Genetics Unit 1 PDF
Document Details
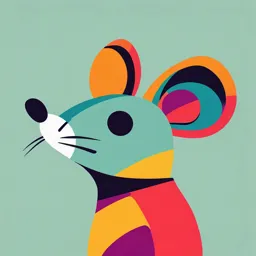
Uploaded by ConsiderateDarmstadtium
Charotar University of Science and Technology (CHARUSAT)
Dr. ANAMIKA JHA
Tags
Summary
This document is a syllabus for a genetics unit, covering topics such as the history of genetics, Mendelism, and the branches of genetics. It also includes information about the central dogma of molecular biology.
Full Transcript
GENETICS Dr. ANAMIKA JHA Assistant Professor, CHARUSAT University BS310 GENETICS: Syllabus Sr. Title of Unit Minimum No. number of hours 1. History of gene...
GENETICS Dr. ANAMIKA JHA Assistant Professor, CHARUSAT University BS310 GENETICS: Syllabus Sr. Title of Unit Minimum No. number of hours 1. History of genetics, Mendelism and its 10 extensions 2. Bases of heredity 08 3. Basic cytogenetics and population 10 genetics 4. Sex Determination 08 5. Basic microbial genetics 09 UNIT-1 History of genetics, Mendelism and its extensions Brief history, branches of genetics and their importance, terminology of the genetics, central dogma of life Mendelism: Mendel’s principles and extensions of Mendel’s law, continuous and discontinuous variation, segregation and independent assortment, multiple alleles and incomplete dominance, epistasis and co- dominance, probability and statistics E. coli Saccharomyces cerevisae Chlamydomonas sp. Neurospora crassa C. elegans ( a nematode) Xenopus laevis big eggs Zebra danio ( transparent development) Arabidopsis thaliana ( a dicot) Drosophila melanogaster WHAT IS GENETICS? “Genetics is the study of heredity, the process in which a parent passes certain genes onto their children.” BRANCHES OF GENETICS 1. Transmission genetics Classical or Mendelian genetics 2. Molecular genetics Chromosomes, DNA, regulation of gene expression Recombinant DNA, Biotechnology, Bioinformatics, genomics, proteomics 3. Population, evolutionary genetics - Allelic frequencies in populations - Effect of migration - Study relatedness of taxa via DNA and protein analysis 4. Quantitative genetics - Effect of many genes History of Genetics People have known about inheritance for a long time. --children resemble their parents --domestication of animals and plants, selective breeding for good characteristics --Sumerian horse breeding records --Egyptian date palm breeding --Bible and hemophilia Old Ideas Despite knowing about inheritance in general, a number of incorrect ideas had to be generated and overcome before modern genetics could arise. 1. All life comes from other life. Living organisms are not spontaneously generated from non-living material. Big exception: origin of life. 2. Species concept: offspring arise only when two members of the same species mate. More Old Ideas 3. As opposed to “preformation”, the idea that in each sperm (or egg) is a tiny, fully-formed human that merely grows in size, organisms develop by expressing information carried in their hereditary material. 4. The environment can’t alter the hereditary material in a directed fashion. There is no “inheritance of acquired characteristics”. Mutations are random events. 5. Female parents are responsible for the sexuality of the offspring. Male and female parents contribute equally to the offspring. Mid 1800’s Discoveries Three major events in the mid-1800’s led directly to the development of modern genetics. 1859: Charles Darwin publishes The Origin of Species, which describes the theory of evolution by natural selection. This theory requires heredity to work. 1866: Gregor Mendel publishes Experiments in Plant Hybridization, which lays out the basic theory of genetics. It is widely ignored until 1900. 1871: Friedrich Miescher isolates “nucleic acid” from pus cells. Major Events in the 20th Century (DNA and genomics era) 1900: rediscovery of Mendel’s work by Robert Correns, Hugo de Vries, and Erich von Tschermak. 1902: Archibald Garrod discovers that alkaptonuria, a human disease, has a genetic basis. 1905: Gregory Bateson discovers linkage between genes. Also coins the word “genetics”. 1910: Thomas Hunt Morgan proves that genes are located on the chromosomes (using Drosophila). 1918: R. A. Fisher begins the study of quantitative genetics by partitioning phenotypic variance into a genetic and an environmental component. More 20th Century Events (genomics era) 1926: Hermann J. Muller shows that X-rays induce mutations. 1944: Oswald Avery, Colin MacLeod and Maclyn McCarty show that DNA can transform bacteria, demonstrating that DNA is the hereditary material. 1953: James Watson and Francis Crick determine the structure of the DNA molecule, which leads directly to knowledge of how it replicates 1966: Marshall Nirenberg solves the genetic code, showing that 3 DNA bases code for one amino acid. 1972: Stanley Cohen and Herbert Boyer combine DNA from two different species in vitro, then transform it into bacterial cells: first DNA cloning. 2001: First draft of Sequence of the entire human genome is announced by Human genome project and Celera genomics. More 21st Century Events 2003 (14 April): Successful completion of Human Genome Project with 99% of the genome sequenced to a 99.99% accuracy. 2003: Paul Hebert introduces the standardisation of molecular species identification and coins the term 'DNA Barcoding 2007: Timothy Ray Brown becomes the first person cured from HIV/AIDS through a Hematopoietic stem cell transplantation 2008: Houston-based Introgen developed Advexin (FDA Approval pending), the first gene therapy for cancer and Li-Fraumeni syndrome, utilizing a form of Adenovirus to carry a replacement gene coding for the p53 protein. 2010: transcription activator-like effector nucleases (or TALENs) are first used to cut specific sequences of DNA. 2011: Fungal Barcoding Consortium propose Internal Transcribed Spacer region (ITS) as the Universal DNA Barcode for Fungi. 2012: The flora of Wales is completely barcoded, and reference specimines stored in the BOLD systems database, by the National Botanic Garden of Wales. 2016: A genome is sequenced in outer space for the first time, with NASA astronaut Kate Rubins using a MinION device aboard the International Space Station. TERMINOLOGY OF GENETICS Factor : The unit responsible for the inheritance and expression of a particular character. Now replaced by the term gene. Gene : It is a particular segment of a DNA molecule which determines the inheritance and expression of a particular character. Alleles or Allelomorphs : Two or more alternative firms of a factor or a gene are called alleles. For example in pea plant, the gene for producing seed shape may occur in two alternative forms: round (R) and wrinkled (r). Genes for round and wrinkled seeds are alleles of each other. Similarly, there are three alleles for gene controlling blood group in man IA, IB, i (I = immunoglobulin gene). Alleles occupy same locus on homologous chromosomes. Trait : is the expressed character, e.g. colour of flower, shape of seed etc. 18 TERMINOLOGY OF GENETICS Dominant trait : Out of the two alternating forms (allelomorphs) of a trait, the one which expresses itself in a heterogygous organism in the F1 hybrid is called the dominant trait (dominant allele) and the phenomenon is called dominance. For example in an organism with Tt, T (tallness) expresses itself and t (dwarfness) cannot, so T is the dominant allele. Recessive trait : Out of the two alternating alleles for a trait, the one which is suppressed (does not express) in the F1 hybrid is called the recessive trait (recessive allele). Recessive allele expresses only in the homozygous state (e.g. tt). Genotype : The genetic constitution of an individual (which he/she inherits from the parents is called the genotype, e.g., the genotype of pure round seeded parent pea plant is RR. Phenotype : The outward (morphological) appearance of an individual for any trait or traits is called the phenotype, e.g. for seeds, round shape or wrinkled shape is the phenotype. 19 TERMINOLOGY OF GENETICS Homozygous : an individual possessing identical alleles for a trait is called homozygous or pure for that trait, e.g. plant with RR alleles is homozygous for the seed shape. Heterozygous : An individual receiving dissimilar alleles for a trait is called heterozygous or impure for that trait, e.g. a plant with Rr alleles is heterozygous for the seed shape. Parent generations : The parents used for the first cross represent the parent (or P1) generation. F1 generation : The progeny produced from a cross between two parents (P1) is called First filial or F1 generation. F2 generation : The progeny resulting from self hybridization or inbreeding of F1 individuals is called Second Filial or F2 generation. 20 TERMINOLOGY OF GENETICS Monohybrid cross : The cross between two parents differing in a single pair of contrasting characters is called monohybrid cross and the F1 offspring as the hybrid. The phenotypic ratio of 3 dominants : 1 recessive obtained in the F2 generation from the monohybrid cross is called monohybrid phenotype ratio (eg. 3 : 1 in Mendelian Inheritance). Dihybrid cross : The cross between two parents in which two pairs of contrasting characters are studied simultaneously for the inheritance pattern is called dihybrid cross. The phenotypic ratio obtained in the F2 generation from a dihybrid cross is called dihybrid ratio phenotypic (e.g. 9 : 3 : 3 : 1 in Mendelian crosses). 21 TERMINOLOGY OF GENETICS Hybridisation : Crossing organisms belonging to different species for getting favourable qualities in the offspring. Test cross : Crossing of the F1 progeny with the homozygous recessive parent. If F1 progeny is heterozygous, then test cross always yields the ratio of 1 : 1. Reciprocal cross : Is the cross in which the sex of the parents is reversed. That is if in the first cross father was dwarf and mother tall, then in the reciprocal cross, dwarf parent will be female and tall parent male. 22 CENTRAL DOGMA Dogma- a principle, belief Proposed by Crick in 1958 Flow of Transfer of information– general mode— DNA-DNA, DNA-RNA, RNA-PROTEIN (Known as central dogma of molecular biology) Exceptions to central dogma Flaw in the rule Special cases: RNA-RNA and RNA-DNA Exceptions to the rule: reverse transcription, RNA replication, Direct translation Central dogma states that “once information has passed into protein it can’t get out again. OR precisely Transfer of information is possible only as follows: DNA RNA Protein Exceptions of central dogma rule: Recent studies have shown that in some special cases Transfer of information is also possible as follows: 1)Reverse transcription: Ex-retroviruses (HIV), retrotransposons in eukaryotes RNA DNA 2) RNA replication: Ex- many viruses, bacteriophages, RNA RNA They have RNA dependent RNA polymerases 3) Direct translation of protein from DNA: demonstrated in cell-free extract containing ribosome from E. coli (in a test tube). Protein could be synthesized from ss DNA templates isolated from other organisms like mouse etc. UNIT-1 1. Central Dogma of molecular biology https://www.khanacademy.org/test- prep/mcat/biomolecules/amino-acids-and-proteins1/v/central- dogma-of-molecular-biology-2 2. Exceptions of central Dogma https://www.khanacademy.org/test- prep/mcat/biomolecules/amino-acids-and- proteins1/v/central-dogma-revisited MENDEL’S LAWS OF INHERITANCE derived by Gregor Mendel, a 19th century monk conducted hybridization experiments in garden peas (Pisum sativum) Between 1856 and 1863 he cultivated and tested some 29,000 pea plants. From these experiments Laws of Heredity or Mendelian inheritance He described these laws in a paper, "Experiments on Plant Hybridization" which was published in 1866. Based on mathematical probabilities Mendel discovered that by crossing white flower and purple flower plants, the result was not a hybrid offspring, rather they were purple flowered. idea of heredity units, called "factors“ (later called genes), one is a recessive characteristic and the other dominant. 28 MENDEL’S LAWS OF INHERITANCE Reasons for Mendel’s success 1. His choice of material was good. He selected garden pea which has a short life cycle, has self pollinated bisexual flowers with closed corolla so pollination Reproduction and Heredity can be easily controlled. Also it is easy to cultivate pea plants and a large number of pure line plants with several pairs of contrasting characters were available. 2. His selection of traits : The seven pairs of contrasting characters of pea plant considered by Mendel in his experiments were responsible for the desired results that helped Mendel postulate the laws of inheritance. 29 MENDEL’S LAWS OF INHERITANCE Reasons for Mendel’s success 3. Mendel’s Technique : His technique of experimentation was excellent and was as follows: (i) Homozygous pure line plants with contrasting characters were crossed. (ii) Self pollination was prevented by removing stamens. (iii) Female plants were dusted with pollen grains from another plant with the opposite feature and were tied in a bag to prevent any further pollination. (iv) Seeds were collected and sown in time. (v) He considered the inheritance of one character at a time. (vi) The results were analysed statistically. (vii) He performed reciprocal crosses and test crosses to confirm the results. 30 MENDEL’S LAWS OF INHERITANCE 31 MENDEL’S LAWS OF INHERITANCE Mendel's Laws Mendel postulated 3 laws, which are now called after his name as Mendel’s laws of heredity. These are: 1. Law of dominance and recessive 2. Law of segregation 3.Law of independent assortment 1. Law of Dominance Definition: When two homozygous individuals with one or more sets of contrasting characters are crossed, the characters that appear in the F1 hybrids are dominant characters and those do not appear in F1 are recessive characters. 32 MENDEL’S LAWS OF INHERITANCE Law of dominance- If there are two alleles coding for the same trait and one is dominant it will show up in the organism while the other won't 33 MENDEL’S LAWS OF INHERITANCE Explanation : on the basis of enzymatic functions of genes. The dominant genes - capable of synthesizing active polypeptides or proteins that form functional enzymes, the recessive genes (mutant genes) code for incomplete or non- functional polypeptides. In the heterozygous condition also the dominant gene is able to express itself, so that the heterozygous and homozygous individuals have similar phenotype. Critical appreciation of Law of Dominance Scientists conducted cross-breeding experiments to find out the applicability of law of dominance. The experiments were conducted by Correns on peas and maize, Tschermak on peas, by De Vries on maize etc., by Bateson and his collaborators on a variety of organisms, by Davenport on poultry, by Furst on rabbits, by Toyama on silk moth and by many others. These scientists observed that a large number of characters in various organisms are related as dominant and recessive. 34 MENDEL’S LAWS OF INHERITANCE Importance of law of dominance The phenomenon of dominance is of practical importance as the harmful recessive characters are masked by the normal dominant characters in the hybrids. In Human beings a form of idiocy, diabetes, haemophilia etc. are recessive characters. A person hybrid for all these characteristics appears perfectly normal. Thus harmful recessive genes can exist for several generations without expressing themselves. 2. Law of Segregation (Purity of Gametes) Definition - The law of segregation states that when a pair of contrasting factors or genes or allelomorphs are brought together in a heterozygote (hybrid) the two members of the allelic pair remain together without being contaminated and when gametes are formed from the hybrid, the two separate out from each other and only one enters each gamete. 35 MENDEL’S LAWS OF INHERITANCE Explanation with Example - Pure tall plants are homozygous, possess genes (factors) TT; dwarf possess genes tt. The tallness and dwarfness are two independent but contrasting factors or determiners. Pure tall plants produce gametes all of which possess gene ‘T’ and dwarf plants ‘t’ type of gametes. During cross fertilization gametes with T and t unite to produce hybrids of F1 generation with genotype Tt. It means F1 plants, though tall phenotypically, possess one gene for tallness and one gene for dwarfness. Apparently, the tall and dwarf characters appear to have become contaminated developing only tall character. But at the time of gamete formation, the genes T (for tallness) and t (for dwarfness) separate and are passed on to separate gametes. As a result, two types of gametes are produced from the heterozygote in equal numerosity. 50% of the gametes possess gene T and other 50% possess gene t. Therefore, these gametes are either pure for tallness or for dwarfness. (This is why the law of segregation is also described as Law of purity of gametes). 36 MENDEL’S LAWS OF INHERITANCE 37 MENDEL’S LAWS OF INHERITANCE 3. Law of Independent Assortment Definition: The inheritance of more than one pair of characters (two pairs or more) is studied simultaneously, the factors or genes for each pair of characters assort out independently of the other pairs. Mendel formulated this law from the results of a dihybrid cross. Explanation: cross between plants having yellow and round cotyledons and plants having green and wrinkled cotyledons F1 hybrids all had yellow and round seeds. After selfing, four types of plants were produced in the following proportion: (i) Yellow and round 9 (ii) Yellow and wrinkled 3 (iii) Green and round 3 (iv) Green and wrinkled 1 38 MENDEL’S LAWS OF INHERITANCE 39 MENDEL’S LAWS OF INHERITANCE Critical appreciation of law of Independent Assortment- The law of independent assortment fails to have a universal applicability. Cytological studies: allelomorphs, located in different homologous pairs of chromosomes only segregate independently during meiosis if allelomorphs for different characters are present in the same homologous pair of chromosomes, these are passed on to the same gamete. Law of independent assortment does not apply to such cases. BIOLOGICAL SIGNIFICANCE OF MENDEL'S LAWS Breeding applications. These are used for improving the varieties of fowls and their eggs; in obtaining rust-resistant and disease-resistant varieties of grains. Various new breeds of horses and dogs are obtained by cross breeding experiments. The science of Eugenics is the outcome of Mendelism, which deals with the betterment of human race. 40 Extensions and Exceptions to Mendels Laws When Gene Expression Appears to Alter Mendelian Ratios A number of factors can appear to disrupt Mendelian ratios. Lethal Allele Combinations Homozygous recessive lethal alleles eliminate a progeny class. Multiple Alleles 1. A gene can have more than two alleles, but a diploid individual only has one or two of them. 2. Different allele combinations can produce different phenotypes and different severities of symptoms (ex- A,B,O Blood groups) Pleiotropy-One Gene, Many Effects 41 Extensions and Exceptions to Mendels Laws Phenocopies-When It's Not in the Genes A trait caused by the environment but resembling a genetic trait or occurring in certain family members is a phenocopy. Maternal Inheritance and Mitochondrial Genes: In maternal inheritance, a trait passes from females to offspring of both sexes, but not from males. Ex- chloroplast and mitochondrial genes Linkage Linkage was discovered in peas when a dihybrid cross did not show a typical 9:3:3:1 ratio, but had an excess of parental types. Codominance and incomplete dominance Epistasis-When One Gene Affects Expression of Another 42 Extensions and Exceptions to Mendels Laws 1. Incomplete dominance Incomplete or partial dominance exists when one allele does not have clear dominance over the other. The phenotype of the heterozygote is intermediate between the homozygous of either allele. For example (1) mating homozygous white snapdragons (Antirrhinum majus) to homozygous red snapdragons yields F1 offspring with pink flowers. (2) In four ‘o’ clock plant (Mirabilis jalapa) there are two types of flower viz., red and white. A cross between red and white flowered plants produced plants with intermediate flower colour i.e. pink colour in F1 and a modified ratio of 1 red: 2 pink: 1 White in F2. 43 Snapdragon sp. showing incomplete dominance Extensions and Exceptions to Mendels Laws Incomplete dominance In this example, the gene dosage for red in the F1 plants is half that of the homozygous red parent, and half as much gene product is made in the offspring. In the F1, the red gene is present, so some red pigment is made (but not as much as in the red parent, which has two red genes) and the flowers are pink. Parents Red flower x White flower RR x rr F1 Rr (pink flower) F2 1 Red (RR) : 2 Pink (Rr) : 1 White (rr) 45 Incomplete dominance in flowers of Mirabilis jalapa Extensions and Exceptions to Mendels Laws 2. Co dominance When alleles of a single gene encode two distinct and detectable gene products, the joint expression of both alleles in the heterozygote is called codominance. both alleles express their phenotypes in heterozygote The example is AB blood group in human. The people who have blood type AB are heterozygous exhibiting phenotypes for both the IA and IB alleles. In other words, heterozygotes for codominant alleles are phenotypically similar to both parental types. The main difference between codominance and incomplete dominance lies in the way in which genes act. In case of codominance both alleles are active while in case of incomplete dominance both alleles blend to make an intermediate one. 47 Codominance - both genes fully expressed 3. Epistasis Definition Epistasis is a form of gene interaction in which one gene masks the phenotypic expression of another. There are no new phenotypes produced by this type of gene interaction. Epistatic versus Hypostatic The alleles that are masking the effect are called epistatic alleles (Dominant epistasis) The alleles whose effect is being masked are called the hypostatic alleles (recessive epistasis) Labrador Retrievers (example of recessive epistasis) Fur color in Labrador Retrievers is controlled by two separate genes. Gene 1: Represented by B : Controls color Gene 2: Represented by E : Controls expression of B Labrador Retrievers If a Labrador retriever has a dominant B allele, they will have black fur. If they have two recessive alleles (bb) they will have brown fur. Labrador Retrievers If a retriever receives at least one dominant “E” allele, they will retain the color that the “B” allele coded for. Either black or brown However, if a dog receives a pair of homozygous recessive “e” alleles, they will be golden regardless of their “B” alleles! Labrador Retrievers BBEE and BbEe --> Black retrievers bbEE and bbEe --> Brown retrievers BBee, Bbee, or bbee --> Golden retrievers Dominant Epistasis Squash fruit color is controlled by two genes. Gene 1 is represented by a W Gene 2 is represented by a G Squash Fruit Color Genotypes and Phenotypes: W-/G- white W-/gg white ww/G- green ww/gg yellow Squash Fruit Color Which allele is epistatic in squash color? The dominant W allele is epistatic How do you know? Because every time a dominant W allele shows up in a squash genotype, the squash fruit color is white. Tall or dwarf, red or white, brown eyes or blue eyes, these are just two varieties or alleles of genes for plant height and flower colour and eye colour The Genes that have 2 or more than two alleles are known as multiple alleles and the phenomenon is known as Multiple allelism About 30% of the genes in humans are di-allelic They exist in two forms, (they have two alleles) About 70% are mono-allelic, they only exist in one form and they show no variation A few are poly-allelic having more than two forms. Combinations Di-allelic genes can generate 3 genotypes AA, Aa and aa Genes with 3 alleles can generate 6 genotypes (3+2+1) Genes with 4 alleles can generate 10 genotypes (4+3+2+1) Genes with 8 alleles can generate 36 genotypes. The ABO blood system Controlled by a tri-allelic gene 6 genotypes The alleles for antigens on the surface of the red blood cells Two of the alleles are codominant to one another and both are dominant over the third Allele IA produces antigen A Allele IB produces antigen B Allele i produces no antigen. Blood types and transfusions Blood types vary and your immune system recognises your own blood type = self Other blood types = non-self If a blood, which is incompatible with your body, is transfused it will result in the agglutination of the foreign red blood cells. Agglutination/clumping of RBCs Blood types and transfusions Type A people produce antibodies to agglutinate cells which carry Type B antigens Recognised as non-self The opposite is true for people who are Type B Neither of these people will agglutinate blood cells which are Type O Type O cells do not carry any antigens for the ABO system, can donate to all blood types (Universal donor) What about type AB people carry antigens for both type A and type B, so can donate blood to AB type only (Universal recipient) Donor-recipient compatibility Recipient Type A B AB O A Donor B AB O Note: Type O blood may be transfused into all = Agglutination the other types = the universal donor Type AB blood can receive blood from all = Safe transfusion the other blood types = the universal recipient. Variation Scientists were looking for inheritance that would explain transmission of characteristics ( e.g. Height, cranium size, longevity) to be measured on a continuous scale Mendel suggested, however that inherited characteristics were discrete and constant with clear cut differences (discontinuous): e.g. Peas were either yellow or green Variation may be due to: Genetic differences Environment Both Inheritance and environment 10 Discontinuous variation shows that: 9 8 7 6 a single gene gives the characteristic 5 4 3 2 and the gene is operating with no 1 0 1 2 environmental effects. discontinuous variation 6 5.5 5 4.5 Continuous variation shows that: either many genes give the characteristic 4 3.5 3 2.5 2 1.5 5 5.5 6 6.5 7 7.5 8 8.5 9 or the gene or genes are operating with environmental effects. continuous variation 1. Discontinuous Variation Sometimes the characteristic has just a few discrete categories (like blood group). The frequency histogram has separate bars (or sometimes peaks).. The characteristics of discontinuous variation : have distinct categories into which individuals can be placed tend to be qualitative, with no overlap between categories are controlled by one gene, or a small number of genes are largely unaffected by the environment Discontinuous characteristics are rare in humans and other animals, but are more common in plants. Some more examples………. Shell colouration in the land snail, Cepaea nemoralis Tongue rolling Attached or unattached earlobes Continuous variation Much of the variation that occurs within a species is to with height, mass, size or shape. Characteristics that do not fall easily into groups. The characteristics of continuous variation : variation is usually controlled by several genes, each of which has an effect. It is difficult to measure the individual contribution of each gene, especially as the environment has an influence on this type of variation There are no separate values but a continuous distribution of values AN INTRODUCTION TO PROBABILITY ❖Scientific method: Hypothesis making, experimentation, data collection, interpretation and comparison with original predictions ❖Because of the stochastic (random) nature of genetics and evolution, we have to rely on the theory of probability. ❖Rounding to a ratio: Mendel converted numbers to ratios and then deduced the mechanism of inheritance. Genetics & Probability Mendel’s laws: segregation independent assortment reflect same laws of probability that apply to tossing coins or rolling dice 76 Terminology The possible outcomes of a stochastic process are called events. (A deterministic process has only one possible outcome.) A stochastic process may have a finite or an infinite number of outcomes. The probability of a particular event is the fraction of outcomes in which the event occurs. The probability of event A is denoted by P(A). Terminology Probability values are between 0 (the event never occurs) and 1 (the event always occurs). Events in which occurrence of one possibility excludes occurrence of other possibility are mutually exclusive events. Events may or may not be mutually exclusive. Events that are not mutually exclusive are called independent events. Unordered events: events whose probability of outcome does not depend on the order on which the events occur. 1.The birth of a son or a daughter are mutually exclusive events. 2. The birth of a daughter and the birth of carrier of the sickle- cell anemia allele are not mutually exclusive (they are independent events). 3. The probability that a family of several children will have 2 boys and 1 girl is same irrespective of their birth order (unordered events). Terminology The sum of probabilities of all mutually exclusive events in a process is 1. For example, if there are n possible mutually exclusive outcomes, then n P(i) = 1 i=1 Simple probabilities If A and B are mutually exclusive events, then the probability of either A or B to occur is the union P(A B) = P(A) + P(B) Example: The probability of a hat being red is ¼, the probability of the hat being green is ¼, and the probability of the hat being black is ½. Then, the probability of a hat being red OR black is ¾. Simple probabilities If A and B are independent events, then the probability that both A and B occur is the intersection P(A B) = P(A) P(B) Simple probabilities Example: The probability that a US president is bearded is ~14%, the probability that a US president died in office is ~19%, thus the probability that a president both had a beard and died in office is ~3%. If the two events are independent, 2.3 bearded presidents are expected to fulfill the two conditions. In reality, 2 bearded presidents died in office. (A close enough result.) Harrison, Taylor, Lincoln*, Garfield*, McKinley, Harding, Roosevelt (*assassinated) Conditional probabilities What is the probability of event A to occur given that event B did occur. The conditional probability of A given B is P(A B) P(A | B) = P(A) Example: The probability that a US president dies in office if he is bearded 0.03/0.14 = 22%. Thus, out of 6 bearded presidents, 22% (or 1.3) are expected to die. In reality, 2 died. (Again, a close enough result.) Probability & genetics Calculating probability of making a specific gamete is just like B calculating the probability in BB 100% flipping a coin probability of tossing heads? B probability making a B gamete? B Bb 50% b 85 Probability & genetics Outcome of 1 toss has no impact on the outcome of the next toss probability of tossing heads each time? probability making a B gamete each time? 50% B 50% Bb b 86 Calculating probability Pp x Pp sperm egg offspring P P PP 1/2 x 1/2 = 1/4 male / sperm P p P p Pp 1/2 x 1/2 = 1/4 p P + female / eggs P PP Pp 1/4 1/2 x 1/2 = 1/2 p Pp pp p p pp 1/2 x 1/2 = 1/4 87 Rule of multiplication Chance that 2 or more independent events will occur together probability that 2 coins tossed at the same time will land heads up 1/2 x 1/2 = 1/4 probability of Pp x Pp → pp P 1/2 x 1/2 = 1/4 Pp p 88 Calculating probability in crosses Use rule of multiplication to predict crosses YyRr x YyRr Yy x Yy yyrr Rr x Rr ?% 1/16 yy rr 89 1/4 x 1/4 1. Apply the Rule of Multiplication AABbccDdEEFf x AaBbccDdeeFf AabbccDdEeFF AA x Aa → Aa 1/2 Got it? Bb x Bb → bb 1/4 Try this! cc x cc → cc 1 Dd x Dd → Dd 1/2 EE x ee → Ee 1 Ff x Ff → FF 1/4 1/64 90 1. Apply the Rule of Multiplication The following genotypes are crossed: AaBbCcDd X AaBbCcDd. Give the proportion of the progeny of this cross having the following genotypes: (a) AaBbCcDd, (b) aabbccdd, (c) AaBbccDd. 91 The cross is broken down into simple crosses and the multiplication rule is used to find the different combinations of genotypes: Locus 1 Aa X Aa = ¼ AA, ½ Aa, ¼ aa Locus 2 Bb X Bb = ¼ BB, ½ Bb, ¼ bb Locus 3 Cc X Cc = ¼CC, ½ Cc, ¼ cc Locus 4 Dd X Dd = ¼DD, ½ Dd, ¼ dd To find the probability of any combination of genotypes, simply multiply the probabilities of the different genotypes: (a) AaBbCcDd ½ (Aa) X ½ (Bb) X ½ (Cc) X ½ (Dd) = 1/16 (b) aabbccdd ¼ (aa)X ¼ (bb) X ¼ (cc) X ¼ (dd) = 1/256 (c) AaBbccDd ½ (Aa) X ½ (Bb) X ¼ (cc) X ½ (Dd) = 1/32 2. Rule of addition Chance that an event can occur 2 or more different ways sum of the separate probabilities probability of Bb x Bb → Bb sperm egg offspring B b Bb 1/4 1/2 x 1/2 = 1/4 + 1/4 b B Bb 1/2 x 1/2 = 1/4 1/2 93 III. Statistics and chi-square How do you know if your data fits your hypothesis? (3:1, 9:3:3:1, etc.) For example, suppose you get the following data in a monohybrid cross: Phenotype Data Expected (3:1) A 760 750 a 240 250 Total 1000 1000 Is the difference between your data and the expected ratio due to chance deviation or is it significant? Chi-square test Test to see if your data supports your hypothesis Compare “observed” vs. “expected” data is variance from expected due to “random chance”? or is there another factor influencing data? null hypothesis degrees of freedom statistical significance 95 Two points about chance deviation 1. Outcomes of segregation, independent assortment, and fertilization, like coin tossing, are subject to random fluctuations. 2. As sample size increases, the average deviation from the expected fraction or ratio should decrease. Therefore, a larger sample size reduces the impact of chance deviation on the final outcome. The null hypothesis The assumption that the data will fit a given ratio, such as 3:1 is the null hypothesis. It assumes that there is no real difference between the measured values and the predicted values. Use statistical analysis to evaluate the validity of the null hypothesis. If rejected, the deviation from the expected is NOT due to chance alone and you must reexamine your assumptions. If failed to be rejected, then observed deviations can be attributed to chance. Process of using chi-square analysis to test goodness of fit Establish a null hypothesis: 1:1, 3:1, etc. Plug data into the chi-square formula. Determine if null hypothesis is either (a) rejected or (b) not rejected. If rejected, propose alternate hypothesis. Chi-square analysis factors in (a) deviation from expected result and (b) sample size to give measure of goodness of fit of the data. Chi-square formula (o − e) 2 X = 2 e where o = observed value for a given category, e = expected value for a given category, and sigma is the sum of the calculated values for each category of the ratio Once X2 is determined, it is converted to a probability value (p) using the degrees of freedom (df) = n- 1 where n = the number of different categories for the outcome. Chi-square - Example 1 Phenotype Expected Observed A 750 760 a 250 240 1000 1000 Null Hypothesis: Data fit a 3:1 ratio. = (o − e )2 (760 − 750 )2 (240 − 250 )2 = + 2 e 750 250 2 = 0.53 degrees of freedom = (number of categories - 1) = 2 - 1 = 1 X2 Table and Graph Unlikely: Reject hypothesis likely unlikely Likely: Do not reject Hypothesis 0.50 > p > 0.20 Interpretation of p 0.05 is a commonly-accepted cut-off point. p > 0.05 means that the probability is greater than 5% that the observed deviation is due to chance alone; therefore the null hypothesis is not rejected. p < 0.05 means that the probability is less than 5% that observed deviation is not due to chance alone; therefore null hypothesis is rejected. Reassess assumptions, propose a new hypothesis. Conclusions: X2 less than 3.84 means that we accept the Null Hypothesis (3:1 ratio). In our example, p = 0.48 (p > 0.05) means that we accept the Null Hypothesis (3:1 ratio). This means we expect the data to vary from expectations this much or more 48% of the time. Conversely, 52% of the repeats would show less deviation as a result of chance than initially observed. 104