Genetic Material Past Paper PDF 2024
Document Details
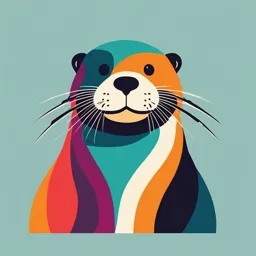
Uploaded by CrisperFoil
Ottawa University
Tags
Summary
This document details the fundamental definitions and principles of genetic material. It provides analysis of experiments by Griffith, Avery, MacLeod, and McCarty, and Hershey and Chase to explore the nature of genetic material.
Full Transcript
Chapter 1. Genetic material Definitions Genetic is a branch of biology which deals with heredity and the expression of hereditary traits. Gene. A unit of genetic information which can be transmitted by an individual to his descendants and which corresponds to a nucleic acid sequence used to specif...
Chapter 1. Genetic material Definitions Genetic is a branch of biology which deals with heredity and the expression of hereditary traits. Gene. A unit of genetic information which can be transmitted by an individual to his descendants and which corresponds to a nucleic acid sequence used to specify the synthesis of an RNA (tRNA, rRNA, snRNA) or a polypeptide chain by translation of an mRNA. Genome. All the genetic information specific to each species of organism, corresponding to the DNA of a haploid batch of chromosomes of this species. Chromosome. Structure consisting of a very long DNA molecule and its associated proteins which carries some (or all) of the hereditary information of an organism. Allele. One of the alternative forms of a gene. In a diploid cell, each gene will have two alleles, each occupying the same position (locus) on homologous chromosomes. Genotype. The specific allelic or genetic constitution of an organism; often the allelic composition of a gene or a limited number of genes. Phenotype. Observable properties of an organism which are genetically controlled. Homozygous. Heterozygous. Individual with different alleles at one or more loci. These individuals produce different gametes and therefore do not constitute pure lines. Homozygote. Individual with identical alleles at one or more loci. These individuals will produce identical gametes and therefore constitute pure lines. 1 1.1 Nature of genetic material 1.1.1. Griffith's experiment (1927) Griffith conducted experiments on different strains of Diplococcus pneumoniae (Streptococcus pneumoniae). Some bacteria were virulent (pathogenic), causing pneumonia in humans and mice, while others were non-virulent. The virulent strains have a polysaccharide capsule and, unlike the non-virulent strains, will not be phagocytosed by mouse phagocytes. Encapsulated bacteria form smooth, shiny colonies on agar plates (S: Smooth), while those without capsules produce rough colonies (R: Rough). Griffith used serotypes II and III (Figure 1). Figure 1 Griffith's transformation experiment. Mice injected with live, non-virulent RII bacteria did not develop pneumonia, ruling out the possibility that RII could have transformed or mutated into SIII. Interactions took place between the live RII bacteria and the heat-killed SIII bacteria. 2 Conclusion: heat-killed SIII bacteria are capable of converting RII bacteria into virulent SIII bacteria. Griffith called this phenomenon transformation and suggested that the transforming principle must be a part of the capsule, or a compound necessary for its synthesis. 1.1.1. The experiments of Oswald Avery, Colin MacLeod and Maclyn McCarty (1944) Chemical nature of the transforming principle They continued Griffith's work and confirmed that DNA is the carrier of genetic information (Figure 2). Figure 2 Experiment demonstrating that DNA is the transforming principle. The results show that a deoxyribose nucleic acid is the transforming principle of Pneumococcus type III. 1.1.1. Alfred Hershey and Martha Chase experiment (1952) Bacteriophages are viruses that infect bacteria. Bacteriophage T2 is one of the viruses of the bacterium Escherichia coli. It consists of a protein envelope containing DNA. Its external structure consists of a hexagonal head surmounted by a tail. The phage adsorbs to the bacterial wall and a component of the phage penetrates the bacteria. After this infection, the viral information controls the host's cellular machinery to produce viruses. What is this component? 3 Hershey and Chase used the radioisotopes P32 and S35. P32 is used to mark DNA (it contains phosphorus) and S35 is used to mark proteins (they contain sulphur). If E. coli bacteria are grown in the presence of P32 and S35 and then infected with T2 phages, the newly synthesised phages will be labelled, either on their DNA or on their proteins. These labelled phages can then be used to infect unlabelled bacteria (Figure 3). Figure 3 Steps in the experiment demonstrating that DNA is responsible for the reproduction of phage T2 after infection of E. coli. The P32-labelled DNA was transferred to the bacteria after adsorption, while the S 35-labelled proteins were not transferred to the bacteria and remained on the ‘ghost’ phages. 4 Conclusion. The phage envelope proteins remained outside the bacteria and were not involved in the production of new phages. The phage DNA entered the bacterium and controlled the production of new phages. The phage's genetic material is DNA and not proteins. 1.1.1. RNA as the carrier of genetic information in certain viruses The central part of some viruses is made up of RNA, while in others it is made up of DNA. Purified RNA from a tobacco mosaic virus (TMV) is spread on tobacco leaves, and the lesions characteristic of viral infection appear on these leaves. It was concluded that RNA is the carrier of genetic information in this virus. Shortly afterwards, Fraenkel-Conrat H. and Singer B. discovered that the RNA and protein envelope of the TMV virus, as well as those of other viruses, could be separated and isolated. They were able to carry out the following experiments (Figure 4). The protein part of the virus is not involved in the characteristics of the offspring. The factor determining hereditary characteristics is RNA. Figure 4 Production of hybrid tobacco mosaic virus. The hybrid virus has the RNA of the wild- type TMV virus and the proteins of the HR strain. The viruses produced after infection with the hybrid strain are made up of wild-type proteins. 1.1 Structure of nucleic acids (DNA-RNA) 1.1.1. Chemical characterisation and structure of nucleic acids Nucleic acid is a polymer of nucleotides (polynucleotide chain). The nucleotide is made up of three components: a nitrogenous base, a pentose (sugar with 5 carbon atoms) and a phosphate group. Nucleic acids contain two purines and three pyrimidines (Figure 5a). Purines: adenine (A) and guanine (G) Pyrimidines: cytosine (C), uracil (U) and thymine (T). 5 Figure 5 (a). Chemical structure of the pyrimidines and purines that constitute the nitrogenous bases of RNA and DNA. DNA and RNA both contain adenine (A), cytosine (C) and guanine (G); only DNA contains thymine (T) and only RNA contains uracil (U). The pentoses present in nucleic acids give their name to the molecule. Ribonucleic acid (RNA) contains ribose, while deoxyribonucleic acid (DNA) contains deoxyribose (figure 5 b). Figure 5 (b). Chemical structure of ribose and 2-deoxyribose, which constitute RNA and DNA respectively. If a molecule is composed of a purine or pyrimidine and a ribose or deoxyribose, the chemical unit is called a nucleoside. If a phosphate group is added to this nucleoside, it becomes a nucleotide (figure 5 c). 6 Figure 5 (c). Structure of the nucleotides and nucleosides that constitute RNA and DNA. Nucleotides are also known as nucleoside monophosphates (NMP). The addition of one or two phosphate groups leads to diphosphate nucleosides (NDP) and triphosphate nucleosides (NTP) (figure 5 d). The triphosphate form is important because it serves as a precursor for nucleic acid synthesis in the cell. Figure 5 (d) Basic structure of nucleoside diphosphates and triphosphates. Example of deoxythymidine diphosphate and deoxyadenosine triphosphate Deux mononucléotides sont liés entre eux au niveau de leur sucre par un groupement phosphate. C’est une liaison phosphodiester (acide phosphorique lié à deux fonctions alcool par une liaison ester de chaque côté) (figure 6). 7 Figure 6 (a) Two nucleotides linked by a phosphodiester bond (3‘-5’) between carbons C-3‘ and C-5’ to form a dinucleotide. Figure 6 (b) Schematic representation of a polynucleotide chain. 1.1.1. Structure of deoxyribonucleotide acid (DNA) DNA is the carrier (support) of genetic information in all organisms (with the exception of certain viruses). But what is the precise structure of DNA? Between 1940 and 1953, a great deal of work was carried out by scientists such as Erwin Chargaff, Maurice Wilkins, Rosalind Franklin, Linus Pauling, Francis Crick and James Watson to discover the structure and function of DNA. 1.1.1.1. Study of base composition, Erwin Chargaff (1950) Between 1994 and 1953, the American biochemist Erwin Chargaff and his colleagues used chromatography techniques to separate the four nitrogenous bases from DNA samples from different organisms. The results show that : - The quantity of adenine is proportional to the quantity of thymine in the DNA of many species. Similarly, the amount of guanine is proportional to that of cytosine (A = T and C = G). - The sum of purines (A+G) is equal to that of pyrimidines (C+T). 8 - The percentage of C + G is not necessarily equal to that of A + T. The ratios between the two values vary considerably between species. Table I. Base composition of DNA in different organisms (corroborates Chargaff's data). Base composition Ratio Ratio Organism A T G C A/T G/C A+G/C+T A+T/C+G man 30,9 29,4 19,9 19,8 1,05 1 1,04 1,52 Sea urchin 32,8 32,1 17,7 17,3 1,02 1,02 1,02 1,58 Bacteriophage T7 26 26 24 24 1 1 1 1,08 A+G=T+C: Chargaff ratio; this ratio provides information about the structure of DNA. A+T/C+G is variable and provides information about the base richness of the DNA. This ratio is called the Chargaff coefficient. A + T + C + G = 100%, whatever the organism. 1.1.1.1.X-ray diffraction analysis When a DNA molecule is exposed to X-rays, these rays are diffracted according to the atomic structure of the molecule. Several researchers have used this method, with Rosalind Franklin (1950-1953) obtaining the best results. She suggested that the structure of DNA was probably a sort of helix. 1.1.1.2.The James Watson and Francis Crick model (1953) Taking into account the previous information, Watson and Crick proposed that the structure of DNA was a double helix (Figure 7) with the following characteristics: Two long polynucleotide chains are coiled around an axis, forming a double helix; The two chains are antiparallel; one is in the 5̍ 3̍ direction, and the other in the opposite direction; The nitrogenous bases of the two chains are horizontal and perpendicular to the central axis; these bases are stacked on top of each other with a spacing of 0.34 nm and are oriented towards the inside of the double helix. The bases of the two chains are paired with each other by hydrogen bonds; in DNA we only find the pairings A=T and G≡C. A complete turn of the helix is 3.4 nm long and consists of ten bases per chain. The diameter of the double helix is 2 nm. 9 Figure 7 (a) Watson and Crick's model of the double helix. The pink ribbon symbolises the ose- phosphate skeleton of the molecule and the horizontal bars represent the nitrogenous bases (10 per helix turn). The vertical black bar represents the axis of the double helix. (b) Detailed view of the double helix. (c) Illustration of the antiparallel nature of the double helix and the horizontal stacking of the nitrogenous bases. 1.1.1. RNA structure Chemically, its structure is identical to that of DNA, but single-stranded. The sugar ribose replaces deoxyribose and the nitrogenous base uracil replaces thymine. There are two exceptions: - RNA molecules can sometimes fold back on themselves to form double-stranded regions; - some viruses have RNA in the form of a double-stranded helix. Three classes of RNA are needed to express genetic information: ribosomal RNA (rRNA), messenger RNA (mRNA) and transfer RNA (tRNA). These molecules are all complementary copies of one of the two strands of DNA and are synthesised during transcription. rRNA is an essential structural component of ribosomes, mRNA transports genetic information from the DNA to the ribosome where translation takes place, and tRNA transports the amino acid to the ribosome during translation. There are functional RNAs (fRNAs) snRNA: small nuclear RNA, play a role in the maturation of mRNA (splicing) and rRNA snoRNA: small nucleolar RNAs, involved in the maturation of rRNAs, siRNAs and miRNAs: small RNAs capable of interfering with mRNAs and modulating gene expression. 10 1.1.2. Physicochemical properties of nucleic acids Electrical charge Nucleic acids are negatively charged due to their phosphate groups. Placed in an electric field (electrophoresis), they will migrate towards the positive pole. UV absorption They absorb UV light at a wavelength between 254 and 260 nm due to the interaction between UV light and the purine and pyrimidine molecule cycle. The A, T, C, G and U bases have a maximum absorption at 260 nm. Denaturation and renaturation When the DNA double strand is denatured, the hydrogen bonds between the two strands are broken and the two strands separate. During the separation of the two strands, induced by heat or chemicals, UV absorption increases. The G≡C pairing is more stable than the A=T pairing because it has an extra hydrogen bond. Cooling the DNA molecule that has been denatured by heat allows the complementary strands to reassociate; the denaruration is reversible, known as renaturation. Degradation They can be degraded by chemical reagents or enzymes (nucleases). 1.2 DNA replication in prokaryotes and eukaryotes Replication is the process by which DNA is synthesised. A parent DNA molecule is copied and gives rise to two daughter DNA molecules identical to the parent molecule. In vitro DNA synthesis: Experiment by Arthur Kornberg et al (1957) The series of experiments carried out by Kornberg and his group determined that in vitro DNA synthesis requires : Four deoxyribonucleoside triphosphates (dNTPs: dATP, dCTP, dGTP, dTTP) ; A double-stranded DNA matrix able of serving as a template; DNA polymerase I: enzyme isolated from E. coli, able of directing DNA synthesis; Mg++ ions (Figure 8). Figure 8 Chemical reaction catalysed by DNA polymerase I. At each stage of synthesis, a nucleoside triphosphate is used as a substrate for the addition of a single nucleotide to the complementary strand of the template. The release of an inorganic pyrophosphate provides the energy for the reaction. 11 DNA synthesis occurs place in the 5‘ to 3’ direction (Figure 9). Figure 9 Demonstration of DNA synthesis in the 5‘ to 3’ direction. Watson and Crick suggested that if the helix was unwound, each strand could serve as a template for the synthesis of its complementary strand (Figure 10.1) Figure 10.1 Generalized model of semi-conservative DNA replication. The neosynthesised strand is shown in blue. Replication models : Three possible modes of replication (Figure 10.2). 12 Figure 10.2 Result of a DNA replication cycle for each of the three possible replication modes. Semi-conservative replication. Each replicated DNA molecule is constitute of a parental strand and a ‘new’ strand. Conservative replication. After synthesis, the two new strands associate and the two parental strands reassociate. The original helix is preserved. Dispersive replication. Each strand contains both parental DNA and newly synthesised DNA. 1.3.1. Semi-conservative replication in bacteria: Matthew Meselson and Franklin Stahl (1958) They cultured E. coli for several generations in a medium culture whose nitrogen source was 15 NH4Cl (a heavy isotope of nitrogen). Molecules containing 15N were denser than those containing 14N. Bacteria labelled with 15N were transferred to a medium containing only 14NH4Cl. As a result, the DNA neosynthesised during replication contained only the lightest nitrogen isotope. The bacteria multiplied over several generations. After one generation, the isolated DNA was present in a single band of intermediate density, as expected for semi-conservative replication; each replicated molecule is made up of a new 14N strand and an old 15N strand (Figure 11). After two divisions, two DNA bands of different density appear, an intermediate band and a lighter band (corresponding to the position of the 14N in the gradient). 13 Figure 11.1 Meselson-Sthal experiment Figure 11.2 Results expected in the Meselson-Stahl experiment after two generations of semi-conservative replication. 1.3.2. Replication in prokaryotes 1.3.2.1. Origin of replication: Cairns (1962) Cairns studied replication in E. coli, using radioactive DNA precursors and autoradiography. He demonstrated that there is only one origin of replication in this bacterium, called oriC (DNA segment containaing a specific nucleotide sequence), the single origin characterising bacteria whose genome is a circular chromosome. 14 A replication protein recognises the sequence and initiates DNA duplication. It attaches to it and separates the two strands, forming a replication ‘eye’. This creates two replication forks, which move in opposite directions along the DNA double strand. Replication is bidirectional. These two forks eventually merge when replication comes to an end at a region called ter (Figure 12). Figure 12 Bidirectional replication of the E. coli chromosome. The thin arrows indicate the progression of the replication forks. 1.3.2.1.Replication mechanisms The enzyme responsible for synthesising the new strand is DNA polymerase III. This adds nucleotides in the 5‘ 3’ direction, respecting the complementarity of the template strand. Replication is complementary and antiparallel. Synthesis is continuous on the leading strand and discontinuous on the lagging strand (Figure 13). Figure 13 On the lagging strand, synthesis is discontinuous, leading to the production of Okazaki fragments. On the leading strand, synthesis is continuous. RNA primers are required to initiate the synthesis of both strands. 15 Initiation involves recognition of the original sequence and opening of the DNA double helix, in a region generally rich in AT. At the moving fork : Helicase unwinds the double helix and breaks the hydrogen bonds ; Single-Stranded Binding (SSB) proteins, bind to DNA preventing the reformation of the helix (they stabilize the open conformation); topoisomerase (DNA gyrase) reduces DNA strand supercoiling; each half of polymerase III (dimeric) is linked to one of the strands by a β subunit, DNA polymerase III requires a primer with a free 3'-hydroxyl end to elongate the polynucleotide chain (elongation), the primer is a segment of RNA (complementary to the DNA template), synthesised by an RNA polymerase called primase. Using this RNA fragment as a starting point, DNA polymerase III adds 5'-deoxyribonucleotide. On the lagging strand, DNA polymerase I replaces the RNA primers with DNA, and DNA ligase connects the Okazaki fragments (5' to 3') (Figure 14)." Termination occurs when the forks meet; a topoisomerase IV with ligase activity links the newly synthesized strands into a circular molecule." Figure 14 Résumé de la réplication de l’ADN bactérien. 16 Table II The proteins involved in bacterial DNA replication and their functions Protein Function in the formation of the leading strand and the lagging strand Helicase Unwinds the parental double helix at the replication forks. Single-stranded binding Binds to single-stranded DNA and stabilises it until it can be used as proteins a template. Topisomerase Corrects supercoiling upstream of the replication forks by cutting and reattaching the DNA strands after allowing the cleaved strand to unwind. Function in continuous Function in discontinuous strand strand formation formation Primase Synthesises a single RNA Réunit les fragments d’Okazaki. primer at the 5' end of the Synthesises an RNA primer at the 5' continious strand. end of each Okazaki strand. ADN pol III Continuously synthesises the Elongates each Okazaki fragment leading strand by addition to by addition to a primer. the primer. ADN pol I Removes the primer from the Remove the primer from the 5‘ end 5‘ end of the leading strand of each fragment and replace it with and replaces it with DNA at DNA by addition to the 3’ end of the the adjacent 3’ end. adjacent fragment. ADN ligase Links the 3' end of the DNA Joins the Okazaki fragments. replacing the primer to the rest of the leading strand. In prokaryotes, 3 DNA polymerases are involved in DNA replication: DNA pol I, through its exonuclease activity, participates in DNA repair. DNA pol II is involved in repairing DNA damaged by external factors (UV). DNA pol III: is responsible for 5‘ 3’ polymerisation, which is essential for replication. Its 3' to 5' exonuclease activity provides a proofreading function to check for incorrect nucleotides. If this occurs, synthesis stops, and the polymerase removes the incorrect nucleotide. Table III: Properties of Prokaryotic DNA Polymerases Polymerases I II III Properties Initiation of synthesis - - - 5' to 3' polymerisation + + + 3' to 5' exonuclease activity + + + 5' to 3' exonuclease activity + - - Exonuclease activity. The exonuclease is an enzyme which has the ability to cut one nucleotide at a time, either in the 5' to 3' direction or the reverse, this cut is made from one end. 1.3.2. Replication in eukaryotes Eukaryotic DNA is replicated similarly to bacterial DNA. However, eukaryotic cells contain more linear DNA associated with proteins, making replication more complex. There are 17 multiple origins of replication per chromosome (20,000 to 30,000 in humans), and various types of DNA polymerases. Table IV Properties of some Eukaryotic DNA polymerases Polymerases α β δ ε γ ζ Localisation Nucleus Nucleus Nucleus Nucleus Mitochondria Nucleus Activity 3´ 5´ exonuclease No No Yes Yes Oui No Essential for nuclear replication? Yes No Yes Yes No No α: α-primase with 5‘-3’ polymerase activity. It is involved in synthesising an RNA primer that it extends. β: Involved in DNA repair and fills gaps left after the removal of RNA primers. δ: has polymerase activity (5‘ 3’) and exonuclease activity (3‘ 5’). It is involved in DNA repair. ϒ: mitochondrial DNA replication. ε: major polymerase in the DNA repair system by excision of nucleotides, can substitute for DNA polymerase δ during replication and repair. Linear chromosome ends and replication Eukaryotes have specific nucleotide sequences at the ends of their chromosomes, known as telomeres. Telomeres comprise numerous tandem repeats of a short nucleotide sequence. In humans, this sequence is 5‘-TTAGGG-3’, and is repeated approximately a thousand times at each telomere. During replication at the ends of a double-stranded DNA, the synthesis of the leading strand proceeds normally to the end of the chromosome. However, a difficulty arises for the lagging strand once the RNA primer is removed (Figure 15.1). Figure 15.1 Difficulty in replicating the end of linear chromosomes. The newly created gap (to the right of gap b) should be filled by adding nucleotides to the 3‘- OH group provided by discontinuous synthesis, since this is the end of the DNA molecule, there 18 is no strand present to provide a 3’-OH group at each cycle of synthesis, which shortens the end of the chromosome. Telomerase replicates chromosome ends Telomerase recognises an existing repetitive sequence at the end of a telomeric DNA and extends it in the 5' to 3' direction. These repeats fold back on themselves to form a "hairpin loop," creating a 3’-OH group that can serve as a substrate for DNA polymerase I. The loop will be cleaved, preventing the loss of DNA with each replication cycle (Figure 15.2). Figure 15.2 Telomerase replicates chromosome ends 1.4 Chromosome organisation 1.4.1. Organisation of bacterial and viral DNA Virus chromosomes consist of a single- or double-stranded nucleic acid molecule (DNA or RNA). They can exist in circular or linear form. This genetic material is often associated with proteins. Bacterial chromosomes consist of a double-stranded DNA molecule, generally circular, organised in supercoils and associated with proteins to form the nucleoid (region containing DNA, inside the cytoplasm). Plasmids may be present. A plasmid is a small circular extrachromosomal double-stranded DNA molecule present in a varying copy numbers. 1.4.2. Organisation of eukaryotic nuclear DNA The carriers of nuclear DNA are the chromosomes, each consisting of a single DNA molecule "wrapped" around packaging proteins, called histones. 19 Chromatin and nucleosome Chromatin. Complex of DNA, histones and non-histone proteins present in the nucleus of a eukaryotic cell. Material from which chromosomes are formed. Nucleosome. The bead-like structure of eukaryotic chromatin. It is composed of a short length of DNA wrapped around a core of histones and represents the fundamental structural unit of chromatin (see Figure 16). Figure 16 Nucleosome structure Histones: Proteins that are complexed with DNA in the nucleus. They are rich in basic amino acids, arginine and lysine, and facilitate the wrapping of DNA to form nucleosomes. 1.4.3 Organization of Mitochondrial DNA DNA is double-stranded and circular, organized into nucleoids, and not associated with histones. In each human cell, the nucleus contains 46 chromosomes, and the mitochondria (100 to 1000 per cell) contain 5 to 10 DNA molecules per mitochondrion. 20