GEO006 Week 1 Lecture 1 (PDF)
Document Details
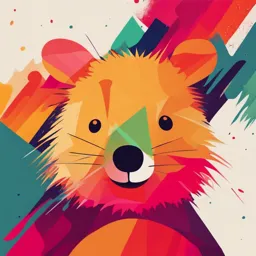
Uploaded by OticSetting3027
University of California, Riverside
Tags
Summary
This document covers the fundamental concepts of the solar system, including the inner and outer planets. It also discusses features like the asteroid belt, and the role of Jupiter. It explores the history of understanding the solar system.
Full Transcript
GEO006 Week 1 Lecture 1 01.07.25 Narrative pathway #1: What is the name of your planet? (Nova’s Notes) Lecture 2: The solar system has a variety of objects that can be divided into different categories: the central star, the planets, comets, asteroids, etc. Inner planets: terrestrial Outer plan...
GEO006 Week 1 Lecture 1 01.07.25 Narrative pathway #1: What is the name of your planet? (Nova’s Notes) Lecture 2: The solar system has a variety of objects that can be divided into different categories: the central star, the planets, comets, asteroids, etc. Inner planets: terrestrial Outer planets Asteroid belt: left over from the formation of the solar system Solar system features: All large bodies orbit in near circular orbits in a pro-grade (anti-clockwise) direction ** moons & planets rotate in an anticlockwise direction Terrestrial planets, closer to the Sun, have a rocky surface, may also have an atmosphere Giant (gas, Jovian) planets, far from the sun, are much more massive than earth, do not have a solid surface Jupiter, Saturn, Uranus, Neptune (both are ice giants) The solar system formed from the material of the sun: asteroids, Kuiper belt, oort cloud (comets) Asteroids require space and are spread out Rocky planetesimals between mars and jupiter did not accrete into a planet Jupiter’s gravity through influence of orbital resonances, stirred up asteroid orbits and prevented their accretion into a planet Orbital resonance: asteroids in orbital resonance with Jupiter experience periodic nudges. Gravitational Shield: Jupiter's immense gravity acts as a barrier, protecting the inner planets (including Earth) from many incoming objects like comets and asteroids. It deflects or captures these objects, preventing them from continuing toward the inner solar system. Asteroid Belt Structure: Jupiter’s gravity helps maintain the asteroid belt's general structure between Mars and Jupiter. While it creates gaps (Kirkwood gaps) in the asteroid belt due to orbital resonance, its influence also prevents the asteroids from coalescing into a planet or scattering completely. Stabilizing Orbits: Jupiter's presence stabilizes the orbits of many smaller bodies. For example, the Trojan asteroids are locked in a stable orbital configuration at Jupiter's Lagrange points (60° ahead and behind Jupiter in its orbit). Ejecting Asteroids: While it protects the inner solar system, Jupiter can also eject asteroids and comets from the solar system entirely by flinging them away through gravitational interactions. This reduces the overall number of potentially hazardous objects. Eventually those nudges move asteroids out of resonant orbits, leaving gaps in the belt Solar system features: Exceptions to the rules Example 1: Uranus is tilted on its side (maybe due to impact) Example 2: Earth has a relatively large moon (popular idea: earth was formed from a large impact) Theia impacted the Earth (same orbit) and the remnants created the moon Why do we see phases of the moon? Lunar phases are a consequence of the moon’s 27.3-day orbit around earth Half of the moon is illuminated by the sun and half is dark We see a changing combination of the bright and dark faces as the moon orbits earth. New moon, Waxing crescent, first quarter, waxing gibbous, full, waning gibbous, third quarter, waning crescent 8 phases Waxing: moon visible in afternoon/evening Becomes “fuller” and rises later each day Waning: Moon visible in late night/morning Becomes “less” and sets Phases being caused by the daylit side Phases of the moon: 29.5-day cycle Each moon should have a phase that makes sense along the story line. Surface gravity: 1.00g- Earth gravity Sister planet, Venus: 0.90 g Pluto: discovered to have 4 moons Lead to the discovery of the kuiper belt What counts as a planet? Definition from the international astronomical union (IAU) 2006: The object is in orbit around the sun. Has sufficient mass to be round. Has “cleared the neighborhood” around its orbit. (pluto is missing this) The planet Hygea: people thought there were 18 planets around the sun. 01.14.25 Lecture 3 Week 2 Narrative Pathway #2: Is your story set in the solar system? Present, past, or future? Giordano Bruno: burned at the stake for his theories that there might be other solar systems that function the same as our solar system, theories of alien existence, planets are “invisible” because light isn’t shone in the dark space Moon was a planet at this time How many exoplanets? 5,811 confirmed exoplanets Kepler results show planet frequency increases towards smaller size–small planets are far more common than larger ones How do we name exoplanets? System named after the star Each component is designated a letter from A-Z, starting with the star (star=a, 1st planet=b,etc.) Planets named in order of discovery, NOT from distance Planet Detection Direct: uses either starlight reflected from or thermal radiation emitted by planet Indirect: uses effects of the planet on the parent star or random background star Gravitational Tug Sun and Jupiter orbit around their common center of mass Sun wobbles around the center of mass with the same period as jupiter Sun's motion around solar systems center of mass depends on tugs from all the planets Astrometric Technique Astronomers who measured this motion around other stars can determine masses and orbits of the planets ○ Detect planets by measuring the change in a star’s position in sky ○ However, these tiny motions are very difficult to measure Doppler Technique Measuring a star’s Doppler shift can tell us its motion toward and away from us The effect of a planet on a star is measured in m/s. ○ Amplitude depends on the planet mass and orbital period Microlensing Predicted by Einstein's Theory of Relativity When a foreground star passes in front of a background star, the light from background star is bent and amplified ○ If foreground star has a planet, there is a spike in the data Transits and Eclipses Eclipse is when planet passes behind the star Transit is when a planet crosses in front of star ○ Results in star becoming dimmer and tells us the planet’s radius ○ Works best for large planets Combining transit radius with the Doppler mass will reveal the planet mean density and composition Transiting Exoplanet Survey Satellite TESS It observes bright stars over the whole sky, enabling detailed follow up Direct Direction Taking a picture Special technique for concentrating or eliminating bright starlight are enabling the direct detection of planets 01.16.25 Lecture 4, Week 2 Earth's Radius: A radius of 6371 kilometers refers to the distance from Earth's center to its surface. Earth's diameter is twice the radius, making it 12742 kilometers (center to one side, through the middle, to the other side). Neptune's Radius in Earth Radii: Neptune’s radius is 3.9 times Earth's radius, meaning Neptune is significantly larger. Comparison of Sizes: Neptune's diameter would be nearly four times Earth’s diameter. This highlights how much larger gas/ice giants like Neptune are compared to terrestrial planets like Earth. Implications in Astronomy: Understanding planetary radii helps estimate their volume, surface area, and gravitational influence. Knowing Neptune's size relative to Earth provides insight into its atmosphere, ring systems, and potential for hosting moons. **super earths: Super-Earths are planets with a mass larger than Earth's but significantly less than that of ice giants like Uranus or Neptune. (REMEMBER THEY ARE EXOPLANETS) Typically, they have masses ranging from 1.5 to 10 times that of Earth. EX: GJ 1214 b was discovered in 2009 using the MEarth Project, which looks for exoplanets around nearby stars. Super-Earth, with a mass approximately 6.5 times Earth's and a radius about 2.7 times Earth's. Host Star: GJ 1214 b orbits a red dwarf star (GJ 1214), located about 40 light-years away in the constellation Ophiuchus. Red dwarf stars are cooler and smaller than the Sun, making planets like GJ 1214 b easier to study. The planet has a thick atmosphere, possibly composed of water vapor or hydrogen. Observations suggest it might be a "water world" with a steamy or hazy atmosphere, but it’s unclear if it has a solid surface beneath. Habitable Zone (also known as the "Goldilocks Zone") is a concept in astronomy describing the specific region around a star where conditions might allow liquid water to exist on a planet's surface. The location of the Habitable Zone depends on the star's size, temperature, and brightness. Around cooler stars (like red dwarfs), the Habitable Zone is much closer, while for hotter stars, it's farther out. Examples in Our Solar System: Earth is in the Sun’s Habitable Zone. Mars is near the outer edge of this zone but is less habitable due to its thin atmosphere. Venus is just outside the inner edge, making it too hot for liquid water. EX: Kepler-186f: Discovery: Found in 2014 by NASA's Kepler space telescope. Location: It orbits a red dwarf star (Kepler-186), about 500 light-years away in the constellation Cygnus. Size: Its radius is about 1.1 times that of Earth, making it a rocky planet like Earth. Habitable Zone: Kepler-186f is the first Earth-sized planet confirmed to be in the habitable zone of its star, where conditions could allow liquid water to exist. This discovery shows that potentially habitable, Earth-like planets could exist outside our solar system. What is the nearest star to the Sun? (D) Proxima Centauri 01.21.25 Lecture 5, Week 3 Planetary Habitability= ability of planet to retain surface liquid water Comes down to water ○ Important for life because: Hydrogen and oxygen compounds are very common water=neutral solvent in which biochemical reactions take place Can be in liquid state at high temps (0-100 degrees C), enabling faster reaction rates For example: saturn has water but it is much colder so its reaction rates are much slower, life progresses along quicker with warm temps than cold temps Stars Blue stars=hot Red stars=cool Stars are usually the primary contributor to a planetary climate energy budget Main Sequence Stars Relationship between size of star and longevity Our sun is “average” because it lays in the middle of our diagram Categories: ○ Blue massive stars: short lifetime, gravity is pushing them down to the middle, nuclear fusion reactions take place quickly…so they do not live long Once they run out of fuel, they produce the most powerful event: supernova explosion ○ Red dwarf stars: live a very long time, are very common, but are very active live about 100 billion years (has not run out of hydrogen) Downside: strong flares all the time, a lot more UV radiation which is harmful for life, the energy is stripped from planets…this brings a question of whether there are atmospheres or not Habitable Zone This zone is the region around a star where water COULD exist in a liquid state on the surface of a planet IF it has sufficient atmospheric pressure ○ One of the common criticisms is that if earth is in the habitable zone so should the moon BUT it can’t because it has no atmosphere MUST have atmosphere It does not mean it should have water, just that if we teleported Earth there, it could continue to exist with water This habitable zone is based on one data point, the Sun Conservative habitable zone: what happens when we move Earth closer to the sun? Water evaporates on the surface and earth starts to warm up, feedback loop–get venus, opposite is mars ○ Venus once had surface water, when Sun was less hot Kepler 452 System Twice the size of earth but same distance from sun as earth Smaller than Earth Planets Occurrence of smaller planets are greater than larger ○ Think of mars (more likely to have their size) Temp is really low What would happen to Mars if we moved it to the orbit of Earth? (A) It would lose the vast majority of its atmosphere. (B) It would become a hot hellscape like Venus. (C) It would become a habitable paradise! (D) Nothing, it would remain the same. Climate/Habitability Possible that plate tectonics are necessary to keep climate of Earth stable enough for life ○ Earth has natural thermostat with CO2 when it gets cold, CO2 increases to maintain temp. Habitable Planets in Eccentric Orbits Narrative Pathway #4: Are there other planets/moons in the system? Role of Giant Planets Other planetary systems do not have giant planets in the same way our solar system does, less than 10% of stars have Jupiter/Saturn analogs Life is limited to those systems who have large planets Role of Jupiter Essential in transporting water from outer to inner parts of planetary systems Necessary to reduce rate of impacts, that otherwise would have hit Earth Earth-like planets are restricted to star systems with Jupiter-like planets Moon Habitability Low tides/high tides ○ Helps move evolution along to facilitate evolution of life EUROPA & ENCELADUS Have water but no atmosphere, potential good life planet 01.23.25 Lecture 6, Week 3 Frank Drake Co-founded the search for extraterrestrial intelligence (SETI) Interested about life in the universe approach about life in the universe was this–want to be friends with extraterrestrial life (keep in mind this was happening during the time of a rise in pacifism) Most of their funding attempts failed Drake Equation Intended to calculate the number of civilizations with whom we could potentially communicate Lowercase f in this equation is a probability= 0-1 ○ 0=means even will never happen ○ 1=will always happen R=rate of star formation in galaxy ○ NOT ALL STARS ARE SUITABLE FOR LIFE Ie hot blue stars fp=fraction of stars with planets ○ Current estimate is around 1 ne= average number of habitable planets per star ○ Current estimate =.5 flife=fraction of habitable planets that develop life ○ We can’t say much but we have one example: Earth Life on earth started pretty quickly As soon as life could form it did form fint.life=fraction of habitable planets with intelligent life ○ Very difficult to determine fciv= fraction of civilization that can communicate with ○ This is the end goal L=length of time that civilization survives ○ Survival time can depend on many internal and external factors The equation is meant to support discussion not to be meant as an actual guide to finding civilizations Enrico Fermi Physicist Contributions to statistical mechanics, quantum theory, and nuclear and particle physics Nobel Prize for his work on radioactivity Manhattan Project and created world’s first nuclear reactor Interested in aliens Fermi Paradox 1. Billions of stars in galaxy, many older than the sun 2. If earth-like planets are common, then intelligent life may also be common 3. Some of these civilizations may develop interstellar travel 4. The milky way galaxy should be completely occupied by now If aliens have expanded where are they? How quickly would galactic expansion occur? Consider advanced civilization expanding from its point of origin Assuming only 1% of planets sends out one ship, it'll take 100,000 years for the colonized planets to become industrial and send out a new ship Species would spread throughout the galaxy in about 250 million years,..small compared to 14 billion years of universe Great Filter It is the dominant factor that prevents dead matter from becoming a galactic civilization Explanation of Fermi Paradox? 01.28.25 Lecture 7, Week 4 Mars: Latin God of War Military god of the Roman army. Festivals were held in March at the starts of military season Prof. Percival Lowell Claimed advanced civilization present on planet mars Led to War of the Worlds–H.G. Wells ○ Used the science of the time to create a narrative War of the Worlds Ending Two brothers narrating Martians were still alive, even when bacteria killed most ○ Decided to take over Venus Earth now is worried about how to respond to these extreme events moving forward ○ Decided to protect borders ○ Response to this created constant paranoia forever Idea was that the Sun is cooling, so if Earth is uninhabitable, man can go to Venus to fight martians off Mars In Other Contemporary Ex. Warhammer , The Expanse ○ Look at Mars as a military resource Mars: Basic Properties Half the size, tenth of the mass of Earth= weak atmosphere Atmosphere ○ Atmospheric pressure has been quickly shrinking to.006 bar (keep in mind Earth= 1 bar) It has had liquid water in the past when it had higher than.5 bar, around 4 bill. years ago ○ Has 96% of CO2 which is good because it acts as a greenhouse gas, enabling the trapping of heat Moon ○ Mars has 2 moons: Phobos, Deimos Travel to Mars from Earth ○ 9 months to get there and 9 months to come back ○ 325k miles away ○ Have to take in account of the movement in Earth along with Mars Have to calculate departure and return ○ Have to worry about communication with Mars Due to light taking a minimum of 4 min to travel between Earth and Mars, communication is slowed down The Martian ○ Accurate but the beginning scene was false because the pressure exerted by the wind is too strong than reality…wind doesn’t exert this much pressure in Mars due to atmospheric pressure Total Recall ○ People live in these cities on Mars, tyrannical dictators try to control the people because of how hard it is to live on Mars, it is easy to cut off supplies for those who go against the dictatorship Challenges to terraforming Mars (some things just can’t change) ○ Lows light levels (further away from sun) ○ Low surface gravity (can not modify, human health and physiology will be affected long term) ○ Long-term sustainability–hard with communication issues and travel issues ○ Atmospheric erosion from solar wind–replenish atmosphere in some way ○ Possible impact risks–closer to asteroid belt ○ Phobos & Deimos–decaying moons 01.30.25 Lecture 8, Week 4 Venus Formed almost same time as Earth ○ Same size as Earth Used to be very similar, but now is very different Evolutionary factors ○ 30% closer to sun so it received twice the amount of the solar energy ○ Rotates slowly backwards (224 days to orbit the sun, 243 days to rotate so its day is longer than its year) ○ Almost vertical rotational axis, no seasons like Earth ○ Little no no surface subduction, so volcanoes that erupt just stay on the atmosphere ○ Negligible magnetic field ○ No substantial moon ○ We see phases of venus because it is closer to the Sun than Earth ○ Lots of volcanoes releasing sulfur dioxide and carbon dioxide Exploration of Venus Vanera most prolific exploration to Venus, starting in the early 60s by the Russians ○ The very first Vanera failed and never left Earth ○ Couple of them failed and missed Venus ○ Had the first pictures once they landed Mariner exploration took pictures of the surface NASA went back to Venus with the Magellan Surface Conditions of Venus Primarily CO2 atmosphere with sulfuric acid haze Surface temp=864 degrees F Surface pressure is 92 times the surface pressure of Earth Greenhouse Gas Venus Venus has a thick CO2 atmosphere so gasses stay in atmosphere forever Silurian Hypothesis Could life have existed on Venus? Starting Conditions between Earth and Venus ○ What caused the divergence between Venus and Earth? Increasing luminosity of the Sun? Loss of plate tectonics? Decreasing magnetic field? Slow rotation? Or maybe a combination of these? Narrative Pathway #5: How has your planet changed through time? Discussion 02.03.25 Brightest Things in the sky #1 sun #2 moon #3 venus, only see it in the morning/evening NOT midnight, because sun is opposite side from planet Orson Welles Radio broadcast, causing panic Runaway Greenhouse Venus Atmosphere is so thick there is a positive feedback loop, so heat gets trapped constantly and never escapes ○ Also clouds keep it from outgassing 02.04.25 Lecture 9, Week 5 Interstellar Devices Human mind has yet to evolve and cope with the scale of moving from planet to planet Science fiction: 3 basic approaches to interstellar travel 1. Fundamental part of the science fiction narrative (travel is the story/plot) 2. Briefly explains or/and depicted–not necessarily dwell on the journey but they go into some effort to explain it a little bit 3. Is essentially ignored Astronomical Unit (AU) Average distance between the earth and the sun (93 million mi) ○ Earth is 1 AU within the Sun ○ Jupiter is 5 AU within the sun Useful unit to use within planetary systems Light Year Distance light travels in a year: 9.5 trillion km Far more useful unit to use within stars Angular Measurements Full circle= 360o 1o= 60’ arcminutes 1/= 60” arcseconds As Earth orbits the Sun, the position of a nearby star appears to shift against the background of more distant stars= parallax Parallax and Distance Parallax is the apparent shift in position of a nearby object against a background of more distant objects One Parsec is the distance at which the mean radius of the Earth’s orbit subtends an angle for a second at a time Sense of scale Proxima Centauri is 1.3 parsecs away from our solar system, and 4 light years away Time to reach the nearest star Using Voyager 2 as an example ○ Voyager 2 passed Neptune and Uranus, and left out of the solar system It is traveling at 55,150 kms/hour ○ The distance to Proxima Centauri is 4.246 light years=1.302 parsecs=40.17 trillion kms Voyager would take 40.17/55,150=728 million hours or 83,000 years to reach the nearest star Solution#1: Travel Faster than Light (FTL) Ex: Star Trek (humans travelling FTL): emerging use of “techno-babble”--dialogue to the engineers so they sound like they know what they’re doing Can we travel faster than light? ○ Einstein formulated the Special Theory of Relativity, including relationship between energy, mass, and the speed of light ○ General Theory of Relativity showed the relationship between space and time Solution #2: Treat all of space and time as a singularity You can go wherever you want, make things up and pass it off as science Ex: Doctor Who and TARDIS Solution #3: Travel through a hole in space time Earliest example of this solution was a Disney movie of 1979 titled, “Black Hole” Physicists think they have found a mathematical loophole that supports the concept of humans being able to travel through wormholes Solution #4: sleep in a hibernation, cryo-frozen state Ex: Pandorum, use cryogenics to be sent to a planet discovered by the Kepler mission, lots of things go wrong Is cryosleep/hypersleep possible? ○ Freezing or thawing biological material results in metabolic slowdown but can also cause ice crystal formation, osmotic shock and membrane damage that will eventually lead to cell death=fatal for most living organisms Solution #5:travel in a generation ship (kind of a non-solution) Load everyone on a ship and generations later will arrive This is a less common idea but it was popular in the 30s and 40s, early stages of science fiction Narrative Pathway #6: what tech. do your characters have Feature Doppler Astrometric Technique Technique Measure Line-of-sight Side-to-side motion (position changes) s velocity shifts (Doppler effect) Best for Large, close-in Large, distant planets planets Works System is edge-on System is face-on best when Detectio Spectral shifts in light Directly tracking star’s position n method Limitatio Hard to detect face-on Requires very precise measurements, works best for ns systems nearby stars