Genetic Disease_Transcript.docx
Document Details
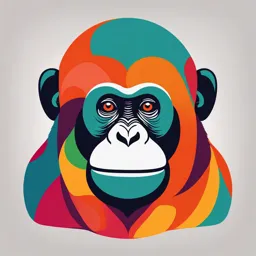
Uploaded by ThrivingSpring
Cardiff University
Tags
Full Transcript
**Basic Genetics & Genetic Disease** **Divided into 3 parts:** Part 1: Chromosomes & DNA packing, and touch upon Inheritance of genetic disease Part 2: Single gene mutations -- how these cause disease Part 3: Chromosomal abnormalities: whether due to a structural abnormality or abnormal number o...
**Basic Genetics & Genetic Disease** **Divided into 3 parts:** Part 1: Chromosomes & DNA packing, and touch upon Inheritance of genetic disease Part 2: Single gene mutations -- how these cause disease Part 3: Chromosomal abnormalities: whether due to a structural abnormality or abnormal number of chromosomes, and then move onto Multifactorial disease and Teratology Slide 2: **Somatic cells contain chromosomes** Chromosomes store genetic information within chromosomes Genetic information is contained within long strands DNA wound round positively charged proteins called histones, giving that beads on a string appearance of chromatin Our somatic cells are diploid cells, which means they have 2 sets of chromosomes, which are paired and homologous. Each somatic cell shas 46 chromosomes: 22 pairs of autosome + a single pair of sex chromosomes which can be either XX or XY. Slide 3: **Chromosomes** Carry genetic information in a Unique sequence of DNA bases The building blocks of DNA are nucleotides which are made up of a nitrogenous base, a sugar ie deoxyribose) as well as phosphate group Each cell contains 3x 10^9^ nucleotides which join together to form DNA strands. 2 DNA strands, composed of polynucleotides, wrap (wind) around each other to form an antiparallel helix with bases projecting interiorly. If we look at diagram we can see there are 4 DNA bases: Guanine, cytosine, Adenine, thymine, which have a unique pairing ie. Guanine (G) pairs with Cytosine (C) and Adenine (A) pairs with Thymine (T) **Slide 4: DNA -\> Protein** Important to remember that the linear sequence of nucleotides within a gene will code for a specific sequence of amino acids, which in turn codes for a specific protein. A codon codes for a single amino acid and a codon is comprised of 3 bases. **Slide 5: DNA Organisation into Chromosomes** Reminder: DNA double helix is wrapped around those positively charged histone proteins to form chromatin, which undergoes further packing to be tightly packed into the 30nm chromatin fibre. This chromatin fibre then underoes further folding + supercoiling until it is packed into rod-shaped chromosomes. Chromatin is at its most condensed during mitosis and this is why we can see the chromosomes under a microscope during metaphase when the spindles separate the 2 chromatids DNA is packed into a 5μm diameter nucleus. DNA is 2 m long, so you can see the importance of all the packing, folding and supercoiling to get that DNA into those rod-shaped chromosomes. **Slide 6: Human Chromosomes** Witihn a somatic cell, each cell has 23 paired chromosomes, including 22 pairs of non-germline (autosomal) chromosomes and a single pair germline which are two Xs ie XX for a female and one X and one Y for a male. Female have 44 autosomes + XX Male has 44 autosomes + XY On the left you can see one of each of these paired chromosomes i.e. 22 chromosomes lined up, and numbered 1 through to 22. They have a constriction within the chromosome which is called a centromere. **Slide 7: Chromosome Identification** Chromosomes can be identified to provide a person's karyotype. Chromosomes in mitosis are stained with Giemsa and lined up as we can see in the image to determine the karyotype. Here we can see 46 chromosomes: 22 pairs of autosomal chromosomes and an X and a Y chromosome, So this is a karyotype for a male ie 46XY Each of the homologous pairs of chromosomes carries a copy of the gene. Each gene copy is known as an allele. If the two alleles or gene copies are identical, this is known as homozygous. If there are two different alleles, this is known as heterozygous **Slide 8: Inheritance of Chromosomes** We inherit one set of chromosomes from each parent ie one set of autosomal chromosomes from mother and one set from the father, plus an X from the mother and either an X or a Y from the father. Our parents have somatic diploid cells with 46 chromosomes, and after meiotic division of the germ cells, the ovum and sperm have 23 chromosomes i.e. are haploid. The sperm and ovum undergo fertilisation to produce a zygote, which will have a diploid number of chromosomes i.e. 46 chromosomes. As this fertilised ovum (zygote) undergoes mitosis to produce the offspring, the offspring will have a diploid quota of chromosomes within their cell ie each cell has 46 chromosomes. These 46 chromosomes provide the genotype of a person. How that genotype is expressed in terms of how we look and various features is known as the phenotype. **Slide 9:** **Basic Genetics & Genetic Disease Part 2: Single gene mutations** In this part 2 of this topic we are going to look at how single gene mutations can cause genetic disease. **Slide 10: Single gene disorders** Important to note that gene mutations are key to human variation and that a gene mutation is simply a change in DNA sequence ie a change in genetic information. We have billions of nucleotide and we often acquire mutations throughout life ie acquired mutations. Also, gene mutations can be passed on to children from parents and these are called inherited gene mutations. Many of the mutations that we have in our genome are silent and harmless and may have no effect on health. Essentially gene mutations are stable heritable changes in DNA that can be inherited in a Mendelian fashion. The Mendelian inheritance pattern may be autosomal dominant, autosomal recessive or X-linked. There are \> 3000 genetic disorders that arise due to a single gene mutation and these gene mutations, like normal genes, will be inherited in Mendelian fashion. Individually each gene disorder is extremely rare, and the gene may have a varying degree of penetrance. By this we mean, that the trait that the gene encodes ie phenotypic expression may be expressed in varying amounts depending on whether the gene has high or low penetrance. For example a gene that has high penetrance such as 95%, this means that it is highly likely that the phenotypic trait of the gene is expressed if that gene is carried. **Slide 11: Mendelian inheritance** Here we can see how genes are inherited in Mendelian fashion. If someone is heterozygous for a mutated gene (blue) ie caries 1 copy of the mutated/abnormal gene, and they have offspring with someone that does not carry any copy of this gene, 50% of offspring will be: Heterozygotes and 50% will not carry the mutated gene. If homozygote (carries 2 copies of the mutated gene) have offspring with someone that does not carry any copy of this gene, 100% offspring will be heterozygous for the mutated gene as they will inherit 1 copy of the mutated/abnormal gene from 1 parent and a normal copy of the gene from the other parent. In the case of 2 heterozygote parents, the offspring will be 25% homozygous (inherit 2 mutated gene copies) and 50% heterozygous (inherit 1 mutated and 1 normal gene copy) for the mutated gene, while 25% will not carry the mutated gene. **Slide 12: Autosomal Dominant** Whether the disease is expressed or not is determined by whether the gene is inherited via autosomal dominant or autosomal recessive inheritance. Note that 'autosomal' refers to the gene involved being on one of the autosomes i.e. one of the chromosome pairs 1-22. In case of autosomal dominant traits, because the gene is dominant, autosomal dominant traits/diseases develop when only one copy of the gene has a mutation that interrupts the genes normal function. This is because the dominant allele will mask the effect of its paired allele. So if a person carries one abnormal gene copy (mutant allele) and the partner allele is normal, they will be heterozygote for the disease and will express disease. If a person carries 2 abnormal genes i.e. is homozygous, the disease will be expressed. **Slide 13: Autosomal Recessive** In the case of autosomal recessive disorders which account for 2.5% of live births in UK, the recessive allele can have its effect on a trait masked by a more dominant allele. In order for disease to be expressed, a person would need to be homozygous for the disease to be expressed ie have 2 mutant alleles In case of a heterozygous carrier, the recessive gene copy is masked by the more dominant gene allele, so a person would not express disease **Slide 14: Sex-linked Inheritance** Sex chromosomes are different from autosomes, the X and Y chromosomes do not carry the same genetic information. In a male who has and X and a Y chromosome, there are genes on the X chromosome that he will only have one copy of, while a female who has two Xs, has 2 copies. Disease or traits that are associated with a mutation in a gene on the X chromosome are inherited differently by a males and females. If males have a mutation on the X chromosome, it is likely that the disease will be expressed, for example in the X-linked recessive disorder haemophilia. Females have two Xs, so if they have a mutation in only one of the X, it is likely that the other X will mask the effect of the mutated allele, unless both Xs are affected, and the chance of this is 1 in 100 million. Therefore, if only 1 X is affected, the female would be a carrier of the disease. Example provide is haemophilia, an X-linked recessive disorder that leads to impaired clotting due to deficiency in a clotting factor. In haemophilia A, factor VIII deficiency occurs and in haemophilia B: factor IX deficiency occurs making people who have this disease at risk of haemorrhage. See diagram (RHS): if an unaffected father, who does not carry a copy of the X-linked recessive gene, has children with a mother who is a carrier of the mutated gene, you can see that 50% of the offspring, whether a son or a daughter, will be unaffected as they do not inherit the mutant allele. 25% of the offspring, if they are female will be a carrier as only one allele is expressed, and the other 25%, in the case of a son who has only one X chromosome, if the mutant allele is inherited, the son will have haemophilia. **Slide 15: Single Gene Disorders** Single gene disorders can arise due to a single gene mutation as a result of: - a single base change where a nucleotide is either inserted or deleted; or due to a rearrangement of intron-exons - fusion of genes can also result in a gene mutation, where a large amount of DNA has been deleted such that two neighbouring genes that are close together fuse and recode to produce a hybrid protein. For instance, you can have you can have the initial sequence of one protein fused to the terminal sequence of another protein. - a whole gene may be deleted if DNA deletion is extensive such that the coding region for the gene is removed. The consequence of these gene mutations could be no effect, incompatibility with cell survival i.e. spontaneous removal of the cells, absence of normal protein or the formation of an abnormal protein. The latter two can lead to disruption of normal function and disease. **Slide 16: Point Mutations** Point mutations describe when there is a spontaneous replacement of one base with another. These occur spontaneously with no known cause, thought to be due to a copying error. The consequence is that new amino acids are coded and therefore a new protein may be formed. Let's look at different examples of point mutation. - Here we have a [missense] mutation where a single base within a codon is swapped so that a new amino acid is coded for. - In the case of a [nonsense] mutation, which accounts for 5% of point mutations, here we have a base change leading to a codon that codes for a **stop** codon. A stop codon is important as it will mean that translation is halted early at the stop codon so that a [nonsense] sequence results and the amino acid sequence is stopped too early so that the protein is not formed. - In the case of [frameshifts,] a single base is either inserted or deleted. If inserted, the reading frame is shifted to the right. If a base is deleted, the reading frame is shifted to left. Consequence is that the sequence of all subsequent amino acids is changed. **Slide 17:** **Single Gene Disorders** Here are number of examples of single gene disorders that can be inherited by autosomal dominant (AD) or autosomal recessive (AR). - Marfan Syndrome is inherited in AD fashion, the gene affected is the one that codes for the structural protein, fibrillin, associated with microfibrils in a number of connective tissue. You will remember that we mentioned Marfans in the Introduction to Pathology lecture and that Marfans Syndrome affects connective tissues throughout the body, including many organs such as the heart, eyes, aorta, skeleton and skin. People with Marfans Syndrome tend to be very tall, have very long limbs, arms and fingers. Eyes are affected in a number of ways which we mentioned in the Introduction lecture , so I recommend you go back to that lecture for information. Essentially, examples include that people with Marfans Syndrome are susceptible to lens dislocation because they have friable zonules due to defect in the fibrillin protein. They may have maldevelopment of the iris or increased axial length, making them predisposed to develop myopia. They are at risk of developing glaucoma. These are a few examples. - Familial Hypercholesteremia is an AD disorder due to a defect in the low density lipoprotein (LDL) receptor. This receptor is important for removal of LDL from the blood, however when there is a defect in the receptor, an accumulation of fat can lead to acceleration of atherosclerosis and the complications associated with it. Phenylketonuria is an AR disorder due to a defect in the enzyme, phenylalanine hydroxylase. This is an inborn error of metabolism and the consequent high circulating levels of phenylalanine due the defect in this enzyme can lead to mental retardation. This is why babies have a heel prick test at birth to test their blood for this, as this condition can be controlled by eliminating phenylalanine from the diet. - Lysosomal storage disease is one of the AR disorders in which a mutation occurs in one of the genes encoding the lysosomal hydrolases, important as it means that due to the defect, lysosomal efficiency is affected, and there is an accumulation of substrate within the lysosomes. - Neurofibromatosis is an AD disorder, as a result in a defect in neurofibromin 1 or 2 genes, which are tumour suppressor genes which are essentially negative regulators of growth. If there are defects in these genes, can lead to growths in the nervous system and skin. - Haemophilia is an X-linked recessive (XR) disorder that we mentioned earlier and is brought about by deficiencies in Factor VIII or IX, therefore people are deficient in these clotting factors have impaired blood clotting and are at risk of haemorrhaging. - Other disorders that you may have heard of due to point mutations are cystic fibrosis and sickle cell anaemia. **Slide 18:** **Mitochondrial Disease** Mitochondria have their own genome, known as mitochondrial DNA (mtDNA), it is circular DNA as apposed to the linear DNA in the nucleus. It contains 16569 DNA bases. Mitochondria has 37 genes that are all essential for normal mitochondrial function; which encode 13 proteins, 22 transfer RNAs and 2 ribosomal RNAs. The 13 mt genes that encode mitochondrial proteins, include those responsible for or involved in oxidative phosphorylation to create ATP for the cells source of energy. Mitochondria have proteins that are derived from both nDNA & mtDNA encoded genes. Important to note that mtDNA is more prone to mutation than nDNA, and that mitochondria disease is a consequence of either inherited or spontaneous mutations in nuclear or mitochondrial genes with consequent mitochondrial dysfunction. **Slide 19: Mitochondrial Disease** Mitochondrial disease usually refers to disorders caused by reduction in ATP production. Many individuals with mitochondrial disease display a cluster of symptoms that fall into a discrete syndrome such as Kearn's-Sayre Syndrome, which causes a person to lose full function of heart, eye and muscle movements. Considerable clinical variation exists making the disease difficult to diagnose & there is variable inheritance. Often there is a visual component to the disease. As you can see in image, health consequences of inherited mtDNA mutations vary widely. More frequently observed features include muscle weakness and wasting, problems with movement, diabetes, kidney failure, heart disease, dementia, loss of hearing and abnormalities involving the eyes and vision. Leber's hereditary optic neuropathy is a result in a mutation of four mitochondrial genes that provide instructions for making proteins that are part of the enzyme complex I, necessary for oxidative phosphorylation. It remains unclear why mutations are limited to the optic nerve in this disease. Other optic atrophies refer to a group of specific optic neuropathies which cause optic nerve dysfunction in either an AD or AR fashion. Autosomal dominant optic atrophy id the most common optic atrophy and has been associated with more than sixty mutations in the OPA1 gene. Its prevalence ranges from 1 in 50 to 1 in 10,000 and the optic neuropathy associated with this disease is characterised by loss of optic nerve tissue as a result of dramatic loss of retinal ganglion cell axons. Effect of mitochondrial disease on the eye includes ptosis (drooping eyelids), external ophthalmoplegia (inability to move eyes from side to side) and retinitis pigmentosa. **Slide 20: Mitochondria are maternally inherited** This is because on fertilisation the mitochondria in the male sperm are lost. It is difficult to predict how mtDNA is passed from mother to child due to the interplay between mitochondrial and nuclear genomes. It is important to remember that each cell has 1000s of copies of mtDNA which at birth are the same, known as homoplasmy. Individuals with mutated mtDNA have a mixture of mutated and non-mutated mtDNA within a particicular cells and this is called as heteroplasmy. This is the reason why a mother with mild or no symptoms can have a child with different levels of presentation of disease. For heteroplasmic mutations of disease a cell can compensate for reduced normal mtDNA up to a certain threshold. However, even if a heteroplasmy is subthreshold for disease in a mother, disease may occur in the offspring depending on how the mitochondria are apportioned in her oocytes during gametogenesis. If there are low levels of mutation, the offspring will not present with disease; if there is an intermediate level of mutation in the mtDNA, the offspring will likely present with mild disease and if there is high level of mutation in mtDNA, it highly likely that the offspring will present with severe disease. **Slide 21: Basic Genetics & Genetic Disease** In third part of this topic we will look at chromosomal abnormalities and how they can lead to disease, before touching upon multifactorial disease and teratology. **Slide 22: Somatic cells contain chromosomes** In first part of this topic, we said that our chromosomes within our somatic cells, store our genetic information in the form of long strands of DNA wrapped around positively charged histones. Within our somatic cells, we have we have 2 sets of paired homologous chromosomes, which makes 46 chromosomes: 22 of which are autosome pairs and 2 of which are the sex chromosomes which can be XX or XY. **Slide 23: Chromosomal Abnormalities** 45% still births are accounted for by chromosomal abnormalities. Chromosomal abnormalities are more common than gene abnormalities, affecting 4% human foetus, 90% of these spontaneously abort in early weeks of pregnancy as a result of trisomies (50% of these) and 18% of these are a result of Turners Syndrome. Incidence of detectable abnormalities are therefore only about 0.7%, with 40% due to sex chromosomes abnormalities and 20% due to autosomal trisomies, and the remaining due to the rearrangement of chromosome parts as we will see later. **Slide 24: Chromosomal Disorders** Are caused by either abnormal structure or an abnormal number of chromosomes. Let's remind ourselves of abnormal chromosome structure. Chromosomes have a p arm and q arm, with constriction in the middle known as a centromere. Depending on the position of that centromere, they are called metacentric, submetacentric, or if the constriction is near the top of the chromosome, the chromosome is called an acrocentric chromosome. **Slide 25: Structural Abnormalities** Structural abnormalities occur as a result of chromosomal breakage which occur randomly, although some sites are more susceptible to breakage than others. Rate of chromosome breakage can be increased by irradiation or chemicals. So structural abnormalities occur due to exchange of genetic material, which when it occurs between non-homologous chromosomes is called translocation, deletion of part or all of a chromosome or rearrangement of parts of a chromosome. **Slide 26: Structural Abnormalities** - [Deletion]: involves breaks at ends of chromosomes, some of the genetic information is lost and results in a shortened chromosome - [Inversion]: 2 breaks on a single chromosome on opposite sides of centromere, if the breaks are on same arm of a chromosome it is a paracentric inversion, if on different arms it is called a pericentric inversion. - [Ring formation] describes when you get breaks at each telomeric end of the chromosome, deletion of acrocentric parts ie ends of chromosome occurs, and the remaining chromosome fuses end-to-end to form a ring shaped chromosome. **Slide 27: Structural Abnormalities** - [Translocation:] exchange of genetic material between two non-homologous chromosomes due to breaks; if the exchange is reciprocal, this is a balanced translocation. If one chromosome donates genetic information, but does not receive any DNA, this is known as a non-balanced translocation. - [Robertsonian Translocation:] a break occurs near the centromere in 2 acrocentric chromosomes such that the p arms are lost and the 2 remaining q arms fuse to form a single chromosome. **Slide 28: Numeric Chromosome Abnormalities** Numeric chromosome abnormalities can also exist: - Where there is not an exact multiple of a haploid set of the 23 chromosome pairs, this is known as aneuploidy - multiples of 23 chromosome pairs is called polyploidy - if there is loss of a single chromosome, this is called monosomy - if there is an extra copy of a single chromosome, this is known as trisomy These numeric chromosome abnormalities are primarily due to non-disjunction, which is unequal sharing of chromosomes at anaphase. **Slide 29: Non-disjunction** LHS: normal process of meiosis that results in fertilisation of diploid cells. However if non-disjunction (RHS) occurs, the two diploid cells enter Anaphase II, however the sister chromatids fail to separate or there is a delayed movement prior to cell division. The consequence of this is that you do not get full separation of the chromatids, so you have one absent which generates a cell with an additional chromosome, known as trisomy and a cell with a missing chromosome, known as monosomy. **Slide 30: Chromosomal Disorders** There are a number of chromosomal disorders. These include disorders affecting autosomal chromosomes: - Down Syndrome or trisomy 21: so-called because there is an additional chromosome 21. So for a female the karyotype is 47 XX +21 and for a male, the karyotype is 47 XY +21 - Edwards Syndrome has an additional Chromosome 18, so also known as trisomy 18. Therefore the karyotype is 47 XX+18 (females) or 47 XY +18 (males). - In Patau Syndrome, a trisomy 13, as the additional chromosome is Chromosome 13, so the karyotype is 47 XX/XY +13 - Cri-du-chat syndrome represents an abnormality in structure of the chromosome. People with this syndrome have 46 chromosomes, but have a deletion on the p arm of chromosome 5, hence the karyotype in males is 46XY del (5p) Sex chromosomes can also be affected, as in the case of: - Klinefelter Syndrome in males: have an additional X, so the karyotype is 47XXY - Turner Syndrome in females: has a missing X, so the karyotype is 45XO **Slide 31: Trisomy 21 -- Downs Syndrome** A little more detail on trisomy 21, the trisomy occurs due to nondisjunction. Morphologic abnormalities associated include slanted eyes, epicanthal folds, dysplastic tongue, protruding tongue. People with trisomy 21 may suffer from congenital heart disease or may have varying degrees of mental retardation. Incidence increases with increasing maternal age, with 1 in 1000 live births in the mothers in the mid-30s, and a dramatic increase to 1 in 30 live births affected with a maternal age of 45 years. **Slide 32: Trisomy 21: karyotype** Here we see the chromosomes of a person with trisomy 21, to give the karyotype of a male with an additional chromosome 21, so the karyotype is 47 XY +21. **Slide 33: Turners Syndrome (45, X)** In Turners syndrome, there can be complete or partial monosomy, where one X chromosome in females is completely lacking, although in 50% of cases, there may be an structural abnormality of one X chromosome. The karyotype here (image) is 45 X as an X chromosome is missing. People with may suffer from cardiovascular anomalies. They are born with fully developed ovaries containing oocytes but as the person matures, they will develop fibrous ovaries that lack oocytes, making people sterile. Females are usually less that 5 feet tall, with a short webbed neck, however if treated with growth hormone and oestrogen they can live a relatively normal life. **Slide 34:** **Multifactorial disease** Next, we will move on to multifactorial disease. In multifactorial disease, the disorders do not follow an inherited Mendelian fashion, because the diseases are caused by the additive effects of a number of abnormal genes and/or the effect of environmental factors. Common chronic disorders include diabetes (that we have talked about previously), atherosclerosis, arthritis, cancer and hypertension. Birth defects that may arise include cleft lip/palate, congenital heart disease **Slide 35: Teratology** Teratology is the study of congenital malformation which is where morphological defects present at birth. It is a major cause of illness, representing 1.6% of total births. It tends to follow multifactorial inheritance as a number of factors may be involved including a single gene combined with environmental factors or several genes and environmental factors. Teratogens are factors that cause congenital malformation, include viruses eg rubella, irradiation such as X-rays, β or γ radiation, or chemicals such as cytotoxic drugs, excess Vit A or pollutants. Thalidomide, many of you may have heard of, was prescribed in the 1960s to combat morning sickness and to aid sleep. However, the consequence of females taking this drug during pregnancy was severe congenital malformation. **Slide 36: Principles of Teratology** If we look at principles of teratology: - Susceptibility to a particular teratogen is variable, some children may be affected, others may not. - Susceptibility to a particular teratogen is specific to the developmental stage of the embryo, for instance rubella will only affect the foetus during the first 3 months of development. In the image, we can see at which stage of development a particular part of the body is at maximal sensitivity to the development of morphological abnormalities if it is exposed to a teratogen during those times. - The mechanism of injury is also specific to the teratogen, for example, the organ or mechanism in the body that is affected is dependent on the particular teratogen that the body is exposed to. - Teratogenesis is dose-dependent, meaning that the level of dose will determine the level of malformation i.e. from extreme consequences such as death, growth retardation, malformation to minimal functional impairment. **Slide 37: Errors of Morphogenesis** Normal intrauterine and postnatal development occurs as a consequence of sequential activation and repression of genes inherited from parents, and these genes are activated in a stage specific manner within the zygote. Any abnormal activation or structurally abnormal genes in the zygote or the early embryonic cells will lead to early death during development. Most major abnormalities arise due faulty gene activity or the effect of exogenous factors or toxins, such as teratogens, on the embryo during the formation of primordial organs. In the last slide, we looked at the stages at which various organs had maximal sensitivity to teratogens, and this is usually within the first 8 weeks of pregnancy. **Slide 38: Errors of Morphogenesis** Clinically important malformations that occur as a consequence of errors of morphogenesis include neural tube defects, such as anencephaly, spina bifida and meningocele - Anencephaly: severe neural tube defect arising as a consequence of reduced size or lack of cerebral hemispheres. - Spina bifida: arises due to incomplete closure of the vertebral column, leaving spinal cord exposed. - Meningocele: arises due to protrusion of meninges through the vertebral column - Fetal alcohol syndrome is a complex of abnormalities that arises due to maternal alcohol consumption. However, only in the case of extreme and chronic alcoholism does growth retardation, dysfunction of the central nervous system and facial defects arise. Often children suffer from mild degrees of mental deficiencies and emotional disorders. - **TORCH** Complex describes a group of diseases or neonatal/foetal infections produced by a wide variety of micro-organisms, but give rise to similar signs and symptoms (see image). **T**oxoplasmosis affects about 0.1% of pregnancies. **O**ther micro-organisms that produce similar signs and symptoms **R**ubella, since the introduction of the vaccine, affects \