Geochemistry Lecture 1b PDF
Document Details
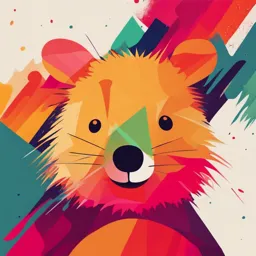
Uploaded by SweetheartSodium
Partido State University
Tags
Summary
This is a lecture on Geochemistry, covering topics such as early history, the role of geochemistry in the U.S.S.R., V.M. Goldschmidt, modern geochemistry, and environmental geochemistry. It also includes sections on the origin of the universe and cosmology.
Full Transcript
Lecture 1: Planet Earth in the Solar System What is Geochemistry? Outline I. Early History II. Geochemistry in the U.S.S.R. III. V.M. Goldschmidt IV. Modern Geochemistry 2 Modern Geochemistry Geochemical prospecting The use of chemica...
Lecture 1: Planet Earth in the Solar System What is Geochemistry? Outline I. Early History II. Geochemistry in the U.S.S.R. III. V.M. Goldschmidt IV. Modern Geochemistry 2 Modern Geochemistry Geochemical prospecting The use of chemical properties of naturally occurring substances (including rocks, glacial debris, soils, stream sediments, waters, vegetation and air) as aids in a search for economic deposits of metallic minerals or hydrocarbons. It continues to be one of the most important practical applications of geochemistry and now relies on a wide range of sophisticated analytical techniques. 3 Modern Geochemistry Environmental geochemistry has arisen recently because of the need to monitor the dispersion of metals and various organic compounds that are introduced into the environment as anthropogenic contaminants. This new application of geochemistry to the welfare of humankind is closely related to pollutant hydrogeology and medical geochemistry. Geochemical prospecting and environmental geochemistry are of paramount importance because they contribute to the continued well-being of the human species on Earth. 4 Modern Geochemistry Since about the middle of the 20th century geochemistry has become highly diversified into many subdivisions, among them Inorganic geochemistry – is the analysis of the composition of rocks, ores, minerals, water and soils adds a vital contribution to the understanding of geological mechanisms. Organic geochemistry - is simply the subdiscipline of geochemistry that focuses on organic (carbon bearing) compounds found in geologic environments Isotope geochemistry - is the study of the relative and absolute concentrations of the elements and their isotopes in samples from the Earth and solar system. Geochemical prospecting/ Geochemical exploration Medical geochemistry - is a sub-branch of medical geology, which focuses on the relationship of geochemical processes of elements with human and animal health. Aqueous geochemistry - studies the role of various elements in natural waters, including copper, sulfur, and mercury. Trace-element geochemistry Trace elements - those elements that occur in a mineral in such small amounts that they are not included in the mineral's chemical formula. Cosmochemistry - the study of the chemical composition of matter in the universe and the processes that led to those compositions. 5 Modern Geochemistry For our purposes the major goals of geochemists are: 1. To know the distribution of the chemical elements in the Earth and in the solar system. 2. To discover the causes for the observed chemical composition of terrestrial and extraterrestrial materials. 3. To study chemical reactions on the surface of the Earth, in its interior, and in the solar system around us. 4. To assemble this information into geochemical cycles and to learn how these cycles have operated in the geologic past and how they may be altered in the future. 6 Lecture 1: Planet Earth in the Solar System In the beginning Outline I. The Big Bang II. Stellar Evolution III. Nucleosynthesis 8 Origin of the Universe – Did it begin with a Big Bang? Most scientists think that the universe medium.com originated about 13.7 billion years ago in what is popularly called the Big Bang. Big Bang is a model for the evolution of the universe in which a dense, hot state was followed by expansion, cooling, and a less dense state. 9 Origin of the Universe – Did it begin with a Big Bang? Cosmology The study of the origin, evolution and nature of the universe. medium.com According to modern cosmology, the universe has no edge and therefore no center. Thus, when the universe began, all matter and energy were compressed into an infinitely small high-temperature and high- density state in which both time and space were set at zero. Therefore, there is no “before the Big Bang” but only what occurred after it. As demonstrated by Einstein’s theory of relativity, space and time are unalterably linked to form a space–time continuum, that is, without space, there can be no time. 10 Origin of the Universe – Did it begin with a Big Bang? Expanding universe How do we know that the Big Bang took place approximately 14 billion years ago? Why couldn’t the universe have always existed as we know it today? bbc.com Two fundamental phenomena indicate that the Big Bang occurred: Big Bang afterglow 1. The universe is expanding 2. It is permeated by background radiation. astronomy.com 11 Origin of the Universe – Did it begin with a Big Bang? Two fundamental phenomena indicate that the Big Bang occurred: 1. The universe is expanding When astronomers look beyond our own solar system, they observe that everywhere in the universe galaxies are moving away from each other at tremendous speeds. Scientificamerican.com 12 Origin of the Universe – Did it begin with a Big Bang? Britannica Two fundamental phenomena indicate that the Big Bang occurred: 1. The universe is expanding Edwin Hubble He first recognized this phenomenon in 1929. By measuring the optical spectra of distant galaxies, Hubble noted that the velocity at which a galaxy moves away Scientificamerican.com from Earth increases proportionally to its distance from Earth. 13 Origin of the Universe – Did it begin with a Big Bang? Britannica Two fundamental phenomena indicate that the Big Bang occurred: 1. The universe is expanding Edwin Hubble He observed that the spectral lines (wavelengths of light) of the galaxies are shifted toward the red end of the spectrum; that is, the lines are shifted Scientificamerican.com toward longer wavelengths. earthsky.com 14 Origin of the Universe – Did it begin with a Big Bang? Two fundamental phenomena Britannica indicate that the Big Bang occurred: 1. The universe is expanding Galaxies receding from each other at tremendous speeds would produce such a redshift. This is an example of the Doppler effect. Doppler effect Scientificamerican.com which is a change in the frequency of a sound, light, or other wave caused by movement of the wave’s source relative to the observer. 15 Origin of the Universe – Did it begin with a Big Bang? Britannica Two fundamental phenomena indicate that the Big Bang occurred: 1. The universe is expanding One way to envision how velocity increases with increasing distance is by reference to the popular analogy of a rising loaf of raisin bread in which the raisins are uniformly distributed throughout the loaf. Scientificamerican.com 16 Origin of the Universe – Did it begin with a Big Bang? Britannica Two fundamental phenomena indicate that the Big Bang occurred: 1. The universe is expanding As the dough rises, the raisins are uniformly pushed away from each other at velocities directly proportional to the distance between any two raisins. The farther away a given raisin is to begin with, the farther it must Scientificamerican.com move to maintain the regular spacing during the expansion, and hence the greater its velocity must be. 17 Origin of the Universe – Did it begin with a Big Bang? Britannica Two fundamental phenomena indicate that the Big Bang occurred: 1. The universe is expanding In the same way that raisins move apart in a rising loaf of bread, galaxies are receding from each other at a rate proportional to the distance between them, which is exactly what Scientificamerican.com astronomers see when they observe the universe. 18 Origin of the Universe – Did it begin with a Big Bang? Britannica Two fundamental phenomena indicate that the Big Bang occurred: 1. The universe is expanding By measuring this expansion rate, astronomers can calculate how long ago the galaxies were all together at a single point, which turns out to be about 14 billion years, the currently Scientificamerican.com accepted age of the universe. 19 Origin of the Universe – Did it begin with a Big Bang? Two fundamental phenomena indicate that the newscientist.com Britannica Big Bang occurred: 2. It is permeated by a background radiation Arno Penzias and Robert Wilson of Bell Telephone Laboratories They made the second important observation that provided evidence of the Big Bang in 1965. They discovered that there is a pervasive background radiation of 2.7 Kelvin (K) above Big Bang afterglow absolute zero (absolute zero equals –273 degree C; 2.7 K = –270.3 degree C) everywhere in the Scientificamerican.com universe. This background radiation is thought to be the fading afterglow of the Big Bang. astronomy.com 20 Origin of the Universe – Did it begin with a Big Bang? Currently, cosmologists cannot say what it was like at time zero of the Big Bang because they do not understand the physics of matter and energy under such extreme conditions. However, it is thought that during the first second following the Big Bang, the four basic forces: gravity (the attraction of one body toward another), electromagnetic force (the combination of electricity and magnetism into one force and binds atoms into molecules), strong nuclear force (the binding of protons and neutrons together), weak nuclear force (the force responsible for the breakdown of an atom’s nucleus, producing radioactive decay) separated, and the universe experienced enormous cosmic inflation. 21 Origin of the Universe – Did it begin with a Big Bang? By the end of the first three minutes following the Big Bang: the universe was cool enough that almost all nuclear reactions had ceased, and by the time it was 30 minutes old, nuclear reactions had completely ended and the universe’s mass consisted of almost entirely of hydrogen and helium nuclei. 22 Origin of the Universe – Did it begin with a Big Bang? As the universe continued expanding and cooling, stars and galaxies began to form and the chemical makeup of the universe changed. Initially, the universe was 76% hydrogen and 24% helium Whereas today it is 70% hydrogen, 28% helium, and 2% all other elements by weight. 23 Origin of the Universe – Did it begin with a Big Bang? How did such a change in the universe’s composition occur? Throughout their life cycle, stars undergo many nuclear reactions in which lighter elements are converted into heavier elements by nuclear fusion. When a star dies, often explosively, the heavier elements that were formed in its core are returned to interstellar space and are available for inclusion in new stars. In this way, the composition of the universe is gradually enhanced in heavier elements. 24 Origin of the Universe – Did it begin with a Big Bang? In fact, it is estimated that when the universe is one trillion years old, it will consist of 20% hydrogen, 60% helium, and 20% all other elements. chem.libretexts.org 25 Stellar evolution Matter in the universe is organized into a “hierarchy of heavenly bodies” listed below in order of decreasing size. clusters of galaxies comets galaxies stars, pulsars, and black holes planets satellites asteroids meteoroids dust particles molecules atoms of H and He 26 Stellar evolution On a subatomic scale, space between stars and galaxies is filled with cosmic rays (energetic nuclear particles) and photons (light). Cosmic rays are a form of high-energy radiation that originate from outside our solar system. When they reach Earth, the rays collide with particles in the upper atmosphere to produce a “shower” of particles, including muons. iaea.org Photons are elementary particles with no charge, no resting mass, and travel at the speed of light. This article will acquaint us with photons and their properties. Stars are the basic units in the hierarchy of heavenly bodies within which matter continues to evolve by nuclear reactions. 27 Stellar evolution Many billions of stars are grouped together to form a galaxy, and large numbers of such galaxies are associated into galactic clusters. Stars may have stellar companions or they iaea.org may have orbiting planets, including ghostly comets that flare briefly when they approach the star on their eccentric orbits. wikipedia 28 Stellar evolution The planets in our solar system have their own retenue of satellites. The space between Mars and Jupiter contains the asteroids, most of which are fragments of larger bodies that have been broken up by collisions and by the gravitational forces of Jupiter and Mars. iaea.org Main Asteroid Belt The majority of known asteroids orbit within the asteroid belt between Mars and Jupiter, generally with not very elongated orbits. The belt is estimated to contain between 1.1 and 1.9 million asteroids larger than 1 kilometer wikipedia (0.6 miles) in diameter, and millions of smaller ones. Persson and Biele, 2022 29 Stellar evolution Trojans These asteroids share an orbit with a larger planet, but do not collide with it because they gather around two special places in the orbit (called the L4 and L5 Lagrangian points). The Jupiter trojans form the most significant population of trojan asteroids. It is thought that they are as numerous as the asteroids in the asteroid belt. There are Mars and Neptune iaea.org trojans, and NASA announced the discovery of an Earth trojan in 2011. Pieces of the asteroids have impacted as meteorites on the surfaces of the planets and their satellites and have left a record of these events in wikipedia craters. Persson and Biele, 2022 30 Stellar evolution On an even smaller scale, space between stars contains clouds of gas and solid particles. The gas is composed primarily of hydrogen and of helium that were produced during the initial expansion of the universe. In addition, the interstellar medium contains elements of higher atomic number that were synthesized by nuclear reactions in the interiors of stars that have since exploded. iaea.org A third component consists of compounds of hydrogen and carbon that are the precursors of life. These clouds of gas and dust may contract to form new stars whose evolution depends newscientist.com on their masses and on the H/He ratio of the gas cloud from which they formed. wikipedia myearthscience.com 31 Stellar evolution Types of stars: Main sequence stars fuse hydrogen to helium in its core make up around 90% of the universe’s stellar population They range in luminosity, color, and size – from a tenth to 200 times the Sun’s mass – and live for millions to billions of years. Examples: Sirius – brightest star in the night sky; found in the Canis Major constellation Rigil Kentaurus (Alpha Centauri) – closest main sequence star that can be seen by the naked eye; found in the southern constellation Centaurus science.nasa.gov 32 Stellar evolution Types of stars: Red giants When a main sequence star less than eight times the Sun’s mass runs out of hydrogen in its core, it starts to collapse because the energy produced by fusion is the only force fighting gravity’s tendency to pull matter together. But squeezing the core also increases its temperature and pressure, so much so that its helium starts to fuse into carbon, which also releases energy. Hydrogen fusion begins moving into the star’s outer layers, causing them to expand. The result is a red giant, which would appear more orange than red. science.nasa.gov astroweb.case.edu 33 Stellar evolution Types of stars: Red giants Eventually, the red giant becomes unstable and begins pulsating, periodically expanding and ejecting some of its atmosphere. Eventually, all of its outer layers blow away, creating an expanding cloud of dust and gas called a planetary nebula. The Sun will become a red giant in about 5 billion years. Examples: Arcturus – red giant in the northern constellation Boötes and Gamma Crucis in the southern constellation Crux (the Southern Cross Betelgeuse – a red supergiant star in science.nasa.gov the constellation of Orion. astroweb.case.edu 34 Stellar evolution Types of stars: White dwarfs After a red giant has shed all its atmosphere, only the core remains. A white dwarf is usually Earth-size but hundreds of thousands of times more massive. A teaspoon of its material would weigh more than a pickup truck. A white dwarf produces no new heat of its own, so it gradually cools over billions of years. Despite the name, white dwarfs can emit visible light that ranges from blue white to red. science.nasa.gov 35 Stellar evolution Types of stars: White dwarfs Scientists sometimes find that white dwarfs are surrounded by dusty disks of material, debris, and even planets – leftovers from the original star’s red giant phase. In about 10 billion years, after its time as a red giant, the Sun will become a white dwarf. White dwarfs are too dim to see with the unaided eye, although some can be found in binary systems with an easily seen main sequence star. Example: Procyon B – found in the northern constellation Canis Minor science.nasa.gov 36 Stellar evolution Types of stars: Neutron stars Neutron stars are stellar remnants that pack more mass than the Sun into a sphere about as wide as New York City’s Manhattan Island is long. A neutron star forms when a main sequence star with between about eight and 20 times the Sun’s mass runs out of hydrogen in its core. (Heavier stars produce stellar-mass black holes.) The star starts fusing helium to carbon, like lower- mass stars. But then, when the core runs out of helium, it shrinks, heats up, and starts converting its carbon into neon, which releases energy. This process continues as the star converts neon into oxygen, oxygen into silicon, and finally silicon into iron. science.nasa.gov 37 Stellar evolution Types of stars: Neutron stars These processes produce energy that keep the core from collapsing, but each new fuel buys it less and less time. By the time silicon fuses into iron, the star runs out of fuel in a matter of days. The next step would be fusing iron into some heavier element, but doing so requires energy instead of releasing it. The core collapses and then rebounds back to its original size, creating a shock wave that travels through the star’s outer layers. The result is a huge explosion called a supernova. The remnant core is a superdense neutron science.nasa.gov star. 38 Stellar evolution Types of stars: Neutron stars Pulsars These are a type of rapidly rotating neutron star. Bright X-ray hot spots form on the surfaces of these objects. As they rotate, the spots spin in and out of view like the beams of a lighthouse. Some pulsars spin faster than blender blades. Magnetars All neutron stars have strong magnetic fields. But a magnetar’s can be 10 trillion times stronger than a refrigerator magnet’s and up to a thousand times stronger than a typical neutron star’s. Neutron stars are too faint to see with the unaided eye or backyard telescopes, although the Hubble Space Telescope has been able to capture a few in visible light. Astronomers usually observe them via X-rays and radio emission. science.nasa.gov 39 Stellar evolution The evolution of stars can be described by specifying their luminosities and surface temperatures. The luminosity of a star is proportional to its mass, and its surface temperature or color is an indicator of its volume. Luminosity (L) is a measure of the total amount of energy radiated by a star or other celestial object per second. This is therefore the power output of a star. When a cloud of interstellar gas contracts, its temperature increases, and it begins to radiate energy in the infrared and visible parts of the spectrum. newscientist.com As the temperature in the core of the gas cloud approaches wikipedia 20x106 K, energy production by hydrogen fusion becomes possible, and a star is born. 40 Stellar evolution Most of the stars of a typical galaxy derive energy from this process and therefore plot in a band, called the main sequence, on the Hertzsprung-Russell diagram shown in Figure 2.1. Massive stars, called blue giants, have high luminosities and high surface temperatures. The Sun is a star of intermediate mass and has a surface temperature of about 5800 K. iaea.org Stars that are less massive than the Sun are called red dwarfs and plot at the lower end of the main sequence. newscientist.com 41 Stellar evolution As a star five times more massive than the Sun converts hydrogen to helium while on the main sequence, the density of the core increases, causing the interior of the star to contract. 3 steps to form from H to He In the first stage two protons combine and one of them converts into a neutron to form a nucleus of the heavy isotope of hydrogen known as deuterium. Next, the deuterium nucleus combines with another proton to form the light helium isotope known as helium-3. Finally, two helium-3 nuclei combine to form helium-4, releasing two protons. iaea.org euro-fusion.org esa.int 42 Stellar evolution The core temperature therefore rises slowly during the hydrogen-burning phase. This higher temperature accelerates the fusion reaction and causes the outer envelope of the star to expand. However, when the core becomes depleted in hydrogen, the rate of energy production declines and the star contracts, raising the core temperature still further. iaea.org The site of energy production now shifts from the core to the surrounding shell. newscientist.com The resulting changes in luminosity and surface temperature cause the star to move off the main sequence toward the realm of the red giants (Figure 2.1). 43 Stellar evolution The helium produced by hydrogen fusion in the shell accumulates in the core, which continues to contract and therefore gets still hotter. The resulting expansion of the envelope lowers the surface temperature and causes the color to turn red. At the same time, the shell in which hydrogen is reacting gradually thins as it moves toward the surface, and the luminosity of the star declines. These changes transform a main-sequence star into a bloated red giant. newscientist.com For example, the radius of a star five times more massive than the Sun increases about 30-fold just before helium burning in the core begins. 44 Stellar evolution When the core temperature approaches 100 x 106 K, helium fusion by means of the “triple alpha process” begins and converts three helium nuclei into the nucleus of carbon-12. At the same time, hydrogen fusion in the shell around the core continues. The luminosities and surface temperatures (color) of red giants become increasingly variable as they evolve, reflecting changes in the rates of energy production in the core and shell. newscientist.com 45 Stellar evolution The evolutionary tracks in Figure 2.1 illustrate the importance of the mass of a star to its evolution. A star five times as massive as the Sun is 1000 times brighter while on the main sequence and has a more eventful life as a red giant than stars below about two solar masses (Iben, 1967,1974). newscientist.com 46 Stellar evolution The length of time a star spends on the main sequence depends primarily on its mass and to a lesser extent on the H /He ratio of its ancestral gas cloud. In general, massive stars (blue giants) consume their fuel rapidly and may spend only 10 x 106 years on the main sequence. Small stars (red dwarfs) have much slower “metabolic” rates and remain on the main sequence for very long periods of time exceeding 10 x 109 years. newscientist.com 47 Stellar evolution The Sun, being a star of modest magnitude, has enough hydrogen in its core to last about 9 x 109 years at the present rate of consumption. Since it formed about 4.5 x 109 years ago, the Sun has achieved middle age and will provide energy to the planets of the solar system for a very long time to come. However, ultimately its luminosity will increase, and it will expand to become a red giant, as shown in Figure 2.1. newscientist.com 48 Stellar evolution The temperature on the surface of the Earth will then rise and become intolerable to life forms! The expansion of the Sun may engulf the terrestrial planets, including Earth, and vaporize them. When all of its nuclear fuel has been consumed, the Sun will assume the end stage of stellar evolution that is appropriate for a star of its mass and chemical composition. newscientist.com 49 Stellar evolution Toward the end of the giant stage, stars become increasingly unstable. When the fuel for a particular energy-producing reaction is exhausted, the star contracts and its internal temperature rises. The increase in temperature may trigger a new set of nuclear reactions. In stars of sufficient mass, this activity culminates in a gigantic explosion (supernova) as a result of which a large fraction of the outer envelope of the star is blown away. newscientist.com The debris from such explosions mixes with hydrogen and helium in interstellar space to form clouds of gas and dust from which new stars may form. 50 Stellar evolution As stars reach the end of their evolution they turn into white dwarfs, or neutron stars (pulsars), or black holes, depending on their masses (Wheeler, 1973). Stars whose mass is less than about 1.2 solar masses contract until their radius is only about 1 x 104 km and their density is between 104 and 108 g/cm3. Stars in this configuration have low luminosities but high surface temperatures and are therefore called white dwarfs (Figure 2.1). newscientist.com They gradually cool and fade from view as their luminosities and surface temperatures diminish with time. 51 Stellar evolution Stars that are appreciably more massive than the Sun develop dense cores because of the synthesis of heavy chemical elements by nuclear reactions. Eventually, such stars become unstable and explode as supernovas. The core then collapses until its radius is reduced to about 10 km and its density is of the order of 1011 to 1015 g/cm3. Such stars are composed of a “neutron gas” because electrons and protons are forced to combine under the enormous pressure and the abundance of neutrons greatly increases as a result. Neutron stars have very rapid rates of rotation and emit pulsed radio waves that were first observed in 1965 by newscientist.com Jocelyn Bell, a graduate student working with A. Hewish at the Cavendish Laboratory of Cambridge University in England (Hewish, 1975). 52 Stellar evolution The Crab Nebula contains such a “pulsar,” which is the remnant of a supernova observed by Chinese astronomers in 1054 a.d. (Fowler, 1967). The cores of the most massive stars collapse to form black holes in accordance with Einstein’s theory of general relativity. Black holes have radii of only a few kilometers and densities in excess of 1016 g/cm3. Their gravitational field is so great that neither light nor matter can escape from them, hence the name “black hole.” newscientist.com Observational evidence supporting the existence of black holes is growing and they are believed to be an im portant phenomenon in the evolution of galaxies. 53 Nucleosynthesis Review on Chemistry Atom the smallest particle that cannot be chemically split Proton positively charged particles Neutron particle with no charge Nucleus central part of an atom composed of neutrons and electrons Electron negatively charged particle surrounding the nucleus energy.gov 54 Nucleosynthesis Review on Chemistry energy.gov 55 Nucleosynthesis Review on Chemistry Isotope Atoms of the same element with different atomic mass number energy.gov 56 Nucleosynthesis Nucleosynthesis process that creates new atomic nuclei from pre-existing nucleons, primarily protons and neutrons The origin of the chemical elements is intimately linked to the evolution of stars because the elements are synthesized by the nuclear reactions from which stars derive the energy they radiate in to space. Only helium and deuterium, the heavy isotope of hydrogen, were synthesized during the initial expansion of the universe. energy.gov 57 Nucleosynthesis ▪ Nuclear fusion reaction powers a star for most of its life. ▪ When a protostar born from nebulae or molecular settles down, it becomes a main- sequence star, and fusion reaction happens in its core. ▪ The primary nuclear fusion happens in the star core is the conversion of proton to helium. ▪ However, depended by the mass, stars achieve this conversion in different ways. Proton-proton chain reaction dominates in stars the size of the Sun or smaller a) Proton-proton chain b) CNO cycle Carbon-Nitrogen-Oxigen (CNO) cycle reaction dominates in stars that are more than 1.3 times energy.gov as massive as the Sun 58 Nucleosynthesis a) Proton-proton chain In the cores of lower-mass main-sequence stars such as the Sun, the dominant energy production process is the proton-proton chain reaction. This creates a He-4 nucleus through a sequence of chain reactions that begin with the fusion of two protons to form a deuterium nucleus along with an ejected positron and neutrino. During this process, two hydrogen atoms are firstly merged together into a deuterium atom, which can then be merged with another hydrogen to form He- 3. Then two of the He-3 nuclei can be merged together to form a He-4 atom. This whole reaction releases a large amount of energy in the form of gamma rays. energy.gov 59 Nucleosynthesis b) CNO cycle In higher-mass stars, the dominant energy production process is the CNO cycle, which is a catalytic cycle that uses nuclei of carbon, nitrogen and oxygen as intermediaries and in the end produces a helium nucleus as with the proton- proton chain. CNO reaction is a very temperature sensitive process. Under typical conditions found in stars, catalytic hydrogen burning by the CNO cycles is limited by proton captures. Specifically, the timescale for beta decay of the radioactive nuclei produced is faster than the timescale for fusion. Thus, this kind of CNO cycle converts hydrogen to energy.gov helium slowly, and is called cold CNO cycle. 60 Nucleosynthesis Burbidge, Burbidge, Fowler and Hoyle (B2FH) The entire theory of nucleosynthesis was presented in their study Schramm and Arnett, 1973 Subsequent contributions in nucleosynthesis which was concerned with specific aspects of the theory and its application to the evolution of stars of different masses and initial compositions. energy.gov 61 Nucleosynthesis The abundance of the chemical elements and their naturally occurring isotopes is the blueprint for all theories of nucleosynthesis. The abundances of elements in the solar system are expressed in terms of the number of atoms relative to 106 atoms of silicon. energy.gov 62 Nucleosynthesis 1. Hydrogen and helium are by far the most abundant elements in the solar system, and the atomic H /He ratio is about 12.5. 2. The abundances of the first 50 elements decrease exponentially. 3. The abundances of the elements having atomic numbers greater than 50 are very low and do not vary appreciably with increasing atomic number. energy.gov 63 Nucleosynthesis 4. Elements having even atomic numbers are more abundant than their immediate neighbors with odd atomic numbers (Oddo- Harkins rule). 5. The abundances of lithium, beryllium, and boron are anomalously low compared to other elements of low atomic number. 6. The abundance of iron is notably higher than those of other elements with similar atomic energy.gov numbers. 64 Nucleosynthesis 7. Two elements, technetium and promethium, do not occur in the solar system because all of their isotopes are unstable and decay rapidly. 8. The elements having atomic numbers greater than 83 (Bi) have no stable isotopes, but occur naturally at very low abundances because they are the daughters of long-lived radioactive isotopes of uranium and thorium. energy.gov 65 References Faure, G. (1998). Principles and Applications of Geochemistry: A comprehensive textbook for geology students. 2nd ed. Prentice Hall, Upper Saddle River, New Jersey. 66