Biochemical Identification PDF
Document Details
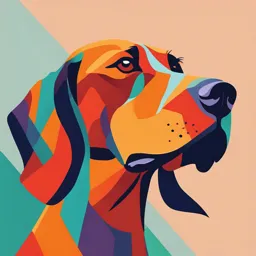
Uploaded by IndividualizedBarbizonSchool
Tags
Summary
This document provides a comprehensive overview of biochemical identification methods for bacteria, including various tests, procedures, and interpretations. It explains the traditional and newer methodologies used in clinical bacteriology.
Full Transcript
Biochemical Identification Clinical Bacteriology (Lecture & Laboratory) Introduction Traditionally, bacterial identification has relied on three main factors: 1. Colony Morphology: Characteristics such as shape, size, color, and hemolytic properties observed on growth media. 2. Mi...
Biochemical Identification Clinical Bacteriology (Lecture & Laboratory) Introduction Traditionally, bacterial identification has relied on three main factors: 1. Colony Morphology: Characteristics such as shape, size, color, and hemolytic properties observed on growth media. 2. Microscopic Morphology: Gram-stained smears examined under a microscope to distinguish Gram-positive from Gram-negative bacteria. 3. Biochemical Testing: Detects the metabolic and enzymatic activities of bacteria to provide further identification. Biochemical Testing: Evolution and Technique Initially, biochemical tests for bacterial identification were carried out in test tubes. Each test detected a specific metabolic activity, such as fermentation of sugars, enzyme production, or gas production. Multitest systems were developed, allowing multiple biochemical reactions to be tested simultaneously in a compact format. Automated systems emerged, further improving efficiency. These computerized systems interpret biochemical reactions and cross- reference the results against databases to rapidly identify the organism and sometimes provide antimicrobial susceptibility data. Serotyping and Other Phenotypic Methods Serotyping is sometimes used to identify different strains of bacteria within a species. For example, certain Gram-negative bacteria like Escherichia coli and Salmonella are classified based on their O and H antigens. Fatty acid analysis using gas-liquid chromatography (GLC), which characterizes the fatty acid composition of the bacterial cell wall to aid in identification. MALDI-TOF and Molecular Techniques Matrix-assisted laser desorption/ionization-time-of-flight (MALDI-TOF) mass spectrometry, a rapid technique that identifies microorganisms based on their unique protein profiles. Molecular techniques based on the genotype of the organism, such as nucleic acid assays, are employed. These methods, which detect specific DNA or RNA sequences, offer high sensitivity and specificity. Techniques such as polymerase chain reaction (PCR) and sequencing are becoming integral to bacterial identification, particularly in complex or difficult-to-identify cases. Carbohydrate Utilization Test This test differentiates between lactose fermenters and non- lactose fermenters, which is key in distinguishing many Gram- negative bacteria, such as members of the Enterobacteriaceae family. Lactose Degradation Process Lactose, a disaccharide composed of glucose and galactose, requires two enzymes for its metabolism β-Galactoside Permease: A transport enzyme that facilitates lactose entry into the bacterial cell. β-Galactosidase: An enzyme that cleaves lactose into its monosaccharide components—glucose and galactose. Lactose fermenters possess both enzymes, allowing them to metabolize lactose effectively. Non-lactose fermenters lack either one or both enzymes and cannot process lactose. Delayed lactose fermenters possess β-galactosidase but lack β- galactoside permease. These bacteria eventually ferment lactose but at a slower rate. Metabolic Pathways for Glucose Utilization Once lactose is hydrolyzed, glucose becomes available for energy production through one of the following pathways: 1. Embden-Meyerhof-Parnas (EMP) Pathway (Glycolysis): This is the most common pathway where glucose is broken down to generate energy. 2. Entner-Doudoroff Pathway: An alternative glycolytic pathway utilized by certain bacterial species, such as Pseudomonas aeruginosa. If a bacterium can ferment glucose, it can often also ferment lactose, but some species can metabolize glucose without utilizing lactose. Other Carbohydrates Bacteria can also utilize a variety of other carbohydrates, including monosaccharides (e.g., glucose, fructose), disaccharides (e.g., sucrose, maltose), and polysaccharides (e.g., starch). Other carbohydrates include polyhydric alcohols such as adonitol, dulcitol, mannitol, and sorbitol. Some bacterial species are asaccharolytic, meaning they are unable to ferment carbohydrates and instead utilize other organic molecules such as amino acids as their energy and carbon sources. Oxidation-Fermentation (O/F) Test The oxidation-fermentation (O/F) test is a biochemical method used to determine how bacteria metabolize carbohydrates, whether by oxidation (aerobic respiration) or fermentation (anaerobic respiration), or both. This test is particularly important in the identification of Gram-negative bacilli, distinguishing glucose fermenters like the Enterobacterales from aerobic nonfermenters like Pseudomonads. Carbohydrate Fermentation In fermentation, bacteria convert carbohydrates into acid (or acid and gas) in an anaerobic environment. This process begins with glycolysis, where glucose is broken down into pyruvic acid, which is then further reduced to acids. The end products of fermentation are acidic, and gas may also be produced. Homolactic acid fermenters (e.g., some streptococci) produce primarily lactic acid. Mixed acid fermenters (e.g., some Enterobacterales) produce various acids, such as formic, lactic, acetic, and succinic acids. A pH indicator in the medium changes color in response to the acid production, allowing detection. Carbohydrate Oxidation In oxidation, which also starts with glycolysis, pyruvic acid is further oxidized into carbon dioxide (CO₂). Oxidation requires oxygen or an alternative terminal electron acceptor, such as nitrate (NO₃⁻), in anaerobic conditions. Oxidation typically produces less acid and more energy than fermentation. Weak Acid Production and Peptone Utilization Oxidizers and fastidious fermenters often produce weak acids, which are difficult to detect in media containing high peptone concentrations, such as triple sugar iron (TSI) agar. Peptones generate alkaline byproducts, which can neutralize the small amounts of acid produced. To better detect weak acids, the Hugh-Leifson oxidation- fermentation basal medium (OFBM) is used. This medium contains: 1% carbohydrates (same as TSI) for acid production 0.2% peptones to minimize alkaline byproducts Bromothymol blue as a pH indicator (green when neutral, yellow when acidic, blue when alkaline) Procedure 1. Inoculate two tubes of OF test medium with the test organism using a straight wire by stabbing “halfway to the bottom” of the tube. Aerobic tube (open): Left uncovered to allow oxygen access. Anaerobic tube (closed): Covered with sterile mineral oil to create an anaerobic environment. 2. Incubate both tubes at 35°C for 48 hours (slow-growing bacteria may take 3 to 4 days before results can be observed) Interpreting O/F Test Results Fermenter: Acid production in both the open (aerobic) and closed (anaerobic) tubes indicates that the organism can ferment carbohydrates both aerobically and anaerobically. Streptococci, for example, generate energy exclusively by fermentation, even in the presence of oxygen. Oxidizer: Acid production in the open tube only suggests that the organism uses carbohydrates by oxidation. This is typical for aerobic organisms like Pseudomonas species. Nonoxidizer: If no acid is produced in the open tube, the organism may not oxidize carbohydrates. Nonoxidizers may instead utilize peptones, creating an alkaline environment, leading to a blue color in the open tube. Interpreting O/F Test Results Triple Sugar Iron (TSI) Agar Triple Sugar Iron (TSI) agar and Kligler Iron Agar (KIA) are key tools in the presumptive identification of Gram-negative enteric bacteria, particularly when screening for intestinal pathogens. The difference between TSI and KIA lies in their sugar composition: TSI contains glucose, lactose, and sucrose, whereas KIA contains only glucose and lactose. Both agars are useful for testing the ability of bacteria to ferment sugars, produce gas during fermentation, and produce hydrogen sulfide gas (H₂S). Triple Sugar Iron (TSI) Agar Composition of TSI Agar Carbohydrates: Glucose (0.1%) Lactose (1%) Sucrose (1%) Indicators: Phenol red (pH indicator): Yellow below pH 6.8 (acidic); red above pH 7.4 (alkaline). Ferrous sulfate and sodium thiosulfate: To detect H₂S production. Procedure 1. Using an inoculating needle, stab the butt of the agar (anaerobic portion) and then streak the slant (aerobic portion). 2. Incubate the tube with a loosely capped lid to allow oxygen to enter. 3. Incubation is typically done at non-CO₂ conditions for 18 to 24 hours. 4. Results are written as slant reaction/butt reaction (e.g., K/A, A/A, etc.). Interpreting TSI Agar Reactions No Fermentation: Reaction: K/K or K/NC (alkaline slant/alkaline butt or no change in butt). Meaning: Organism does not ferment glucose or lactose and instead degrades peptones, leading to an alkaline reaction (deep red color) in both the slant and butt. Typical organisms: Non-fermenters (e.g., Pseudomonas). Interpreting TSI Agar Reactions Glucose Fermentation Only: Reaction: K/A (alkaline slant/acid butt). Meaning: The organism ferments glucose, producing enough acid to turn the medium yellow initially. After 12 hours, glucose is consumed, and the bacteria on the slant utilize peptones aerobically, reverting to alkaline. The butt remains acidic due to anaerobic fermentation. Caution: Reading results too early may falsely suggest lactose or sucrose fermentation. Interpreting TSI Agar Reactions Lactose and/or Sucrose Fermentation: Reaction: A/A (acid slant/acid butt). Meaning: The organism ferments glucose first, then lactose or sucrose, producing enough acid to keep both the slant and butt yellow at the end of 18-24 hours. Note: If incubated beyond 24 hours, lactose or sucrose may be depleted, leading to peptone utilization and an alkaline slant Interpreting TSI Agar Reactions H₂S Production: Reaction: K/A, H₂S or A/A, H₂S. Meaning: H₂S is produced in an acidic environment, combining with ferric ions to form black precipitate(ferrous sulfide) in the butt. Even if the yellow color is obscured by black precipitate, glucose fermentation is assumed since H₂S formation requires acid. Process: Bacterium + sodium thiosulfate → H₂S gas. H₂S + ferric ions → ferrous sulfide (black precipitate). Interpreting TSI Agar Reactions Gas Production: Reaction: Presence of bubbles, cracks, or splitting in the agar, or complete displacement of the medium in the butt. Meaning: Gas formation occurs during carbohydrate fermentation, indicating an aerogenic organism (producing gas). Interpreting TSI Agar Reactions Interpreting TSI Agar Reactions Reaction Interpretation Example Organisms K/K or K/NC No fermentation Pseudomonas aeruginosa K/A Glucose fermentation only Shigella, Salmonella A/A Lactose and/or sucrose fermentation Escherichia coli, Klebsiella K/A H2S Glucose fermentation, H2S production Salmonella A/A H2S Lactose/sucrose fermentation, H2S Proteus spp. production Gas Aerogenic organisms Enterobacter, E. coli Ortho-nitrophenyl-β-D-galactopyranoside (ONPG) Test The ortho-nitrophenyl-β-D-galactopyranoside (ONPG) test is used to determine if an organism is a delayed lactose fermenter or a true non-lactose fermenter. This test is particularly important because some bacteria initially appear as non-lactose fermenters on primary isolation media, but they may possess the enzyme β-galactosidase without having β- galactoside permease. Key Points of the ONPG Test Delayed vs. True Non-Lactose Fermenters Delayed lactose fermenters lack β-galactoside permease, the enzyme responsible for transporting lactose across the bacterial cell membrane, but they have β-galactosidase, which can break down lactose into glucose and galactose. True non-lactose fermenters lack both β-galactoside permease and β- galactosidase. ONPG Structure ONPG is structurally similar to lactose but does not require β-galactoside permease to enter the bacterial cell. Once inside, β-galactosidase hydrolyzes ONPG into o-nitrophenol (yellow color) and galactose. Key Points of the ONPG Test Interpretation Positive test: The organism possesses β- galactosidase and turns the ONPG solution yellow (indicating delayed lactose fermentation). Negative test: The ONPG solution remains colorless, indicating a true non-lactose fermenter. Test Procedure A heavy suspension of the bacteria is prepared in sterile saline. ONPG disks or tablets are added to the suspension, which is incubated at 35°C. Positive results are typically seen within 6 hours. Key Points of the ONPG Test Inducible Enzyme β-galactosidase is inducible, meaning it is only produced when lactose or a similar substrate is present. Therefore, organisms must be taken from lactose-containing media for the test. Considerations Bacteria producing a yellow pigment should not be tested using ONPG, as this could result in a false-positive result. Glucose Metabolism and its Metabolic Product Glucose metabolism in bacteria can proceed through various pathways, with two major ones being the mixed acid fermentation pathway and the butylene glycol pathway. These pathways produce different end products, which can be detected using the Methyl Red (MR) and Voges-Proskauer (VP) tests. Methyl Red (MR) Test The MR test identifies organisms that produce stable acid end products from glucose metabolism via the mixed acid fermentation pathway. Procedure Bacteria are incubated in a broth medium containing glucose for 3 to 5 days at 35°C. After incubation, a pH indicator (methyl red) is added to detect acid production. Interpretation Positive result: If the organism uses the mixed acid fermentation pathway, stable acids lower the pH to around 4.4, turning the indicator red. Negative result: If the organism does not produce stable acids, the pH remains higher (around 6.0), and the broth stays yellow. Reaction Pathway Voges-Proskauer (VP) Test The VP test detects organisms that metabolize glucose via the butylene glycol pathway, leading to the production of acetoin and eventually 2,3-butanediol. Procedure After incubation in glucose-containing medium, α-naphthol (a color intensifier) and 40% potassium hydroxide (KOH) or sodium hydroxide (NaOH) are added. The tube is shaken to enhance oxygenation, allowing acetoin to oxidize to diacetyl. Diacetyl reacts with KOH and α-naphthol, forming a red complex. Interpretation Positive result: Formation of the red complex indicates the presence of acetoin and confirms glucose metabolism via the butylene glycol pathway. Negative result: The absence of a red complex indicates no acetoin production, thus a negative VP result. Reaction Pathway Key Points of the MRVP Test Most commercial methyl red–Voges-Proskauer (MRVP) media are a modification of Clark and Lubs medium. Bacteria are generally either MR-positive or VP-positive, but not both, due to the different metabolic pathways they use. Some bacteria may be negative for both tests, indicating they do not use either pathway to metabolize glucose. Amino Acid Utilization Bacteria can utilize amino acids as sources of energy and carbon. Various tests, such as decarboxylase and deaminase tests, help identify specific bacterial capabilities in amino acid metabolism. Decarboxylase Test The decarboxylase test assesses whether bacteria can remove the carboxyl group (COOH) from specific amino acids, producing alkaline by-products. Commonly tested amino acids include lysine, ornithine, and arginine. The resulting products of decarboxylation are amines or diamines and CO₂, leading to an increase in the medium's pH. Decarboxylation Pathways Lysine → Lysine decarboxylase → Cadaverine (amine) + CO₂ Ornithine → Ornithine decarboxylase → Putrescine (diamine) + CO₂ Arginine → Arginine dihydrolase → Citrulline → Ornithine → Putrescine Mechanism Lysine is decarboxylated to cadaverine. Ornithine is converted to putrescine. Arginine is decarboxylated to agmatine, which is further broken down into putrescine and urea. If the organism also produces urease, urea is degraded to ammonia (NH₃) and CO₂. Arginine can also be broken down via the arginine dihydrolase pathway to produce citrulline, which is then cleaved to form ornithine, which may be decarboxylated to putrescine. Testing Moeller decarboxylase base medium is used, containing glucose, peptones, bromocresol purple, and cresol red as pH indicators. It starts at a pH of 6.0. The medium turns yellow due to glucose fermentation (pH 5.5). If the bacteria produce the specific decarboxylase, the medium turns purple, indicating an alkaline shift. Anaerobic conditions are achieved by overlaying the medium with mineral oil. The test is considered positive if the medium turns purple after incubation at 35°C. A control tube without the amino acid remains yellow. Testing Results can usually be recorded in 24hours; however, bacteria with weak decarboxylase activity may take 4 days to be positive. Modifications of the decarboxylase test to detect other biochemical reactions are also used. Examples of these include the MIO and LIA tests Deaminase Test The deaminase test detects whether bacteria possess deaminases, which remove an amine group (NH₂) from amino acids. Phenylalanine Deaminase (PAD) Test: The PAD test determines if bacteria can convert phenylalanine into phenylpyruvic acid. The test medium contains 0.2% phenylalanine on an agar slant. After incubation, 10% ferric chloride (FeCl₃) is added. If phenylpyruvic acid is present, a green color develops, indicating a positive result. This test differentiates Proteus, Morganella, and Providencia species (which are PAD positive) from other Enterobacterales. Deaminase Test Reaction Phenylalanine → Phenylalanine deaminase → Phenylpyruvic acid+FeCl₃ (green color) Positive decarboxylase tests show a purple color, while negative tests show yellow. The PAD test is useful for distinguishing certain species of enteric bacteria by detecting their ability to deaminate phenylalanine. Citrate Utilization Test Citrate Utilization Test: The citrate test determines if bacteria can use sodium citrate as their sole carbon source. The commonly used medium is Simmons' citrate agar, which also contains ammonium salts as the nitrogen source and bromothymol blue as the pH indicator. When bacteria metabolize citrate, they also use the ammonium salts, producing ammonia, which increases the pH, turning the medium from green to blue. Citrate Utilization Test Procedure A light inoculum is important to avoid false-positive results from dead organisms that can serve as a carbon source. Incubation is typically done at 35°C. Positive result: Blue color (alkaline pH). Negative result: Green color (no citrate utilization). Alternative method: Christensen’s citrate medium uses phenol red as the pH indicator. A positive result turns the medium pink at an alkaline pH. Deoxyribonuclease (DNase) Production Test The DNase test checks if bacteria produce deoxyribonucleases (DNases), which break down DNA into smaller subunits. The test medium contains 0.2% DNA, and bacterial growth is streaked on the medium surface. Procedure The plate is incubated at 35°C for 18-24 hours. After incubation, 1 N HCl is added to precipitate unhydrolyzed DNA. If DNase is present, it hydrolyzes the DNA, leaving a clear zone (halo) around the bacterial growth, indicating a positive result. Methyl green can also be used in the medium. It binds to intact DNA, forming a green color. If the DNA is hydrolyzed, the color fades, and a clear zone indicates a positive result. Positive result: Clear halo around the bacteria. Negative result: No clear halo, the medium remains opaque. Indole Production Test The indole test identifies bacteria that can degrade tryptophan into indole, pyruvic acid, and ammonia using the enzyme tryptophanase. Procedure Bacteria are inoculated into tryptophan or peptone broth and incubated at 35°C for 48 hours. The test can be performed using either the Ehrlich or Kovac's method: Ehrlich method: After incubation, xylene is added to extract indole from the broth. After shaking, Ehrlich’s reagent (p- dimethylaminobenzaldehyde or PDAB) is added. A red color indicates the presence of indole. Indole Production Test Kovac’s method: Kovac’s reagent (which also contains PDAB) is added directly to the broth. A red color indicates a positive result. Kovac’s method does not involve xylene extraction and is less sensitive than the Ehrlich method. For a rapid indole test, isolated bacterial colonies are smeared onto filter paper moistened with p- dimethylaminocinnamaldehyde. A blue-green color within 2 minutes indicates indole production. Positive result: Red color (Ehrlich or Kovac’s method) or blue-green color (rapid test). Negative result: No color change. Lysine Iron Agar (LIA) Slant The LIA test is used to detect the ability of bacteria to decarboxylate or deaminate lysine and to produce H₂S. The medium contains: Lysine (amino acid) Glucose Ferric ammonium citrate (for detecting H₂S) Sodium thiosulfate (for H₂S detection) Bromocresol purple (pH indicator) Lysine Iron Agar (LIA) Slant Procedure Inoculation is done like a triple sugar iron (TSI) slant, using a straight inoculating needle to stab the butt and streak the slant. Lysine decarboxylation occurs anaerobically (in the butt), producing cadaverine and leading to a purple butt (positive result). Lysine deamination (on the slant) results in a reddish- purple slant. H₂S production results in a black precipitate, which also indicates a positive result for decarboxylation, as H₂S is formed only in an alkaline environment. Results Purple butt: Positive for lysine decarboxylation. Yellow butt: Negative for lysine decarboxylation (due to glucose fermentation). Red slant: Positive for lysine deamination. Black precipitate: Positive for H₂S production. Motility Test Motility is determined by bacterial movement in a semi-solid medium. Motility test media have a low agar concentration (0.4% or less) to allow bacteria to move. Procedure Make a straight stab with an inoculating needle into the center of the semi-solid medium. After incubation, motility is indicated by growth spreading away from the stab line or a hazy appearance throughout the medium. Temperature: Some organisms are motile only at room temperature, so it’s recommended to incubate one tube at room temperature and another at 35°C. Results Motile: Growth radiates from the stab line or is spread throughout the medium. Non-motile: Growth remains confined to the stab line. Motility-Indole-Ornithine (MIO) Agar MIO agar is used to detect motility, indole production, and ornithine decarboxylase activity. This medium is particularly useful for differentiating Klebsiella from Enterobacter and Serrati a. Procedure The medium is inoculated by stabbing straight down the center. Motility is indicated by diffuse growth or clouding of the medium. Ornithine decarboxylation results in a purple medium. Indole production is detected by adding Kovac’s reagent, which turns red if indole is present. Results Purple color: Positive for ornithine decarboxylation. Cloudy medium: Positive for motility. Red color after Kovac’s reagent: Positive for indole. Nitrate and Nitrite Reduction Test The nitrate reduction test determines if bacteria can reduce nitrate (NO₃) to nitrite (NO₂) or further reduce nitrite to nitrogen gas (N₂) or other nitrogenous compounds. Procedure 1.Inoculate the organism into a broth containing potassium nitrate (0.1%). 2.Incubate for 24 hours. 3.After incubation, add N,N-dimethyl-α-naphthylamine and sulfanilic acid to detect nitrite. 4.Red color indicates the presence of nitrite, confirming nitrate reduction. Nitrate and Nitrite Reduction Test If no color develops Add zinc dust to the medium. Zinc reduces nitrate to nitrite. A red color after zinc indicates a true-negative result (nitrate was not reduced by the bacteria). If no red color forms after zinc, this indicates that nitrate was reduced beyond nitrite, possibly to N₂, NO, or N₂O. Results Red after first reagents: Positive for nitrate reduction to nitrite. Red after zinc: Negative for nitrate reduction. No color change after zinc: Positive for nitrate reduction beyond nitrite to nitrogen gas (N₂). Oxidase Test The oxidase test is used to detect the presence of the cytochrome oxidase enzyme, which participates in the electron transport chain by oxidizing reduced cytochrome with oxygen. A drop of Kovac’s oxidase reagent (0.5% or 1% aqueous solution of tetramethyl-p-phenylenediamine dihydrochloride) is added to a piece of filter paper. Procedure A colony is rubbed onto the moistened filter paper using a wooden applicator stick. The development of a lavender color within 10 to 15 seconds indicates a positive reaction. Alternate reagents: p-Aminodimethylaniline oxalate is a less sensitive but more stable and cheaper alternative reagent. Important Note: Oxidase testing should not be done on bacteria grown on media containing dyes like eosin- methylene blue (EMB), as these dyes can cause false- positive results. Sulfide-Indole-Motility (SIM) Agar The SIM test is a semi-solid agar used to differentiate gram-negative bacteria, especially Enterobacterales. It evaluates: 1.Motility 2.H₂S production 3.Indole production Procedure A straight stab is made down the center of the medium using an inoculating needle. Motility: Cloudiness or spreading away from the stab line indicates a positive result. H₂S production: A black precipitate indicates the production of hydrogen sulfide. Indole production: After incubation, Kovac’s reagent is added, and a pink to red color indicates a positive indole result. Urease Test The urease test detects the ability of a microorganism to hydrolyze urea into ammonia, water, and carbon dioxide using the enzyme urease. The ammonia raises the pH of the medium, causing a color change. Procedure Christensen’s urea agar is commonly used, containing urea and phenol red as a pH indicator. The surface of the agar slant is inoculated but not stabbed. If the organism hydrolyzes urea, ammonia is released, raising the pH and turning the medium a bright pink. Results Bright pink: Positive for urease activity (alkaline pH). No color change: Negative for urease activity. Manual Multitest Systems for Microbial Identification Microbial identification can be achieved using various methods that generally fall into five categories: 1.pH-based reactions: Changes in pH indicate metabolic processes. 2.Enzyme-based reactions: Detection of specific enzyme activities. 3.Utilization of carbon sources: Ability to metabolize different carbon sources. 4.Visual detection of bacterial growth: Observing growth characteristics. 5.Molecular assays: Techniques based on the characterization of molecules such as fatty acids, nucleic acids, and proteins. Immunoassays detect microbial antigens using antibodies, while molecular assays can identify organisms without the need for microbial growth. Manual Multitest Systems for Microbial Identification Automated or packaged kit systems often facilitate identification through computer-assisted databases or numeric codes derived from metabolic profiles. Each metabolic reaction is recorded as a positive (+) or negative (–), which are then converted into binary codes. These codes are matched with a database to assign a probability of correct identification. Analytical Profile Index (API) The Analytical Profile Index (API), introduced by bioMérieux in 1970, is widely used for identifying gram-negative fermentative bacteria (API 20E). The system includes: 20 cupules attached to a plastic strip, each containing lyophilized substrates. Bacterial suspension is prepared in saline to rehydrate the substrates. The strip is incubated at 35°C for 18 to 24 hours, with certain cupules requiring mineral oil overlay for anaerobic conditions. Analytical Profile Index (API) The oxidase test is performed separately to finalize the profile number, which is a 7-digit code. This number is cross-referenced with a manufacturer-provided database for identification. Supplemental tests, such as nitrate reduction and motility, can refine the profile number if necessary. Accuracy: The API system shows high accuracy rates — approximately 87.7% for common Enterobacterales (e.g., Escherichia coli, Klebsiella pneumoniae) and 78.7% for less common isolates within 24 hours. Other Multitest Systems Several alternative multitest systems exist, including: Crystal E/NF (BD Diagnostic Systems) RapID NF Plus (Thermo Fisher Scientific) Microbact Biochemical Identification (Thermo Fisher Scientific) Enterotube II (BD Diagnostic Systems) Micro-ID (Thermo Fisher Scientific) Gen III MicroLog M (Biolog) Other Multitest Systems Rapid and Automated Systems Historically, microbiologists relied on traditional culture methods to identify bacteria, which could take 24 to 48 hours for isolation and additional time for biochemical identification. The need for rapid diagnosis has become increasingly important in clinical microbiology, especially with the rise of new pathogens, healthcare-associated infections, and multidrug-resistant organisms. Rapid and accurate identification of infectious agents can significantly improve patient outcomes by facilitating timely antimicrobial therapy. Rapid Identification Methods Direct Microscopy: Provides quick results (15 to 30 minutes) from body fluids. Useful for initial assessments, such as Gram stains from cerebrospinal fluid in cases of suspected meningitis. However, sensitivity is generally low, which limits its standalone use. Miniaturization of Biochemical Tests: In the 1950s and 1960s, traditional biochemical tests were miniaturized into smaller tubes and molded plastic vessels. Although this made testing more convenient, it did not significantly reduce turnaround times. Rapid Identification Methods Computerized Databases: Introduced in the 1970s to analyze multiple test results simultaneously, improving reliability but not speed. Semiautomated Instruments: Emerged in the mid- to late 1970s, these instruments reduced turnaround times and improved accuracy in identification and susceptibility testing. Rapid Methods: Encompass a range of procedures yielding faster results than conventional methods. Examples include enzyme immunoassays (e.g., detecting Clostridioides difficile toxins) and fluorescence quenching methods for detecting Mycobacterium tuberculosis. Microscopic procedures using stains and fluorescent antibodies also fall under this category. Rapid Identification Methods Commercial Identification Kits and Automated Systems Packaged Identification Kits: Often use chromogenic or fluorogenic substrates. Chromogenic substrates produce color upon enzyme cleavage, while fluorogenic substrates emit fluorescence only after activation. Reaction endpoints typically range from 2 to 6 hours, with some requiring overnight incubation. Automated Instruments: Some systems incubate, read, and interpret results automatically, reducing the need for manual input. They may utilize spectrophotometry, numeric coding, and computerized databases for analysis. Rapid Biochemical Test Perform on Isolated Colonies Rapid biochemical tests are crucial for quick presumptive differentiation among groups of microorganisms or for the identification of specific bacterial species. While one test may provide initial insights, multiple tests are often necessary for a definitive identification. Here's an overview of key principles and methods: Principles of Rapid Biochemical Tests Modes of Action: These tests detect end products from carbohydrate metabolism or enzymatic activity, typically resulting in color changes or other measurable reactions. Test Formats: Common formats include plastic cupules, reaction chambers, or filter paper strips containing dehydrated reagents. Carbohydrate Utilization and Chromogenic Substrates Testing Methodology: A bacterial suspension or colony is added to the reaction system. A positive reaction is indicated by a measurable enzymatic activity or color change. Multitest Kits: These allow for one inoculum to be tested across multiple reaction sites, yielding various results simultaneously. Modifications for Speed: Conventional methods have been adapted to reduce medium volumes and increase bacterial concentrations, allowing for faster results. Carbohydrate Utilization and Chromogenic Substrates Advantages of Enzymatic Methods Growth Independence: Since these tests rely on preformed enzymes, they do not require the organism to grow, allowing results to be obtained in minutes to hours. Sensitivity: Enzymatic tests are sensitive to the presence of the enzyme, although they may lack specificity for genus and species identification. Factors Affecting Sensitivity: The concentration and stability of the substrate, enzyme, and age of the inoculum can all influence sensitivity. Automated Identification Systems Automated identification systems have revolutionized microbiology laboratories by streamlining the identification process and improving turnaround times. These systems primarily utilize turbidity, colorimetry, or fluorescence technology to identify microorganisms efficiently. Advantages of Automated Systems Efficiency Data Integration Predictive Accuracy Rapid Reporting MicroScan Systems Developed by Beckman Coulter, the MicroScan system features 96-well microtiter trays with 32 reagent substrates for bacterial and yeast identification. Components autoSCAN and WalkAway: The autoSCAN reads standard panels, while WalkAway fully automates incubation, reagent addition, reading, and reporting. Combo Trays: These include antimicrobial susceptibility tests along with biochemical tests. Rapid Panels: Provide preliminary results in as little as 2 hours and final results in about 18 hours. Performance: Studies show high accuracy in identifying bacteria from positive blood cultures, with 96.5% correct identifications. TREK Diagnostic System Systems: Includes the Sensititre Autoreader and the fully automated Sensititre ARIS2X system. Technology: Uses fluorescence to detect bacterial growth and enzyme activity, with biochemical reactions formatted for fluorescent signal detection. Results: Available within 5 hours, with capabilities for overnight incubation. Vitek 2 System History: Introduced in the 1980s by bioMérieux Inc. Functionality: A bacterial suspension is prepared, loaded into a card, and placed in a reader-incubator for optical scanning. Performance: Identifies various organisms including gram- positive cocci and yeasts. Studies have shown high accuracy in identification when compared to 16S rRNA sequencing and MALDI-TOF mass spectrometry, with excellent agreement rates (e.g., 92.59% match with 1020 isolates). BD Phoenix Automated System Introduction: Launched in 2004, the BD Phoenix system is an automated microbiology platform that enhances laboratory efficiency by minimizing hands-on time. Panel Capacity: Holds up to 100 panels, including 99 samples and 1 control. Reading Frequency: Panels are read every 20 minutes for up to 16 hours. Result Availability: Typically provides results within 2 to 12 hours for bacteria and 4 to 15 hours for yeasts. Detection Methods: Utilizes colorimetric and fluorometric indicators for identification, with a redox indicator for antimicrobial susceptibility testing. Performance: A study showed a concordance rate of 95.9% for genus-level identification and 81.6% for species- level identification of nonfermentative gram-negative bacilli when compared to MALDI-TOF mass spectrometry. For gram-positive bacteria, the concordance was 100% at the genus level and 86.6% at the species level. Biolog OmniLog ID System Overview: The fully automated Biolog OmniLog ID System identifies organisms based on their ability to utilize 71 carbon sources and their sensitivity to 27 chemical substances. Panel Design: Uses a single 96-well plate per organism, where the reduction of tetrazolium violet serves as an indicator. Database: One of the largest in microbiology, containing over 2900 species or taxa. Applications: Panels are available for both aerobic and anaerobic gram-positive and gram-negative bacteria, as well as yeasts. Semiautomated Version: The Gen III OmniLog ID System offers semiautomated capabilities but is not approved for human in vitro testing; it is primarily utilized in agricultural and veterinary microbiology laboratories. Evaluation of Identification Systems Speed and Precision Rapid and automated systems are designed to deliver results faster and with greater precision than traditional methods, often within 2 to 4 hours, particularly for Enterobacterales. Performance varies; lower accuracy is noted with: Coagulase-negative staphylococci Non-fermentative gram-negative bacilli Slow-growing or fastidious bacteria, such as anaerobes. Evaluation of Identification Systems Laboratory Context The effectiveness of multitest and automated systems depends on the specific laboratory's needs and the institutions they support. Prospective, side-by-side evaluations comparing current methods with new systems are recommended to assess: Accuracy Cost-effectiveness Impact on workflow. Evaluation of Identification Systems Reference Method 16S rRNA sequencing is considered the reference method in comparative studies for identifying bacterial species. Sensitivity and Specificity Automated systems may show decreased sensitivity and specificity for biochemically inert and fastidious organisms. Supplemental and differential media, along with conventional biochemical tests, should remain available to support accurate identification. Evaluation of Identification Systems Database Management Regular updates to identification databases are crucial for maintaining accuracy and ensuring correct naming of microorganisms. Biochemical Identification -recap Phenotyping, serotyping, and genotyping all have important uses in the identification of bacteria. Molecular biology assays, such as nucleic acid amplification tests and MALDI-TOF mass spectrometry, provide accurate identification more rapidly than conventional biochemical testing. Bacteria can utilize carbohydrates oxidatively, fermenta- tively, or not at all. TSI agar and KIA are useful in determining the ability of bacteria to utilize certain carbohydrates and to produce H2S. Biochemical Identification -recap The MR and VP tests are used to determine the end products of glucose fermentation. Decarboxylase, dihydrolases, and deaminases are enzymes used by bacteria to metabolize amino acids. Numerous tests, such as citrate, DNase, indole, nitrate reduc- tion, oxidase, and urease, are important in the identification of gram- negative bacteria. Manual multitest systems have improved the identification of bacteria by simplifying inoculation of many different biochemical tests and producing numeric codes that can be compared with numbers in a database. Biochemical Identification -recap Rapid tests often use chromogenic or fluorogenic substrates to assay for preformed bacterial enzymes. Automated microbial identification systems offer accurate, rapid identifications with less hands-on time by laboratory scientists.