by the normal range of concentration of elements in regional perspective rather than localized mineral occurrences. It is significant to establish the background value of the area against which the anomalies due to economic mineral accumulations, if any, can be identified. Large number of samples comprised of rock, soil, sediments, groundwater and volatile matters are analysed for multiple elements separately for each area before the exploration begins. Each type of sampled material should be treated separately. The values may vary significantly between samples. The frequency distribution is usually positively skewed. The arithmetic average (mean) is evidently biased by few scattered high value. The most frequently occurring value (mode) tends to lie within relatively restricted range and considered to be the normal abundance or background for that particular element of the area (Figure 1). “Threshold” value is defined as the probable upper or lower limit of the background value (Figure 1B) at some statistically precise confidence level. Any sample that exceeds this threshold is considered as possibly anomalous and belongs to a separate population. It may vary for each element, each rock type, different types of samples and in each area. The negative anomalous threshold defines the lower limit of background fluctuation. A geochemical section of a traverse-line is given in 1C. 1.4 Orientation Survey The success of exploration geochemistry depends on the appropriate detection of the pathfinder elements in primary and secondary environment. In practice, a first round “orientation survey” is conducted in every new area to draw detail work plan that adequately distinguish anomaly from background value. The important parameters to be considered in combination during orientation survey are: a) host rock environment, b) identify criteria that influence dispersion, c) possible local contamination if any, d) effect of topography, 3 e) best sample medium, f) optimum sample interval, g) depth of soil sample, h) size fraction, i) analysis of group of elements, j) anomaly enhancement and k) analytical techniques for establishing the background and threshold value. Figure 1: A typical illustration of (A) Histogram of Cu values showing the most frequently occurring samples, (B) geochemical profile of soil samples viewing background, threshold values and anomalous zone and (C) geological section of a concealed copper deposit. In the reconnaissance phase, regional geological knowledge, possible presence of economic mineral association and previous experience elsewhere will be a guiding factor to plan the orientation survey. The activities are focused around the probable targets with better knowledge of geochemical characteristics of the area for detailed exploration. The procedure should be simplified and finite. Orientation survey is always justified for any new area. It will optimize the sampling program and increase efficiency of interpretation with higher confidence. In turn, it saves considerable time and money in the long run. “Anomaly enhancement” techniques are commonly practiced during orientation surveys with weak anomalies, particularly for deep-seated mineralization. Value enhancement can be obtained by 4 physical, chemical and statistical means. Physical methods are enrichment of metallic, magnetic and heavy minerals by panning, magnetic and Chemical methods include selective leaching of iron and manganese oxides in the host environment. Statistical means are anomalous to background and trace elements ratio, additive and multiplicative halo concept. This technique highlights insignificant values of elements of interest in locating concealed mineral deposits. 1.5. Regional, District and Local Scale Geochemistry “Geochemical province” can be defined as a relatively large segment of area in which the chemical composition of bedrock is significantly different from the surrounding. It is manifested by certain suite of rocks relatively enriched in certain specific elements such as: Southern Australia and south-eastern Tanzania favourable for locating copper, chromium, nickel and platinum group of elements, Aravalli Mountain province for base metals, Chhattisgarh and Goa for iron ore, India. Similarly, “metallogenic province” represents large area characterized by an unusual abundance of particular type of metal in the country rocks such as: cop per producing area of Peru, Chile, Singhbhumand Khetri (India), lead-zinc- silver-producing area of Mt Isa (Australia) and Sullivan province (Canada), Zawar, Dariba, Rampura Agucha (India), tin at north-western Europe and Baster in India. There is no certain or unique sample interval. Traditionally low-density stream sediment surveys of one sample per 50 - 200 or 5 - 20 km2 will be adequate for selection of a province depending on the regional geological knowledge and terrain. The analysis is done for 16 - 25 elements. A “mineral district” is defined by the presence of known characteristic mineralization such as chromium mineralization in Jajpur-Keonjhar district, India. Stream sediment and limited soil and rock chip samples at 3 - 6 or even 1 - 2 km2 grid interval are followed during prospecting stage depending on the geological heterogeneity and signatures. The aim of “local-scale” geochemistry is to outline the location and broad extent of mineralization by detailed stream sediments sample at interval of 30 - 300 m. Rock and soil geochemistry can be exercised in the absence of inadequate drainage system. Once the target is identified more close spaced traverses at 100 - 300 m apart are sampled for soil and rocks at an interval 10 - 50 m across. The interpretation is further upgraded and precised by addition of pitting and deep trenching in close grid pattern. The target is now ready for drill testing. The mission and extent of geochemical exploration is generalized by progressively diminishing the size of search area in which an economic deposit may exist. The activity continues from grassroots reconnaissance to detailed sampling until a target is defined that can be tested by drilling. The sequential program demands increasingly more detail and expensive techniques with a sole objective of maximum probability of discovery at the lowest possible time and cost. 2. FIELD PROCEDURE The field procedure covers the collection of samples. It includes best sample material and optimum sampling design that identifies the presence of mineralization. The major sources of samples are from stream sediments, soils, rocks, groundwater and volatile matter. During field survey, 250 - 1000 g samples are collected by hand or with a plastic or aluminium scoop at specified interval from unconsolidated soil. Rock chips are collected by chisel and hammer. It is kept in plastic bags for soil and cloth bag for rocks with code numbers indicating project name, location, and sample type with description. The geological observations and sketches are recorded in field notebook. The grain size is preferred to be fines, say (-) 80 mesh in case of soil. The sample is reduced to 50 g by 5 drying, screening, grinding, cone and quartering at camp site; 5 g will be sufficient for laboratory, and about 1 g for analysis. The remaining sample part is preserved for future references. The quantity of sample to be collected from surface and groundwater varies between 100 and 1000 ml depending upon the number of elements and type of analysis. The water is collected in an exceptionally clean “hard” polyethylene sampling bottle as the elements are in the variation of parts per billion. 3. ANALYTICAL METHODS The methods for analyzing geochemical samples require utmost accuracy and precision, so as to detect background and related anomaly levels by rapid and inexpensive techniques. The analytical methods used in geochemical survey are visual colorimetry, emission spectrograph (ES), atomic absorption spectrometry (AAS), X-ray fluorescence (XRF), X-ray diffraction (XRD), radon atomic emission counter and radiometric analysis, mercury detector, inductively coupled plasma-atomic emission spectrometry (ICP-AES), instrumental neutron activation analysis (INAA), scanning/transmission electron microscope (SEM/TEM), electron microprobe and secondary ion mass spectrometers (SIMS). 3.1. Visual Colorimeter The “visual colorimeter (VC)” is a rapid and inexpensive method to reduce the bulk of samples collected during the field survey. It is primarily a semi-quantitative and hence less accurate technique to determine Pb, Zn, Cu, Ni, Co, Mo, As, and W, etc., from soil, bedrock and stream sediments. About 10 mg of sample at 80 - 100 mesh is decomposed by acid digestion and the digested mass is extracted with water or acid. The extract is masked by suitable masking agents for interfering radicals at suitably adjusting pH. An organic chelating agent (say, dithizone and furidioxime, etc.) is added to the solution to develop a coloured compound with one of the expected elements. The colour intensity of the solvent layer is visually compared with pre-calibrated solution. The lower level of detection (LLD) is 10 - 50 g/t. The reliability of the results can be accepted with practice. 3.2. Emission Spectrograph The “emission spectrograph (ES)” is also a rapid and low-cost semi-quantitative analytical technique capable of analysing broad spectrum of elements of widely varying composition with tolerable accuracy. About 10 - 15 mg of sample at (-) 80 mesh is thoroughly mixed with equal weight of pure graphite powder. The processed sample is completely burned between two carbon electrodes. The arc light passes through a dispersion medium with simultaneous recording of isolated radiation on a photographic plate. The sample plate is compared visually with standards to determine the qualitative presence of an expected element. The Intensity of blackening of plate emulsion is indicative of concentration of element present. The LLD is between 100 and 150 g/t. 3.3. Atomic Absorption Spectrometry The “atomic absorption spectrometry (AAS)” is the most suitable, rapid, precise and commonly used method for quantitative determination of large number of samples for multi-elemental analysis. About 50 mg of sample is treated with 5 ml of aqua regia and digested over hot plate. The solution is aspirated in to an air-acetylene or N2O-acetylene flame for complete atomization of test metals. Absorbance of a characteristic radiation of the desired metal is measured for computation of elemental concentration. About 30 elements can be determined from one solution, one after another, by AAS using specific hollow cathode lamps with background correction. 6 3.4. X-ray Fluorescence The “X-ray fluorescence (XRF)” technique uses high energy X-ray photons from an X-ray generation analyser to excite secondary fluorescence characteristic X-ray from the sample. The characteristic line spectra emitted by different elements of the sample are detected in the analyzer. The intensity of each line is proportional to the concentration of individual elements. 3.5. Inductively Coupled Plasma-Atomic Emission Spectrometry The “inductively coupled plasma-atomic emission spectrometry (ICP-AES)” works on optical emission method excited by inductively coupled plasma. It is a promising emission technique which has been successfully used as a powerful tool for fast multi-elemental analysis since 1975. The flame for this technique consists of incandescent plasma of argon heated inductively by radio-frequency energy at 4 - 50 MHz and 2 - 5 kW. The energy is transferred to a stream of argon through an induction coil, whereby temperature up to 10,000 oK is attained. The sample solutions are forced through capillary tube, nebulizer system and spray chamber to relatively cool central hole of the plasma torus. The spray chamber reduces the particle size of the aerosol to an ideal 10 mm. The sample atomizes and ionizes. The radiation from the plasma enters through a single slit and is then dispersed b -y a concave reflection grating. The light from each exit slit is directed to fall on the cathode of a photomultiplier tube, one for each spectral line isolated. The light falling on the photomultiplier gives an output which is integrated on a capacitor; the resulting voltages are proportional to the concentration of the elements in the sample. Multichannel instruments are capable of measuring the intensities of emission lines of up to 60 elements simultaneously. 3.6. Instrumental Neutron Activation Analysis The “instrumental neutron activation analysis (INAA)” technique utilizes the high-energy neutrons for irradiation of a sample to produce gamma radiation that can be analyzed for detection of elements. The method is suitable for detection of trace elements and Rare Earth Elements (REE), namely, Lanthanides, Scandium and Yttrium with high level of accuracy. 3.7. Scanning/Transmission Electron Microscope The detailed knowledge of physical nature of the surfaces of solids is of great importance in the field of geology, chemistry, and material science. Finer surface information at considerably higher resolution is obtained by scanning electron microprobe. In obtaining an image of “scanning electron microscope (SEM/TEM)” technique, the surface of a solid sample is swept in a raster pattern with a finely focused beam of electrons. The beam is swept across a surface in a straight line (the X-direction), then returned to its starting position and shifted downward (the Y-direction) by standard increment. The process is repeated until a desired area of the surface has been scanned. During the scanning process, a signal is received above the surface (the Z-direction) and stored in a computer system, where it is ultimately converted to an image. In case of “transmission electron microscope” the instrument is used either in biological application for ultra-high-resolution transmission electron photomicroscope studies of thin slice of cell and tissue material or in metallurgical studies, including, for example the investigation of defect structures in alloy material. Subject to some limitation, equivalent studies are carried out on geological samples. However, samples must be prepared as thin foils. As well as photomicrograph data, quantitative measurements may be derived in two ways, first crystal structure data may be derived from the pattern 7 resulting from electron diffraction within the sample. Second, compositional data may be obtained from characteristic X-ray emissions excited by the electron beam as it is transmitted through the foil. 3.8. Electron Microprobe and Secondary Ion Mass Spectrometers The “electron microprobe” provides a wealth of information about the physical and chemical nature of surfaces. With the electron microprobe method, X-ray emission is stimulated on the surface of the sample by a narrow focused beam of electrons, the resulting X-ray emission is detected and analyzed with either a wavelength or energy-dispersive spectrometer. “Secondary ion mass spectrometers (SIMS)” has proven useful for determining both the atomic and molecular composition of solid surface. SIMS is based upon bombarding the surface of the sample with a beam of 5 - 20 KeV ions such as Ar+, CS+, N2+, and O2+. The ion beam is formed in an ion gun, in which gaseous atoms or molecules are ionized by an electron impact source. The positive ions are then accelerated by applying a high Direct Current (DC) potential. The impact of these primary ions causes the surface layer of atoms of the sample to be tripped off, largely as neutral atom. A small fraction, however, forms as positive (or negative) secondary ions that are drawn into a spectrometer for mass analysis. 3.9. Choice of Analysis The choice of analytical technique for a particular element depends upon number of factors, not all related to analytical sensitivity. Such factors might include the stage of exploration, availability of specialized facilities, capital cost of instrumentation, speed and convenience to user for undertaking the analysis. The primary objective is to identify the techniques that are most widely used to determine individual elements and free of bias. The second aim is to examine the analytical potential of individual techniques. VC and ES are used for determination of selected major elements, including, SiO2, TiO2, Al2O3, Fe2O3 and P2O5. These techniques are usually advantageous during reconnaissance survey for availability of results at shortest time in the field. It can identify the presence of certain trace elements for target selection. A comparative analysis of inter-laboratory standard rock/mineral analysis indicated that XRF and AAS analysis are the most widely used and acceptable for determination of major elements as a group (except P2 O5, H2O). Since 1984, ICP-AES has become much better established technique for the analysis of trace elements including rare earth elements. There is a considerable overlap in the element coverage of ICP-AES and AAS, whereas the former is versatile and the latter is specific. XRF, AAS, and ICP-AES can determine a core of element including Ba, Co, Cr, Cu, Ni, Rb, Sr, Th, V and Zn. AAS is moderately expensive but precise and specific method for Co, Cr, Cu, Cd, Li, Ni, Rb, Sr, V and Zn at trace level. ICP-AES are costly and fast method. SEM, TEM and electron microprobes are excellent, efficient and accurate method for surface- and structure-related compositional analysis. However, the instruments are very costly and may not be within the reach of every one. 4. DATA INTERPRETATION The multi-elemental data from the geochemical survey can be analyzed by standard statistical methods to express the characteristics of each element. Analysis of frequency distribution, median, mode and mean of the sample population can identify and distinguish the background, threshold and anomalous value. Trend surface analysis and moving average method can produce the regional distribution pattern 8 of the elements being investigated. The isograde contour map will guide the detailed exploration program such as drill testing around the most anomalous zone (Figure 2). Correlation coefficient matrix of multi-element data will clearly show the maximum affinity between elements. Cluster analysis deals with mutually correlated elements. It exhibits the greatest within-group correlation relative to the between group, taking in to account all possible combinations of the given elements. Various other statistical methods can also be used. Figure 2: Data interpretation of soil and pit geochemical samples by contouring copper values to identify best target for drill testing. 5. GEOCHEMICAL METHODS Geochemical prospecting can broadly be classified into the following types depending on the stages of survey, nature of terrain, signal associated with the mineralization, type of analytical instrumentation available and finally time and cost permissible for the program: 1. Pedo-geochemical (soil survey), 2. Consolidated weathered cover prospecting, 3. Litho-geochemical (rock survey), 4. Drift or till geochemical survey, 5. Stream sediment survey, 6. Hydro-geochemical survey, 7. Vegetation survey (a) Geobotany, (b) Biogeochemical, 8. Geo-zoological/homo-geochemical survey, 9. Atmo-geochemical (vapour survey), 10. Electro-geochemical survey, 11. Radiogenic isotope geochemistry, 12. Heavy mineral survey, 13. Polymetallic-polynodule survey. 9 5.1 Pedo-Geochemical “Pedo-geochemical survey” is also known as “soil survey.” Soil is the unconsolidated weathering product. It generally lies on or close to its source of formation such as residual soil. It may be transported over large distances forming alluvial soil. Soil survey is widely used in geochemical exploration and often yields successful results. Anomalous enrichment of elements from underlying mineralization may occur due to secondary dispersion in the overlying soil, weathered product and groundwater during the process of weathering and leaching. The dispersion of elements spreads outward forming a larger exploration target than the actual size of the orebody. Soils display layering of individual characteristic horizons differing in mineralogy and trace element composition. Therefore, sampling of different horizons will present different results. The soil profile can be classified in three broad groups such as A, B and C horizons (Fig. 3). “A” horizon is composed of humus charged topsoil with minerals. “B” zone represents accumulation of clays enriched with trace elements. “C” horizon consists of bedrock fragments in various stages of degradation and gradually changes to hard parent rock. The metal content is generally higher in B horizon than in the A zone. The anomalous behaviour of C zone is similar to the parent bedrock. Therefore, samples from B layer enriched with trace elements are most preferred during soil survey. FIGURE 3 Diagrammatic presentation of soil horizons, relative vertical and lateral spread of dispersion and associated metal contents. Soil samples, from residual or transported material, play significant role during reconnaissance survey. It can provide a quick idea about presence or absence of target metals in the environment. Soil geochemistry as a successful exploration tool was demonstrated in discovery and development of the Kalkaroo Cu-Au-Mo deposit, South Australia, large PGE mineralization at Ural Mountain, Russia. Soil sampling had extensively been used for locating base metal deposits. 5.2 Consolidated Weathered Cover Weathering of cover sequence undergoes various types of chemical fractionation over millions of years due to paleoclimatic setup of the region. The consequential resistant residual weathering component of rocks and soils consolidates to form landscape geochemistry. The weathered cover can broadly be classified into four types depending on their composition, type of weathering and can guide in mineral search. 10 5.2.1. Calcrete The weathered crust in arid and semiarid region is represented by mixture of sand and silt cemented by calcite, dolomite, gypsum, halite, and ferric oxide simulated mainly by near-surface groundwater and vertical-lateral concentration of minerals like uranium, vanadium, potassium, calcium, magnesium, base and noble metals. Economic calcrete-type uranium deposits occur typically in Australia, Namibia, South Africa, Botswana, and China. Calcrete-type weathering indicates presence of carbonates nearby that may be metalliferrous as in case of Rajpura-Dariba base metal deposit in Rajasthan, India. This is also formed near ultramafic intrusions where carbonate nodules are common in soil along river banks like Subarnarekha, east of Singhbhum copper belt, Jharkhand, indicating presence of Cu-Ni-Co-PGE. 5.2.2 Silcrete This is a surface crust of residual weathering where sand and silt are cemented by silica. This is formed in the semiarid region in a stable groundwater condition. Silcrete is commonly found in association with gossan over the copper deposits of Khetri region, Rajasthan. 5.2.3 Laterite Laterite is a consolidated product of humid tropical weathering predominantly composed of goethite, hematite, kaolin, quartz, ± bauxite and other clay minerals. It is red, brown to chocolate coloured at the top showing hollow, vesicular, and botryoidal structure. It changes progressively from a nodular iron oxide-rich zone at the top to structureless clay-rich zone and ultimately merges with the partially altered to unaltered bedrocks. Laterite carry enriched grade of Fe, Al, Mn, Ni, Cu, Ti, and V. Lateritic cover can turn into low-grade iron, aluminum, nickel-copper and gold deposits with the increase of metal content. Geochemical studies of laterite have been successfully used in exploration for Ni-Cu and gold deposits in Western Australia and Ni deposit of Kansa at Sukinda chromite belt, Orissa, India. 5.2.4 Gossans “Gossans are signboards that point to what lies beneath the surface” Gossans are highly ferruginous rock which is the product of the oxidation by weathering and leaching of a sulphide body. The colour is significantly dependent on the mineralogical composition of the iron hydroxides and oxides phases and varies between reddish (hematite), yellow (jarosite), brown, black with stains of azure blue, malachite green and peacock colour (copper). Their texture can be brecciated, cleavaged, banded, diamond mesh, triangular, cellular, contour, sponge and colloform with box work of primary sulphides. When grains of sulphide are oxidized and residual limonite remains in the cavities, the texture assumes a honeycomb pattern, called box work of various colours that exist in the capping. The characteristic relic textures and colours resulting from the weathering of certain primary sulphide minerals, like sphalerite, galena, and chalcopyrite, will be specifically diagnostic. The identification can be corroborated by microscopic observation and chemical analysis. The field observations make it easy to detect gossan in prospecting areas with good outcropping conditions. The study of colour air photos and satellite images is of much use to focus on certain dark reddish bodies which have to be checked in the field. The depth of gossan may extend from few centimetres to hundreds of meters. The weathering of sphalerite above massive primary sulphide deposits usually depicts yellow-brown colour with coarse cellular box work and sponge structure (Fig. 4). The primary mineral sphalerite (ZnS) often changes to willemite (Zn2SiO4). The gossans of multi sulphide deposits are often associated with typical contour box work from silver-rich tetrahedrite (copper-stibnite) and tennantite (copper-arsenic) minerals. 11 FIGURE 4 The yellow-brown colour, cellular boxwork and sponge structure of unique gossans formation above Rajpura-Dariba zinc-lead-copper-silver deposit indicate presence of sphalerite underneath. The dark chocolate colour with cleavages, crust, radiate structures and cubic diamond mesh cellular boxwork (Fig. 5) in the gossan zone indicate the presence of galena and tetrahedrite as primary mineralization of the sulphide deposit at depth. The primary galena (PbS) often changes to anglesite (PbSO4), cerussite (PbCO3), pyromorphite (Pb5(PO4)3Cl) and mimetite (PbS(AsO4)3Cl). FIGURE 5 The dark chocolate color, crust radiate structure and diamond mesh cellular box work of unique gossans formation n above Rajpura-Dariba deposit indicate presence of galena and tetrahedrite underneath. The chalcopyrite often changes into native copper, melaconite (CuO), azurite (Cu3(CO3)2(OH)2) and malachite (CuCO3Cu(OH)3). The dark shades of peacock blue, green, black colour (Fig. 6) and triangular cellular structure is easily recognizable to expect primary copper sulphide at depth. Massive sulphide deposits contain usually large quantities of iron sulphides and carbonates (pyrite, pyrrhotite, marcasite, siderite and ankerite) which oxidize and produce an exceptional acidic environment above groundwater table. The acidic environment induces formation of characteristic gossan. Disseminated sulphides with less amounts of pyrite will produce a less acidic environment and gossan with a different geochemical signature. Geochemical studies of gossan have been successfully used in exploration of Ni-Cu at Malanjkhand Copper deposit, Madhya Pradesh, India. It shows complete alteration and enrichment profile (Fig. 7) to form a typical textbook gossan. The thin oxidized cap is represented by limonite with stains of malachite, azurite, and native copper. This is followed by zone of secondary sulphide enrichment in central and southern part of orebody with predominance of covellite, bornite, chalcocite and chalcopyrite. This is the most copper-enriched horizon of the deposit. The secondary enrichment grades into the primary orebody with gradational decrease in secondary minerals with predominance of chalcopyrite and pyrite. 12 FIGURE 6 The dark shades of multicolor peacock blue, green, brown and black, tiny boxwork of unique gossans formation above Rajpura-Dariba deposit indicate presence of primary chalcopyrite, tetrahedrite and tennantite ore deposit. Most significant examples can be cited from unique textbook type images as: World-class gossans formation at Rajpura-Dariba Pb-Zn-Cu-Ag deposit India, Zn-Pb-Ag deposit at Broken Hill (Australia), Ashanti Cu-Au deposit, Ghana, Zn mineralization in Togo (western Africa), Rouez gold deposit (France), Hassai Cu-Zn-Au deposit (Sudan), and Al Hazar Cu-Au deposit (Saudi Arabia), (refer Figs 8 and 9) and Khetri (refer Fig. 10). FIGURE 7 A generalized profile of gossan formation over massive copper sulfide deposits conceptualized after Malanjkhand copper deposit, India. All the facies of the gossan have to be sampled as they may correspond to different type of hosting mineralization. The weight of sample is about few kilograms scooped from the surface depending upon the homogeneity and chips collected in grid pattern for consolidated mass. Multielemental analysis in the reconnaissance stage is recommended. True gossan can be difficult to distinguish from ironstone and other iron oxide accumulation such as laterite. 13 FIGURE 8 Unique World-class gossans hill above the massive silver rich zinc-lead-copper deposit at Rajpura- Dariba. In 1977, the site dedicated to the Nation as “National Geological Monument” by Government of India. 5.3 Litho-geochemical Survey “Litho-geochemical surveys” (rock survey) are useful during regional work to recognize favourable geochemical province and favourable host rocks. Most of the epigenetic and syngenetic mineral deposits show primary dispersion around the mineralization by presence of abnormal high value of target elements. Litho-geochemistry aims at identifying that primary dispersion, other diagnostic geochemical features and trace element association which are different from barren rocks. Some granite possesses above-average contents of Mn, Mo, Au, and Te, indicating the potential of hosting porphyry copper deposits as in case of Malanjkhand, Madhya Pradesh. Other granite may contain high uranium. Rocks associated with tin deposits in Tasman geo-syncline content 3 - 10 times Sn value than barren rocks. Most of the SEDEX-type lead-zinc-silver deposits show primary pyrite halo. FIGURE 9 Part of the unique gossans collapsed in the eastern part of the hill during active underground mining operation leaving fresh exposure (Photo: Author, January 2010). The survey is based on analysis of unweathered whole rock samples or individual minerals. The sampling is carried out on a uniform grid across a geological terrain that includes several rock types from fresh outcrops, wall rocks and drill cores. Rock chip sample consists of five to six small broken fragments which individually or collectively represent a sample. Sample weighing around 1 kg will be 14 adequate for uniform fine- to medium-grained rocks. The weight increases with larger grain size. Primary halos in rocks play a great role in discovering deep-seated hidden deposits particularly having favourable structure. This is possible due to advanced analytical methods for correct assaying of very low value and knowledge of multielemental statistical techniques. FIGURE 10 Gossans are very common features as surface exploration guide at Khetri copper belt, India. The dimension is small compared to Rajpura-Dariba belt. The key guiding factors in litho-geochemical survey are: 1. Maximal concentration area with target elements around 10 times higher than background values. 2. Additive halo procedure by adding all the anomalous content of group of indicator elements. 3. Multiplicative halo concept by the ratio between product of all economic elements and product of impurity elements. 4. Vertical zoning of metal distribution. Linear productivity is the product of width of anomaly with % content of the element. 5. Anomaly ratio is the ratio of anomalous to the background value. 5.4 Drift or Till Geochemical Survey Drift prospecting is a broad term for sediments created, transported and deposited under the influence of moving ice of glaciers particularly in steep mountain terrain. The various sizes of rock fragments travel longer distances to form the drift sequences. The size and shape of the mineralized boulders along with stream sediments would reveal the extent of transportation and to trace back the source of the parent deposit a few kilometres away at higher elevation. The deposits are classified as glaciofluvial gravels and sands, silts and clays, and till or moraine. Till is a favoured sample medium for locating mineral deposits in glaciated terrain. The basal till sequence is studied for the presence of mineralized clasts, heavy minerals and relative abundance of major, minor and trace elements to assess the potentiality. The sample density, depth and method should be selected according to the needs of the exploration program. Concentration of oxidized ore minerals and their product of decomposition can be detected from the fine fractions in surface till sampling. Portable reverse circulation drills are used for collection of till samples at depth and to determine the vertical and lateral variation in till geochemistry. The survey also traces the detrital dispersal of the bedrock mineralization to the primary source. The activity components of till survey are collection of basal till sample, determination of ice flow history and data interpretation. Till geochemical exploration is extensively conducted in Canada (Thompson Ni-Cu-PGE and Bell copper deposit), North America (Eagle Bay Cu-Mo-Au-Ag deposit) and Glenlyon and Carmacks Cu- Zn-Pb-Ag-Au deposits of Yukon-Tanana terrain and Finland. Gorubathan massive multimetal deposit 15 of Himalaya at Darjeeling district, India, was discovered by the presence of float mineralized boulders in the downstream. The parent body was located few kilometres uphill. 5.5 Stream Sediment Survey Stream sediment sampling is most widely used in all reconnaissance and detailed survey of drainage basins. Many minerals, particularly the sulphide minerals, are unstable in weathering environment and will break down as a result of oxidation and other chemical reactions. The process will motivate secondary dispersion of both ore and indicator elements. The elements will move in solid and solution form to relative further distances within the drainage basin. The mobility of the different elements will vary significantly and eventually detrital fine-grained particles of rocks, minerals, clay, solutions, organic and inorganic colloids enriched in ore and indicator elements will be deposited downstream. The samples represent the best possible composite of weathered and primary rocks of the upstream catchments area. It is comprised of unconsolidated material in a state of mechanical transportation by streams, springs and creeks. Sample density of 1 in 200 km2 to close space following the course of the stream depends on the stages of exploration and results obtained. The optimum size fraction varies in different environment and generally - 80-mesh size is recommended. The samples are generally collected in dry season from natural sediment traps along stream courses below confluences or in three-point set around the confluence (Fig. 11). The choice of samples from first-, second- and third-order stream will depend on the terrain, climate and nature of weathering of the region. Two sets of samples are collected at each location. FIGURE 11 A schematic diagram showing (A) stream sediment sampling along the course of water channel and three-point sample collection around the confluence and (B) detailed pit or soil samples for drill testing (top inset). The first set is panned for heavy mineral concentration and the second one is the wet-sieved -200 mesh (-75 micron) or - 80 mesh fraction of stream sediments. The second set is allowed to settle for 10 min., decanted and transferred in high-quality plastic bag without any contamination, decanted again and air- dried. The discovery of large porphyry copper deposit of Bougainvillea, Papua New Guinea, gold deposits in British Columbia, lateritic nickel deposit of Sukinda belt, Orissa, and diamond-bearing kimberlite 16 deposit a6t Wajrakarur, Andhra Pradesh, India, by heavy mineral concentration are few examples of stream sediment survey. 5.6 Hydro-geochemical Survey There are two types of water sources, i.e. groundwater and surface water, having distinctly different chemical and physical properties. Groundwater occurs in dug wells, springs, and boreholes. It has a better potential in exploration geochemistry particularly if it is acidic (low pH) to dissolve and transport metal elements like Cu, Pb, Zn, Mo, Sn, S, U, Ni, and Co than surface water caused by chemical weathering and oxidation followed by leaching. Sample collection will be better if it is shallow enough for easy approach. Surface water from streams, river and oceans has less dissolving power and fine- grained sediments adsorb much of the metal carried by the water. The samples from stream water and sediments are collected simultaneously for analysis. Water samples are easy to collect. About a litre of water is collected and stored in a special quality container. Solubility of metals reduces with increase of pH from 4 to 7. Therefore, the pH is recorded at the time of sample collection. The suspended solids are filtered before analysis. The elemental value changes with time and season. It is desirable to analyze the sample before 48 h of collection. Sample cannot be preserved for future check studies. Hot springs are probable location for B, Li and Hg mineralization. Geochemical methods are also applied for search of mineral deposits under the sea such as manganese and phosphate nodules on the ocean floor. Water sample is becoming benchmark information on natural dispersion of toxic elements and for identifying pollution. 5.7 Vegetation Survey Vegetation survey can broadly be grouped in two types such as geobotany and biogeochemistry. Vegetation survey will receive prominence as an exploration guide in future as much of the world’s mineral resources are hidden beneath the vegetation. 5.7.1 Geobotany A plant will response to its geologic environment in which it grows and may show characteristic variations with respect to form, size, color, rate of growth and toxic effects. Geobotany uses these environmental variations. It involves visual survey of vegetation by recognition of a specific plant population and presence and absence of certain plant varieties associated with particular elements. “Alamine violet” thrives only on zinc-rich soils in the zinc district of Central and Western Europe. Viola calaminaria spp. acts as an indicator plant for base metal prospecting. Prolific growth of Impatiens balsamina and Nyctanthes arbortristis (Seuli) in the rainy seasons, exactly over the outcrops of lead-zinc deposits at Zawar (Fig. 12 and Fig. 13) and Leucas aspera in the ancient mine dump of Rajpura lead-zinc-silver deposit are location-specific indicator plants. Sometime normal growth of certain plant variety suffered from malformed or oddly coloured caused by excess presence of certain deleterious toxic trace elements on or near the mineralization. Dwarfing of plants and total absence of Sal (Shorea robusta) over the Kansa nickel deposit, Orissa, is significant. In contrast, it is abundant in rest of the valley. Bryophyte moss has been a good indicator of U mineralization in the Siwalik sandstone of Himachal Pradesh, India. 17 FIGURE 12 “Impatiens balsamina” or “Garden Balsam” or “Rose Balsam” (Balsaminaceae family) often grow over the outcrops of zinc-lead deposits and act as a natural geobotanical guide for mineral exploration as evidenced at, Zawar belt, India. FIGURE 13 Growth of “Nyctanthes arbortristis” or “Night Jasmine” or “Seuli” (Oleaceae family) often play as geobotanical guide for exploration of sulphide deposits, Zawar belt, India. 5.7.2 Biogeochemical Biogeochemistry involves the collection and chemical analysis of whole plants, selected parts and humus. During chemical weathering, mobilized elements dissolved and enrich in soils. As the plants and trees grow, these dissolved elements, including metals, from the soil are extracted by the roots of the trees which act as a sampling agent. The elements migrate to various parts of the tree such as roots, trunk, stem and finally to the leaves. When the leaves fall to the ground they enrich humus in the metal and the cycle becomes complete (Fig. 14). Anomalies indicating buried mineralization can be detected by judicial selection of appropriate parts of the plants (such as roots, bark, twigs, needles, leaves) and subject to analysis. Widely distributed plants of the same species, age and same parts should be sampled from point to point and compared for definition of anomaly. Samples should be washed thoroughly, cleaned and dried before analysis. The quantity should be large enough to generate adequate ash for trace element analysis. Artemisia (Sagebrush) accumulates high Cu in British Columbia and Pb, Zn, and Ba in Kazakhstan. Curatella americana L. is known to be potentially reliable indicator tree for epithermal gold quartz veins in Costa Rica. 18 FIGURE 14 Schematic illustration showing growth of plants by extraction of metallic elements from soil and migration from root acting as a sampling agent to the leaves. 5.8 Geo-zoological Survey Human and animals of certain territory suffer from specific diseases due to excess intake or deficiency of certain elements enriched in the surrounding rocks and soils. The common transfer routes are through drinking water, milk, and vegetables; cattle feed grass from the local area. People suffering from arsenosis, arthritis, fluorosis, sclerosis, and goiter indicate presence of anomalous trace elements of As, Cd, F, Hg and I deficiency, respectively in the surrounding area. The copper, zinc and lead contents of the trout livers have been used as pathfinder elements for mineralization. 5.9 Vapour Survey “Vapour surveys” (atmo-geochemical) helps in the location of buried deposits through detection of halos of mercury, helium, nitrogen, sulphur dioxide, hydrogen sulphide, hydrocarbon, radon, methane and other gases and volatile elements, often at a considerable distance from the source of mineralization. Vapours can be detected from air and soils and in groundwater. Volatile elements are released through oxidation of ore deposits. Common types of anomalies are as follows. Mercury vapour anomalies can be determined over structurally controlled mineralization in arid terrain. Hg anomalies are associated with concealed deep-seated high temperature geothermal system, lead- zinc-bearing sulphide assemblages, and over hydrocarbon gas and oil fields. Hg gas from the soil can be sampled (Fig. 15) by precipitation of Hg as an amalgam on extra pure noble metal foils (Ag) in couple of hours and analyzed. “He” anomalies are produced by radioactive decay and found over oil reservoirs, hot spring, porphyry copper and uranium deposits. Samples from shallow depth of soil are collected and analysed by mass spectrometry. Nitrogen anomalies are based on the reason that N2 concentrations increase toward the centre of hydrocarbon bearing basins. Methane (CH4), nitrogen, other natural gases and asphalt (a sticky black and highly viscous liquid or semi-liquid) are present in most crude petroleum basins and coal deposits. The bubbles of natural gases, crude oil and hot asphalt from the underlying oil and gas field escape 19 with high pressure to the Earth’s surface through fissures. The gas causes bubbles that make the asphalt appear to boil. The release of gas and asphalt to the surface acts as an excellent guide to locate and develop hydrocarbon (oil and gas) basin and coalfields. These features (Figs 16 and 17) can be seen today at Rancho La Brea Lake Pits in front of the Page Museum in the heart of the Los Angeles City. The site represents one of the world’s most famous fossil locations, trapped approximately between 110,000 and 10,000 years from now, above the renowned petroleum-producing basin of twentieth century at Los Angeles County. Asphalt and methane appear under surrounding buildings and require special operations for removal to prevent weakening building foundations. SO2, CO2 and CS2 are commonly found in soil over copper deposits and their wall rocks. FIGURE 15 Vapour sampling instrument (Credit: AK Talapatra, 2006). FIGURE 16 Oozing of hot methane and nitrogen gas bubbles from Rancho La Brea Tar Pits, Los Angeles County, one of the world’s most famous fossil locations. The pits are on the top of crude oil basin discovered in 1900 followed by exploration and production from 1907. 5.10 Electro-geochemical Survey Electro-geochemical survey, also known as CHIM (CHastichnoe Izvlechennye Metallov) survey in China, became well accepted during 1970s for landscape geochemical prospecting around arid to semi- arid region with deeply weathered terrain. The vertical dispersion of metal ions from the deep-seated 20 orebody to the surface by electrochemically mass transport through rock capillaries can be identified either in the soil or by vapour sampling. However, the ionic concentrations are too feeble to be detected by traditional geochemical methods. The electro-geochemical technique is capable of collecting larger volumes of mobilized metal ions on electrodes placed in the soil and applying small currents for a sustained period. The survey line set up over the expected hidden orebody is comprised of a series of specially coated carbon electrode pairs. The electrodes are placed at about 20 cm depth, about 60 cm apart and covered by soil. The electrodes are connected with 9 V DC battery and left for about 48 - 72 h. The electrode units are unearthed; the absorbent coatings scaled out and digested in concentrated nitric acid for elemental analysis by ICPMS. Soil samples are also collected from each electrode pit and analyzed by ICPMS for comparison. The survey scan lines are shifted on either side along the strike direction of expected mineralization at interval anywhere depending on stages of application. FIGURE 17 Oozing of hot crude oil and shiny black asphalt to the surface (foreground) over the abandoned petroleum basin in front of Page Museum, Rancho La Brea, in the heart of Los Angeles County. The background is the lass green grass park for public recreation around the Museum (Author, Srishti and Srishta, reconnaissance tour, July 2010). This technique is often recommended to validate targets indicated by geophysical conductivity, prominence of Hg concentration, nonpedogenic calcrete anomalies and shallow soil sampling before venturing for costly diamond drilling. It has successfully been tested for probing strike and depth extension for the concealed part of Challenger gold deposit in Australia and other deposits in United States, Canada and China. This method, together with Hg-soil geochemistry, is effective for selection of prospecting targets during reconnaissance for concealed ore beneath thick weathered overburden. The method has the advantage of being simple and rapid technique with high efficiency and reproducibility at relatively low costs. 5.11 Radiogenic Isotope Geochemistry The study of radiogenic isotopes geochemistry plays a significant role in modern-day scientific research for determination of chronology of rock-forming events. Isotope geochemistry is an attribute of the relative and absolute concentrations of the elements and their isotopes in the Earth. Variations in the abundance of these isotopes can be measured by “isotope ratio mass spectrometer.” The information can reveal the age of the rocks and minerals or the source of air and water. The study of isotope is divided into (a) stable isotope and (b) radiogenic isotope geochemistry. The most stable 21 isotopes are comprised of carbon (stable 12C, 13C and radioactive 14C), nitrogen (stable 14 N and 15 N), oxygen (stable 16O, 17O and 18O) and sulphur (stable 32S, 33S, 34S and 36S). The radiogenic isotope is comprised of lead-lead isotope geochemistry. Lead has four stable isotopes - 204 Pb, 206Pb, 207Pb, 208Pb and one common radioactive isotope 202Pb with a half-life of ~53,000 years. Lead is created in the Earth via decay of primarily uranium and thorium. The most important ratio pertains to daughter Pb isotope (206Pb and 207Pb) derived from the decay of radiogenic parent uranium (238U and 235U) and thorium isotopes (232Th). The other radiogenic ratios are among (Sm-Nd), Rb-Sr and K-Ar system. Samarium-neodymium isotope system can be utilized to provide a date or isotope signature or fingerprint of geological and archaeological finds (pots, ceramics). 147Sm decays to produce 143Nd with a half-life of 1.06 x 1011 years. 5.12 Heavy Mineral Survey Heavy minerals like ilmenite, sillimanite, garnet, zircon, rutile, monazite, magnetite, titanium, chromite, cassiterite, diamond, gold and platinum have a tendency to form onshore beach and offshore placer deposits. Prominent deposits occurring along coastline of countries like India (Fig. 18), Indonesia, Malaysia, and Australia bordering Indian Ocean are the largest marine resources of the world. The beaches in the east and west coast of India are enriched with on and offshore heavy minerals placer deposits and are exploited economically. The sampling is conducted by collecting regular interval vertical column of layered placer deposits. The isograde and isopach contours are drawn for computation of reserves. 5.13 Polymetallic-Polynodule Survey Polymetallic-polynodules, popularly known as manganese nodules, are rock concretions on the sea bottom formed by concentric layers of iron, manganese and other high value metals around a tiny core. The size of a fully developed nodule vary from fraction of millimeter to as large as 20 cm with an average size between 5 and 10 cm. Nodules are formed by precipitation of metals from sea water over several million years. Polymetallic nodules occur in most oceans of the world with greatest abundance at vast abyssal floor at depth between 4000 and 6000 m. The areas of economic interest have been identified at north central Pacific Ocean, the Peru basin in the southeast Pacific and the center of the north Indian Ocean. The most promising deposits with respect to resource and metal content occur between Hawaii Island and Central America in equatorial Pacific Ocean. The worldwide resource has been estimated at 5,00,000 million tonnes. The nodules are of greatest economic interest with metal contents varying between nickel (1.25 and 1.50%), copper (1.00 and 1.40%), cobalt (0.20 and 0.25%), manganese (~30%), iron (~6%), silicon (~5%), aluminium (~3%) and with lesser amounts of Ca, Na, Mg, K, Ti and Ba. Since 1970, research and development works initiated to identify the best ocean bed nodule deposits, establishing mining and process route by prospective mining consortia comprised of Federal and Private Companies from UK, United States, Germany, Belgium, Netherlands, Italy, Japan, France, Soviet Union, India and China. As a result, multi ton of nodules from the abyssal plains (~5500 m depth) of the eastern equatorial Pacific Ocean were collected. Significant quantities of Ni, Cu, and Co extracted from this ore using both pyro and hydro methods. However, the activities could not be commercialized due to excess availability of onshore nickel metal, anticipated ecological imbalance and finally conservation of the natural resources for the future. The research continues. 22 FIGURE 18 Heavy mineral placer deposits containing titanium, chromite, diamond, ilmenite, magnetite, monazite, rutile, zircon, garnet, and sillimanite along Indian coastline. 6. REVIEW Geochemistry plays vital role in mineral exploration long before conceptualization and continues much beyond the closure of mine operation. Baseline geochemical maps, at national and international levels, are generated by multielemental study of soil and rocks at interval on a scale of tens to thousands of kilometers covering the Earth’s surface. The samples are analyzed for total range of trace element (Ag, As, Au, Bi, Ca, Co, Cr, Cu, F, Fe, Hg, K, Mn, Mo, Na, Ni, Pb, Pd, Pt, Rn, Sb, Si, Te, Zn, etc.). The baseline maps, showing the regional spatial variation in chemical composition, are aimed at mineral exploration and broad-based environmental issues. An entire range of geochemical techniques have been focused with decision making criteria based on site conditions, judgment and previous experience during reconnaissance to closure of operations. Important parameters to consider when designing or evaluating a geochemical survey are: Orientation survey, Areal extent of the survey, Sampling density, Type of samples to collect (soil, bedrock, stream water, vegetation, gas, etc.), Post collection treatment of the samples (anomaly enhancement, sieving of soil samples into different particle size fractions), Choice of chemical analysis. Geochemical exploration eventually works on real-time modelling the ability to determine new sampling, drilling locations before moving drill rig for target testing, infill drilling, need to redrill and step-out decisions. It reduces lead times, which is especially important, when exploration season is short. 23