Gas Transfer Notes PDF
Document Details
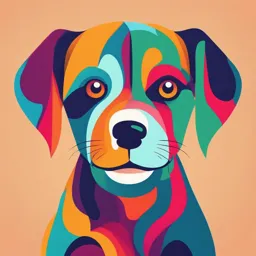
Uploaded by FruitfulIntegral
WSUSOM
Tags
Summary
These notes cover gas transfer in medical physiology, including learning objectives, a lecture outline, and discussions of oxygen and carbon dioxide transport. They provide a detailed explanation of the process, referencing Henry's law and the oxyhemoglobin dissociation curve.
Full Transcript
WSUSOM Medical Physiology – Respiratory Physiology Page | 1 of 4 Gas Transfer GAS TRANSFER Learning Objectives 1. Define oxygen partial pressure (tension), oxygen content, and percent hemoglobin saturation as they perta...
WSUSOM Medical Physiology – Respiratory Physiology Page | 1 of 4 Gas Transfer GAS TRANSFER Learning Objectives 1. Define oxygen partial pressure (tension), oxygen content, and percent hemoglobin saturation as they pertain to blood. 2. Draw an oxyhemoglobin dissociation curve (hemoglobin oxygen equilibrium curve) showing the relationships between oxygen partial pressure, hemoglobin saturation, and blood oxygen content. On the same axes, draw the relationship between PO2 and dissolved plasma O2 content (Henry’s Law). Compare the relative amounts of O2 carried bound to hemoglobin with that carried in the dissolved form. Describe how the shape of the oxyhemoglobin dissociation curve influences the uptake and delivery of oxygen. Define P50. 3. Show how the oxyhemoglobin dissociation curve is affected by changes in blood temperature, pH, PCO2, and 2,3-DPG and describe a situation where such changes have important physiological consequences. Describe how anemia and carbon monoxide poisoning affect the shape of the oxyhemoglobin dissociation curve, PaO2, and SaO2. 4. List the forms in which carbon dioxide is carried in the blood. Identify the percentage of total CO2 transported as each form. 5. Identify the enzyme that is essential to normal carbon dioxide transport by the blood and its location. 6. Describe the interplay between CO2 and O2 binding on hemoglobin that causes the Haldane effect. Describe the importance of the chloride shift in the transport of CO2 by the blood. Lecture Outline I. Oxygen Transport Role of plasma in oxygen transport Role of hemoglobin in oxygen transport II. Oxyhemoglobin dissociation curve A. Factors that influence the oxyhemoglobin dissociation curve Temperature DPG concentration acid-base balance carbon monoxide III. Carbon Dioxide Transport Role of plasma in carbon dioxide transport Role of hemoglobin in carbon dioxide transport Role of bicarbonate in carbon dioxide transport IV. Acid-Base Balance Kassirer-Bleich Equation Respiratory and metabolic acidosis Respiratory and metabolic alkalosis WSUSOM Medical Physiology – Respiratory Physiology Page | 2 of 4 Gas Transfer Oxygen Transport Once oxygen diffuses into the pulmonary capillaries from the alveoli, it is transported to the tissue in order to produce energy. Oxygen is carried in two forms: dissolved in plasma combined with hemoglobin Dissolved in plasma - Henry’s law states that the amount of dissolved gas is proportional to the partial pressure of that gas. For each mmHg of partial pressure of oxygen (PO2), there will be 0.003 ml of O2/100 ml of blood. Therefore, at a normal PO2 of 100 mmHg only 0.3 ml of O2 will be dissolved in the blood and transferred to the tissue. The normal oxygen content of blood is 20 ml/100 ml of blood. Therefore, another method of transporting oxygen to the blood must be present. Combined with hemoglobin - Each hemoglobin (Hb) molecule is capable of binding 4 molecules of oxygen. When a Hb molecule binds 4 oxygen molecules than the molecule is said to be fully saturated. When fully saturated, each gram of Hb can bind 1.34 ml of oxygen. Therefore, the oxygen content of hemoglobin equals the hemoglobin concentration multiplied by 1.34 multiplied by the degree of saturation of hemoglobin with oxygen. Example: Hb content in women is 14 grams. In men it is 16 grams. O2 content = 16 g/100 ml * 1.34 ml O2/g * 1.0 = 21.44 mlO2/100 ml of blood WSUSOM Medical Physiology – Respiratory Physiology Page | 3 of 4 Gas Transfer Oxyhemoglobin Dissociation Curve The relationship between PO2 in arterial blood and the degree of hemoglobin saturated is shown in the figures above. Note that the relationship between PO2 and % saturation is curvilinear (top graph). This is important because it shows that hemoglobin will remain close to 100 % saturated even though the PO2 decreases significantly from 100 mmHg to approximately 60 mmHg. It is only after PO2 has decreased significantly (i.e. lower than 60 mmHg) that significant reductions in oxygen saturation occur. There are a number of variables (bottom graphs) that are capable of eliciting a right-ward shift in the PO2 - % saturation curve. The implications of this shift are for a given value of PO2 less hemoglobin is saturated. Thus, any condition that results in an increase in temperature, 2,3 diphosphoglycerate, partial pressure of carbon dioxide (PCO2) or hydrogen ion concentration, will decrease the affinity of hemoglobin for oxygen. For example, carbon dioxide, which is a by-product of metabolism, is released at the tissue level. This increase in carbon dioxide will decrease the affinity of hemoglobin for oxygen, which allows oxygen to diffuse into the tissue to be utilized during aerobic metabolism (i.e. Bohr effect). Increases in temperature and hydrogen ion concentration often occur under conditions of extreme exercise and thus these alterations would also promote the release of oxygen at the tissue level. Lastly, carbon monoxide competes for oxygen binding sites on hemoglobin (see graph labelled carbon monoxide poisoning). The affinity of hemoglobin for carbon monoxide is 200 times its affinity for oxygen. Carbon monoxide occupies oxygen binding sites on hemoglobin leading to a decrease in oxygen content. Binding of carbon monoxide to hemoglobin increases the affinity of the remaining sites for oxygen causing the curve to shift to the left. WSUSOM Medical Physiology – Respiratory Physiology Page | 4 of 4 Gas Transfer Carbon Dioxide Transport Carbon dioxide is transported in three forms: Approximately 6 – 7 % of CO2 is transported in the dissolved form. Approximately 90 % in arterial blood and 70 % in venous blood is transported in the form of bicarbonate ions Approximately 4 % in arterial blood and 23 % in venous blood is transported in the form of a carbamino compound. At the tissue (top figure) CO2 diffuses from the tissue into the blood plasma where it is dissolved. Some CO2 will also combine with water to form carbonic acid and ultimately bicarbonate and hydrogen ions. The chemical reaction is slow because of the absence of carbonic anhydrase. The hydrogen ions produced are buffered by plasma proteins. Carbon dioxide will also enter hemoglobin where it binds reversibly to the amino terminus of alpha and beta chains of hemoglobin (carbamino compound). The remainder of CO2 is carried as bicarbonate (i.e. CO2 + H2O H2CO3 H+ + HCO3-). Note the series of interactions that take place regarding transport of CO2 in the form of bicarbonate. First, CO2 binds with water to form carbonic acid. The reaction occurs quickly because of the presence of carbonic anhydrase. Once carbonic acid dissociates the hydrogen ions that are produced are buffered by hemoglobin. Consequently, the pH of red blood cells remains constant. The bicarbonate produced is exchanged with chloride and transported thereafter. At the level of the lung the reactions that occur are simply the reverse of those described for the tissue level. In a healthy individual, arterial and alveolar PCO2 are virtually identical. Arterial PCO2 is a balance between CO2 production and elimination. Mixed venous CO2 content is a balance between CO2 production, CO2 delivery and the CO2equilibrium curve.