FSANZ TiO2 Assessment Report 2022 PDF
Document Details
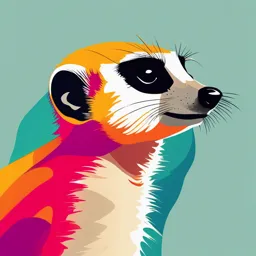
Uploaded by ImmenseGeometry
Swinburne University of Technology
2022
Tags
Summary
This FSANZ report reviews the safety of titanium dioxide (TiO2) as a food additive, in light of recent EFSA assessments. The report concludes that there is currently no evidence to suggest dietary exposures to food-grade TiO2 are a concern for human health. This document analyses published studies and considers the use of TiO2 in various food products.
Full Transcript
Titanium Dioxide as a Food Additive Food Standards Australia New Zealand 2022 Titanium dioxide as a food additive Executive summary Titanium dioxide (TiO21) is used as a white pigment for food colouring and is permitted in Australia and New Zealand for use in a wide range of foods at levels consis...
Titanium Dioxide as a Food Additive Food Standards Australia New Zealand 2022 Titanium dioxide as a food additive Executive summary Titanium dioxide (TiO21) is used as a white pigment for food colouring and is permitted in Australia and New Zealand for use in a wide range of foods at levels consistent with Good Manufacturing Practice. Its long use as a food additive has not given rise to reports of adverse effects. In this report, FSANZ has reviewed key evidence relating to the safety of TiO2 when used as a food additive. The review was initiated following the release in 2021 of an updated safety assessment of TiO2 by the European Food Safety Authority (EFSA) which concluded TiO2 could no longer be considered safe when used as a food additive. This conclusion is in contrast to previous favourable assessments by EFSA and others. There has been renewed interest in the safety of TiO2 following reports it may contain a proportion of particles in the nanoscale. FSANZ commissioned a review a number of years ago to consider the potential health risks associated with TiO2 and other food additives containing particles in the nanoscale. The review, published in 2016, concluded there was insufficient evidence at that time to support a risk assessment. The updated safety assessment published by EFSA in 2021 included consideration of studies performed with TiO2 nanoparticles. They concluded that although the evidence for general toxic effects was not conclusive, they could not rule out a concern for genotoxicity and consequently could not establish a safe level for daily intake of the additive. Other uncertainties included potential immunotoxicity, inflammation and neurotoxicity; potential induction of aberrant crypt foci in the colon; and lack of adequate carcinogenicity studies of TiO2 nanoparticles. Recent concerns about the safety of TiO2 as a food additive are primarily based on studies with TiO2 test items in the nanoscale range, or studies in which food-grade TiO2 has been sonicated in solution and administered by oral gavage or via drinking water. The relevance of such studies for assessing the human health risk from dietary exposure to food-grade TiO2 is limited because TiO2 in food is not sonicated, there is no evidence that TiO2 in foodstuffs de- agglomerates in the gastrointestinal tract and exposure to TiO2 via gavage or drinking water does not take into consideration food matrix effects. In vivo genotoxicity studies using dietary administration of food-grade TiO2 are currently lacking. However, there is no evidence that food-grade TiO2 administered by other modes (oral gavage, intraperitoneal injection) is genotoxic in vivo. In addition, no evidence of genotoxicity was found in in vitro studies with food-grade TiO2. Additional GLP- and test guideline-compliant in vivo genotoxicity (e.g. mutagenicity and micronucleus) studies with food-grade TiO2 would be valuable to confirm this conclusion. While aberrant crypt foci (considered potential pre-neoplastic lesions) were observed in the colon in a drinking water study with sonicated food-grade TiO2 at 10 mg/kg bw/day, these findings were not replicated in two studies using food-grade TiO2 administered via the diet at much higher doses (up to 267 or 1000 mg/kg bw/day). Observations of pre-neoplastic lesions are also inconsistent with the findings of a 2-year bioassay of TiO2 in rats and mice conducted by the United States National Cancer Institute. No evidence of toxicity or carcinogenicity was observed at dietary concentrations up to 1 INS 171; referred to as E171 in Europe. 1 50,000 ppm in this study. While questions have been raised about whether the test item used in this study was sufficiently representative of TiO2 preparations used as a food additive, recent unpublished information submitted to FSANZ confirms its suitability. A recent extended one-generation reproductive toxicity study in rats with food-grade TiO2 administered via the diet at doses up to 1000 mg/kg bw/day found no evidence of systemic toxicity, developmental or reproductive toxicity, developmental neurotoxicity or developmental immunotoxicity. Based on the data currently available, FSANZ concludes there is no evidence to suggest that dietary exposures to food-grade TiO2 are of concern for human health. 2 Contents Executive summary........................................................................................................... 1 1. Background.................................................................................................................... 4 2. Assessment approach and methodology........................................................................ 5 2.1 Scope...................................................................................................................... 5 2.2 Call for data and literature search............................................................................ 5 2.3 TiO2 test items......................................................................................................... 5 3. Hazard assessment........................................................................................................ 7 3.1 Toxicokinetics.......................................................................................................... 7 3.2 Toxicity data...........................................................................................................10 3.2.1 Carcinogenicity................................................................................................10 3.2.2 Genotoxicity....................................................................................................13 3.2.3 Developmental and reproductive toxicity.........................................................17 3.2.4 Immunotoxicity................................................................................................18 3.2.5 Neurotoxicity...................................................................................................19 4. Discussion and conclusions..............................................................................................19 5. References....................................................................................................................21 Appendix 1: Independent literature search........................................................................26 3 1. Background Titanium dioxide (TiO2; E171) has been used as a white pigment for food colouring for decades. It is listed in Schedule 16—3 of the Australia New Zealand Food Standards Code (the Code), ‘Colourings permitted at Good Manufacturing Practice (GMP)’. TiO2 is permitted for use in a wide range of foods in Australia and New Zealand including dairy products, confectionary, bakery products and beverages. The Joint FAO/WHO Expert Committee on Food Additives (JECFA) evaluated TiO2 in 1969. JECFA concluded that studies in several species, including humans, do not show significant absorption or tissue storage and that no toxicity was observed following absorption. An acceptable daily intake (ADI) ‘not limited’ was assigned, a term previously used by the Committee to refer to food additives that do not represent a hazard to health (WHO 1969). In 2012 it was reported that food-grade TiO2 may contain up to approximately 36% of particles in the nanoscale, raising new interest in its safety (Weir et al. 2012). In light of this observation, FSANZ commissioned a review of the scientific literature on whether there is evidence of health risks associated with oral ingestion of TiO2 and other food additives which may contain a proportion of material in the nanoscale range. This review noted that TiO2 has been used safely in food for decades, and concluded the weight of evidence does not support claims of significant health risks for food-grade TiO2 (ToxConsult 2016). The European Food Safety Authority (EFSA) re-evaluated TiO2 in 2016, and concluded based on the available data that TiO2 used as a food additive did not raise concern with respect to genotoxicity, and that it was not carcinogenic after oral administration (EFSA 2016). A number of uncertainties were highlighted, however, and it was recommended that an extended one-generation reproductive toxicity (EOGRT) study be performed. Another uncertainty identified related to characterisation of the material used as a food additive, including particle size. Following an evaluation of data submitted by interested business operators, EFSA recommended the EU specifications for TiO2 be amended to include additional parameters related to its particle size distribution. That is, > 100 nm for median minimum external dimension, equivalent to less than 50% of the number of constituent particles being below 100 nm. Because the data demonstrated the presence of a fraction of nanoparticles in TiO2, it was recommended the toxicological database be reassessed in line with the data requirements set out in EFSA’s 2018 guidance on nanotechnology (EFSA 2019). In May 2021, EFSA published an updated safety assessment of TiO2 as a food additive, which included consideration of studies performed with TiO2 nanoparticles. EFSA concluded that although the evidence for general toxic effects was not conclusive, they could not rule out a concern for genotoxicity and consequently could not establish a safe level for daily intake of the additive. Other uncertainties included potential immunotoxicity, inflammation and neurotoxicity; potential induction of aberrant crypt foci in the colon; and lack of adequate carcinogenicity studies of TiO2 nanoparticles. Following publication of the EFSA opinion on TiO2, the Food Standards Agency (FSA) initiated a review of the report, and sought independent expert advice from the UK Committee on Toxicity of Chemicals in Food, Consumer Products and the Environment (COT) and the Committee on Mutagenicity of Chemicals in Food, Consumer Products and the Environment (COM). The COT issued an interim position paper on TiO2 in January 2022, which concluded that on balance, the weight of evidence did not support the conclusions drawn by EFSA. The COT 4 also agreed with comments of the COM with regards to risk communication that “As it stands the conclusion is highly risk adverse based on the weak evidence available, and it might create unnecessary concern to the public.” They considered that care should be taken when expressing the conclusions as they might cause unnecessary concern (COT 2022). The FSA is now conducting its own review of the safety of TiO2 as a food additive, with support from the COT and COM. In June 2022, Health Canada published a State of the Science report on TiO2 as a Food Additive. This review noted the adverse effects associated with oral exposure to TiO2 are largely derived from non-guideline studies that administered stable, homogenized suspensions of ultrasonically dispersed particles by gavage or in drinking water, which do not fully represent exposure to TiO2 as a constituent of food. Health Canada concluded there is no conclusive scientific evidence that the food additive TiO2 is a concern for human health (Health Canada 2022). TiO2 has also been added to the Priority List of Food Additives Proposed for Evaluation by JECFA. In light of the concerns raised by EFSA FSANZ has reviewed key evidence relating to the safety of TiO2 when used as a food additive. 2. Assessment approach and methodology 2.1 Scope The purpose of FSANZ’s assessment is not to comprehensively review the toxicity of TiO2, but to focus on the key areas highlighted as being of concern by EFSA. A full review of TiO2 by FSANZ was considered unnecessary at this stage given TiO2 is scheduled for re- evaluation by JECFA. 2.2 Call for data and literature search A call for data was issued by FSANZ on 8 July 2021, requesting information on the physico- chemical properties of TiO2 as currently used as a food additive, genotoxicity studies and any other studies relevant to the safety of TiO2 as a food additive. The call for data closed on 17 September 2021. FSANZ also conducted a literature search for relevant toxicokinetic and toxicological studies in animals and humans using PubMed and Web of Science. The search terms used are listed in Appendix 1. In addition, the recent EFSA assessments and data submitted to FSANZ were reviewed to ensure key studies had been captured. The titles and abstracts of all studies identified in the literature search were screened for their relevance using inclusion and exclusion criteria (see Appendix 1). Studies that remained following this initial screening underwent a full text review, which included further consideration of their relevance and reliability. 2.3 TiO2 test items A large number of toxicokinetic and toxicity studies of TiO2 have used sonication to generate stable dispersions of TiO2 particles in simple liquid solutions. In addition to studies with food- grade TiO2, other studies have used TiO2 nanoparticles < 100 nm or other non-food grade forms of TiO2 such as pigment grade or cosmetic grade TiO2. 5 This review has focussed on in vivo studies in which food-grade TiO2 was administered via the diet without prior sonication. Where relevant, studies involving other modes of administration or grades of TiO2 were taken into account as additional supporting evidence. The reason for this approach is as follows: For TiO2 to achieve the desired whitening effect in food, optimal light scattering is required. The ideal primary particle size for food-grade TiO2 is therefore in the range of 200 – 350 nm, which is approximately half the wavelength of visible light (400 – 700 nm). In contrast, ultrafine particles below 100 nm are transparent and not suitable for whitening purposes in food (Winkler et al. 2018). Information submitted to FSANZ by food industry groups indicates that, when used as a food additive, TiO2 is incorporated in foods without sonication. It is noted that the ability of TiO2 to function as a whitener/opacifier would be reduced or eliminated by sonication as it would break up the agglomerates that cause light scattering. Studies in which TiO2 is administered as a dispersion in a liquid also do not account for interactions of TiO2 with the food matrix as well as the contents of the gastrointestinal tract. Interactions with the food matrix may result in agglomeration of TiO2 particles and affect its bioavailability, while TiO2 particles incubated in a simulated intestinal digestion juice form a corona of bile acids and proteins, and the extent of macromolecular interactions and composition of the resulting corona depends on both the surface chemistry of the particles and their diameter (Winkler et al 2018). The available evidence does not suggest that TiO2 in foods de-agglomerates in the gastrointestinal tract. A recent study of food-grade TiO2 in simulated oral, gastric and intestinal phases reported formation of large agglomerates up to 500 µm mediated by interactions with enzymes from the digestive fluid (Dudefoi et al 2021). Sonication of water results in formation of hydrogen and hydroxyl radicals that subsequently recombine to form hydrogen peroxide. TiO2 nanoparticles are themselves sonocatalysts, also contributing to the formation of highly reactive hydroxyl radicals. These reactive species may interact with test materials, resulting in oxidative and other chemical transformations that may influence the results of toxicity studies (Taurozzi et al 2011). Additional changes may occur if the dispersion medium contains other chemical species (Taurozzi et al 2011). A review of in vitro genotoxicity studies of TiO2 nanoparticles noted that modifications potentially induced by sonication may lead to false positives (Charles et al 2018). Studies using test items comprised entirely of TiO2 nanoparticles are of limited relevance for assessing the safety from dietary exposure to TiO2 when used as a food additive. A recent comparison of food-grade TiO2 and the nanomaterial P25, which is used for photocatalytic applications and the subject of many toxicity studies, found a number of differences in physicochemical properties, including differences in primary size distribution, specific surface area, crystalline form and surface composition (Dudefoi et al 2017). As food-grade TiO2 contains a substantial number of particles in the nanoscale range, studies using food-grade TiO2 are also relevant for evaluating the potential toxicity of any nanoparticles that may be present. Several unpublished toxicity studies with food-grade TiO2 were submitted in response to FSANZ’s call for data. The test item in these studies corresponds to a commercial brand of E171, referred to as E171-E, which is one of a cross-section of E171 grades on the market 6 for which data on particle size distribution were submitted to EFSA (EFSA 2019). As E171-E had the highest nanoparticle content this grade material was selected for use in subsequent toxicity testing. The median particle size of E171-E was reported as 104 nm (average 110 – 115 nm) with approximately 36 – 45% of particles by number < 100 nm (EFSA 2019; Blevins et al 2019). 3. Hazard assessment 3.1 Toxicokinetics Studies in laboratory animals An unpublished study evaluating the relative oral bioavailability in male and female CD rats of five different TiO2 grades, including the food additive E171-E, was provided to FSANZ (Provivo Biosciences 2022). The study was compliant with Good Laboratory Practice (GLP) and performed in accordance with OECD Test Guideline (TG) 417. The bioavailability of the five different test items was compared to a soluble titanium reference substance (titanium(IV)bis(ammonium lactate)dihyroxide solution, 50% in water). The TiO2 test items were administered by single gavage at 1000 mg/kg bw and the reference substance administered orally at 100 mg/kg bw and intravenously at 10 mg/kg bw. Blood total titanium concentrations were monitored for 96 hours post dosing. A comparison of the blood titanium concentrations in rats administered the reference substance intravenously with that of rats given E171-E orally found that the maximum relative oral bioavailability of E171-E was 0.0013%. Blood titanium levels after administration of the other TiO2 test items were below the limit of detection after correction for background concentrations. In a non-guideline study, food-grade anatase TiO2 (particle diameter range 50 – 350 nm, maximum frequency 100 nm; source Sensient Colors) was administered via the diet to C57CL/6 mice (6/sex/group) for 18 weeks (Riedle et al 2020). Concentrations in the diet were 6.25, 62.5 and 652 mg/kg diet, resulting in calculated doses of 0.68, 6.9 or 63 mg/kg bw/day and 0.76, 7.9 or 72 mg/kg bw/day in males and females, respectively. At the end of the treatment period mice were killed and the gastrointestinal tracts were harvested. Peyer’s patches were excised and examined by confocal microscopy and scanning electron microscopy (SEM) with energy dispersive X-ray spectroscopy (EDX) analysis. TiO2 particles were detected at the base of the Peyer’s patches in all three treatment groups, with a dose- dependent increase. Systemic bioavailability was not assessed in this study, however. In a GLP-compliant study conducted in line with OECD TG 417, the absorption, distribution and excretion of food-grade TiO2 was assessed following dietary administration in rats (Farrell and Magnuson 2017). Anatase TiO22 was administered via the diet at a concentration of 200 ppm to Sprague-Dawley rats (age 6 – 10 weeks; 10/sex/group) for 7 days. Doses were calculated as 30 mg/kg bw/day in males and 24 mg/kg bw/day in females. Control animals were fed diets without added TiO2 (background concentration 7 – 9 ppm wet weight). At the end of the exposure period animals were fed control diet and housed individually in metabolism cages, with urine, faeces and cagewash collected. At 1 hour, 24 h and 72 h after treatment was withdrawn, 3 rats/sex/group were killed and blood, liver, kidney and muscle samples collected. The titanium concentration in all samples was determined by inductively coupled plasma – atomic emission spectrometry (ICP-AES). Titanium concentrations in tissues were below the limit of detection (LOD; 0.1 – 0.2 mg/kg wet weight) in the majority of samples (65%). Sporadic observations of titanium concentrations above the LOD were reported in all tissues, but this occurred at a similar 2 E171 from Brenntag, USA; 36% discrete but aggregated particles < 100 nm 7 frequency and concentration range in controls and treated rats. Whole blood concentrations of titanium were below the limit of quantification (LOQ; < 0.04 mg/L equivalent to < 2% daily dose) for all groups. Titanium concentrations in urine were also below the LOQ. Titanium was excreted in the faeces of rats given anatase TiO2, with 42 – 51% of the mean daily dose recovered in males and females, respectively. Faecal excretion was only assessed over the 72 h following withdrawal of treatment, so the total amount excreted in the faeces could not be determined. The study authors concluded that food-grade TiO2 administered in the diet is not appreciably absorbed and distributed in mammalian tissues, with no evidence of tissue accumulation after repeated oral exposure. In EFSA’s recent review, concerns were raised that toxicokinetic studies involving intravenous (i.v.) administration of TiO2 nanoparticles to rats suggested long half-lives (Geraets et al 2014; Disdier et al 2015; Kreyling et al 2017a) and therefore there may be a potential for accumulation of TiO2 nanoparticles in the body, even if systemic exposure from oral administration is low. However, a companion study to the i.v. study by Kreyling et al involving oral gavage administration of 48V-radiolabelled TiO2 nanoparticles to rats found that 77.4 ± 3.1% and 99.7 ± 0.01% of the administered dose was excreted in the faeces by 24 hours and 7 days after exposure, respectively (Kreyling et al 2017b). Approximately 0.6% of the administered dose was absorbed in the first hour post-gavage based on analysis of 48V-activity, with the fraction retained in the body declining to ~ 0.2% after 4 hours and ~ 0.05% after 7 days. While the vast majority of TiO2 nanoparticles was distributed to the liver following i.v. administration, only a small fraction was observed in the liver and other organs following oral administration with the greatest proportion of the absorbed dose found in the carcass (skeleton and soft tissue minus major organs). The significant differences in pharmacokinetics between the two exposure routes led the study authors to conclude that i.v. injection is not an adequate surrogate for assessing biokinetics following oral exposure to TiO2 nanoparticles (Kreyling et al 2017b). Studies in humans In an unpublished human volunteer study reviewed by JECFA (West and Wyzan 1963), five males consumed 5 g of food-grade TiO2 suspended in milk for three consecutive days, with urine samples being collected for five days from the start of the intervention. No change in urinary titanium concentrations was detected, suggesting a lack of significant absorption (WHO 1969). Limited details of another human volunteer study are available in the English language abstract of a paper published in German (Böckmann et al 2000), with some additional details discussed in more recent papers (Jones et al 2015; Naegli and Gsell 2022). In this study, blood titanium concentrations in male volunteers were measured by ICP-AES before and after oral administration of 23 mg and 46 mg of two different grades of anatase TiO23. Mean pre-dose blood titanium concentrations were reported to be 11.2 ng/mL (range 5.9 – 18.1 ng/mL). After TiO2 ingestion, increased titanium blood concentrations were observed, with a high degree of individual variation (Jones et al reported the time of peak blood levels ranged between 30 minutes and 12 h). Doubling the administered dose did not result in a doubling of blood levels. Absorption of the larger particles, administered as a powder, was lower than that of the smaller particles which were administered as gelatine capsules. It was suggested that the lower absorption was most likely due to agglomeration in the stomach acid, which gelatine capsules may have protected against. 3 mean particle size 160 or 380 nm; administered as gelatine capsules or powder, respectively 8 Seven human volunteers with normal intestinal permeability were asked to consume gelatine capsules containing 100 mg pharmaceutical/food grade anatase TiO24 (Pele et al 2015). Blood samples were collected at baseline and at 0.5, 1, 1.5, 2, 3, 6, 8 and 10 hours following TiO2 ingestion. Blood was analysed for the presence of TiO2 particles by dark field microscopy, and for total titanium by ICP-mass spectrometry (ICP-MS). Occasional positive signals assumed to be TiO2 particles were detected in baseline blood samples, with a significant increase observed from 2 h, reaching a peak at 6 h and declining at 8 and 10 h. Blood titanium concentrations were approximately 2 ng/mL at baseline and up until 3 h, increasing to a peak of ~ 12 ng/mL at 6 h and declining to ~ 5 ng/mL 10 h post-ingestion. Oral absorption of three commercially available TiO2 materials was assessed in nine human volunteers (4 male/5 female) (Jones et al 2015). The three test items were 15 nm anatase (~ 100% < 50 nm by number, ~ 30% < 50 nm by weight); < 100 nm rutile (95% 48-154 nm by number); and < 5000 nm rutile (100% > 105 nm by number, ~ 100% > 700 nm by weight). Volunteers consumed a 5 mg/kg bw single oral dose of TiO2 dispersed in water, and serial urine (24 h before dosing until 72 hours post-dose) and blood (baseline and 2, 4, 24 and 48 h post-dosing) were collected for analysis of titanium concentration by ICP-MS. The three different materials were given at least 4 weeks apart. Statistical modelling found no significant differences between mean pre-dose and post-dose titanium levels in urine, whole blood, serum or erythrocytes. The study authors concluded that less than 0.1% of the administered dose was absorbed. Levels of total titanium and TiO2 particles were assessed in 15 post-mortem human livers and spleens from 6 men and 9 women who had died age 56 – 104 years (Heringa et al 2018). Titanium concentrations were below the limit of detection (LOD) of 0.01 mg/kg tissue in 8 liver samples and 1 spleen sample. Based on values above the LOD, total titanium content in the liver and spleen ranged from 0.02 – 0.09 mg/kg tissue (mean 0.04 ± 0.02 mg/kg) and 0.02 – 0.4 mg /kg tissue (mean 0.08 ± 0.1 mg/kg), respectively. Two of the study subjects carried a titanium implant (type not reported), but titanium concentrations in these individuals were similar to those observed in the other subjects. TiO2 particles were detected in 7/15 liver samples and 13/15 spleen samples. Number-based size distributions in liver and spleen were 85 – 550 nm and 85 – 720 nm, respectively, with 24% of particles < 100 nm, although this was likely to have been underestimated as 85 nm was the size-based LOD of the single-particle ICP-high resolution MS ((sp)ICP-HRMS) method. No correlation was observed between the liver and spleen TiO2 particle content within the same subjects. A follow-up to the above study examined the presence of total titanium and TiO2 particles in the liver, spleen and kidney from 15 deceased persons (7 men/8 women, aged 64 – 98 years) (Peters et al 2020). Jejunum and ileum samples were collected from 12 of the subjects. Total titanium concentrations were below the LOD (0.01 mg/kg tissue) in four liver samples, two spleen samples and one kidney and jejunum sample. Median titanium concentrations were 0.02 (liver), 0.04 (spleen), 0.05 (kidney), 0.13 (jejunum) and 0.26 (ileum) mg/kg. Particulate TiO2 explained about 80% of the total titanium concentration, comprising primary particles, aggregates or agglomerates in the range of 50 – 500 nm diameter, with a mode of 100 – 160 nm. About 17% of the detected TiO2 particles had a size < 100 nm. Summary Taken together, the evidence from the animal and human studies above suggests that absorption of TiO2 following oral exposure is very limited. Newly available studies with food- grade TiO2 submitted to FSANZ indicates that the relative oral bioavailability in rats is ≤ 0.0013%. The majority of absorbed material is retained in the Peyer’s patches in the intestine, with some distribution to the liver, spleen and kidneys. Given the age of the 4 Kronos® 1171; d50 260 nm; Fagron UK 9 subjects in the available studies with human cadavers, TiO2 levels in the tissue can be expected to have achieved steady state, and these studies indicate relatively low bioavailability. FSANZ’s previous review of TiO2 noted that in humans, the presence of inorganic micro- particles containing TiO2 and other sub-micron particles in basal ‘pigmented cells’ of Peyer’s patches is a normal occurrence throughout Western populations. These particle-containing pigmented cells are mainly mature macrophages that appear to be of low metabolic and immunological activity, with no evidence for differential phenotype or activation (ToxConsult 2016; Thoree et al 2008). 3.2 Toxicity data 3.2.1 Carcinogenicity No chronic toxicity/carcinogenicity studies involving oral administration of food-grade TiO2 E171 are available in the scientific literature. The US National Cancer Institute (NCI) conducted a chronic dietary toxicity/carcinogenicity bioassay using a TiO2 material identified as Unitane 0-220 (NCI 1979). This study has previously been considered suitable for assessing the potential carcinogenicity of food-grade TiO2 (EFSA 2016; ToxConsult 2016), although more recently questions have been raised about whether Unitane 0-220 is sufficiently representative of food-grade TiO2 (EFSA 2021). Unitane 0-220 was described by the NCI as 98% minimum TiO2 anatase, with a moisture content < 0.4%, obtained from American Cyanamid Company, Wayne, New Jersey. The particle size distribution was not reported in this study. NCI (1979) reported that the Unitane 0-220 lots tested in the bioassay contained low levels (0.1 – 1.0%) of niobium, chlorine, phosphorus, silicon and/or potassium. Unitane 0-220 is no longer in production and neither the manufacturing company or factory still exist. However, two samples of Unitane 0-200 were retained by member companies of the TiO2 Manufacturers Association (TDMA). An unpublished report submitted to FSANZ provides a comparison of analytical data for these samples, as well as historical records of previous sample analyses, with data on food-grade E171 currently in use and EFSA’s draft specification for E171 (TDMA 2022). These data indicate that Unitane 0-220 has a median particle diameter of 109 – 135 nm, with 20 – 44% of particles by number < 100 nm. This is consistent with anatase E171 samples analysed by the TDMA (d50 by number 104 – 166 nm; 11.4 – 45.6% < 100 nm by number). The data also demonstrate that Unitane 0-220 meets the draft EU specification for food-grade TiO2 in relation to particle size, absence of surface treatments or coatings and purity including elemental composition and levels of metal contaminants. This information confirms that the US NCI study using Unitane 0-200 is suitable for assessing the potential carcinogenicity of food-grade TiO2. In this study, groups of Fischer 344 (F344) rats and B6C3F1 mice (50/sex/group) were administered TiO2 in the diet at concentrations of 0, 25,000 or 50,000 ppm for 103 weeks and then observed for one additional week prior to study termination (NCI 1979). These concentrations were calculated by EFSA as equivalent to 0, 1125 and 2250 mg/kg bw/day and 0, 1450 and 2900 mg/kg bw/day in male and female rats, respectively (EFSA 2016). Doses in male and female mice were equivalent to 0, 3250 and 6500 mg/kg bw/day and 0, 4175 and 8350 mg/kg bw/day, respectively. These doses were selected based on 13-week dietary studies in rats and mice (10/sex/group) in which no adverse effects were found at concentrations up to 100,000 ppm, with the maximum concentration in the bioassay being 10 the maximum allowed for use in chronic studies in the Carcinogenesis Testing Program. Clinical signs, body weight and food consumption were monitored regularly. Animals that were moribund and those that survived to the end of the study were killed, and gross and microscopic examination of major tissues/organs and all gross lesions was performed. Body weight and body weight gain were not affected by administration of TiO2 in rats or mice. No treatment-related clinical signs were observed, with the exception of white faeces in both species. Survival of rats and male mice were unaffected by TiO2. Survival of female mice in the high-dose group was shorter than that of the low-dose and control groups: 45/50 (90%) of control mice were alive at week 104, compared with 39/50 (78%) and 33/50 (66%) mice in the low-dose and high-dose groups, respectively. Non-neoplastic lesions observed in treated animals were of the usual kind and number observed in aged F344 rats and B6C3F1 mice. In male rats, pheochromocytomas of the adrenal medulla and fibromas of the subcutaneous tissue were observed at a slightly greater frequency in treated groups compared with controls. In female rats, an increased incidence of endometrial stromal polyps was observed in treated groups than controls. These lesions were not considered to be treatment-related as the incidence was within the historical control range for rats of the same strain, sex and age. Keratoacanthomas of the skin were observed in 3/50 high-dose male rats compared with 0/49 control and 0/50 low-dose male rats, with a significant dose-response trend. However, as the incidence in the high-dose group was not significantly higher than controls, it was not considered to be treatment-related. A significant positive dose-response trend for the combined incidence of C-cell adenomas or carcinomas of the thyroid was observed in female rats, but the incidence of these lesions in the high-dose group was not significantly higher than controls and it was concluded that they were not treatment-related. No significant increases in the incidence of tumours were observed in treated mice compared with controls. A slightly increased number of hepatocellular carcinomas was observed in high-dose male mice compared with controls, but the incidence was not statistically significant and was within the range seen in historical control mice of the same age and strain. It was concluded that TiO2 was not toxic or carcinogenic to F344 rats or B6C3F1 mice under the conditions of this study. Aberrant crypt foci EFSA’s 2021 review of TiO2 expressed concerns about the potential for induction of aberrant crypt foci (ACF) in the colon, based on a study by Bettini et al (2017). ACF are considered potential precursors to colorectal cancer, although not all ACF progress to neoplasia and questions have been raised about their value as a biomarker of colorectal carcinogenesis (Quintanilla et al 2019; Clapper et al 2020). Adult male Wistar rats (n=11 – 12 per group) were treated with 1,2-dimethylhydrazine (DMH) i.p. to induce colon carcinogenesis and 7 days later were exposed to food-grade TiO2 at 0, 0.2 or 10 mg/kg bw/day via drinking water for 100 days (Bettini et al 2017). A second study administered 0 (n=12) or 10 (n=11) mg/kg bw/day TiO2 for 100 days without DMH pre- treatment. The test item was ultrasonicated in water prior to administration, following the NANOGENOTOX dispersion protocol5. In the first study, 10 mg/kg bw/day E171 significantly increased the total number of aberrant crypts per colon as well as the number of large ACF per colon (defined as > 3 aberrant crypts per ACF) relative to DMH-only controls, but not in the 0.2 mg/kg bw/day group. No significant differences in the mean number of ACF per colon 5The NANOGENOTOX dispersion protocol involves sonication and aims to ensure nanomaterials are highly dispersed for in vitro and in vivo toxicity testing. 11 were observed in rats treated with TiO2 compared with the DMH-only controls, however. In the second study conducted in the absence of a colon carcinogenesis initiator, no ACF were observed in the colons of the vehicle control rats, while 4/11 animals exposed to 10 mg/kg bw/day E171 group developed 1 – 3 ACF per colon. The ACFs contained 1 – 3 aberrant crypts in the second study, with the exception of one comprising 12 aberrant crypts. EFSA considered that E171 at a dose of 10 mg/kg bw per day may induce development of ACF in male rats based on this study. FSANZ previously assessed this study in 2017 and concluded its design has a number of limitations which restrict its relevance to humans. A particular uncertainty relates to the relevance of the sonication dispersion protocol and delivery via drinking water to food additive use of TiO2. In 2017, the French Agency for Food, Environmental and Occupational Health and Safety (ANSES) issued an opinion on this study which concluded the results could not be used for risk assessment without confirmation in a study using dietary administration (ANSES 2017). EFSA also published an opinion on the Bettini et al study, concluding that the findings were not sufficient to raise a concern on the potential initiation or promotion properties of TiO2 on colon carcinogenesis (EFSA 2018). The spontaneous development of ACF is not an uncommon finding in control rats (Singh et al 2022; Furukawa et al 2002; Raju et al 2011). In addition, the presence of ACF with 1 – 3 aberrant crypts per focus in rodents is not predictive of tumour formation (Kristiansen, 1996; Magnuson et al 1993; Pretlow et al 1992). Given this, the finding of ACFs containing 1 – 3 aberrant crypts is not considered conclusive evidence of a potential toxicological concern following exposure to sonicated TiO2 in drinking water. Two studies conducted subsequent to Bettini et al have examined the potential effects of dietary administration of food-grade TiO2 on ACF formation in the colon, using E171-E. In addition, a test guideline-compliant sub-chronic toxicity study evaluated the effects of gavage administration of sonicated food-grade TiO2 on the gastrointestinal tract, as well as other endpoints. Blevins et al (2019) administered E171-E to 6 week old male Wistar Han (Crl:WI (Han)) rats (16/group) via the diet at concentrations of 0, 40, 400 or 5000 ppm for 100 days, resulting in calculated doses of 1.3, 3.6, 22.4 or 267 mg/kg bw/day, respectively. Two groups were included at each dose level, one of which was pre-treated with a single i.p. injection with DMH dihydrochloride. Clinical signs, body weight and food consumption were monitored and at the end of the study animals were killed and colon tissue was collected for analysis of ACFs and goblet cell number and length. Blinded histopathological analyses were performed on the spleen, liver, lung, duodenum, jejunum, ileum, colon and testes. Due to a technical issue, much of the epithelial surface of the colon samples examined for ACF were obscured when observed by light microscopy. However, significant increases in the mean number of ACF/cm2 and number of aberrant crypts/cm2 were observed in the groups pre-treated with DMH produced compared with the groups that were not pre-treated, suggesting that the ability to detect such changes was not significantly impaired. Treatment with E171 with or without DMH pre-treatment did not result in significant increases in the number of ACF or aberrant crypts compared with the respective controls. No effect of E171 on the number of goblet cells per colonic gland or colonic gland length was observed. No treatment-related histopathological changes were observed in the small and large intestine, liver, spleen, lungs or testes of rats treated with E171. Rats pre-treated with DMH displayed several histopathological changes compared with animals that were not pre-treated, but with no evidence of an effect of E171. One rat in the DMH + control group developed two invasive adenocarcinomas, and single adenomas were detected in one rat in each of the DMH + 40 12 ppm E171 group and DMH + 400 ppm E171 groups. No adenomas were detected in the DMH + 4000 ppm E171 group, however. In an unpublished extended one-generation reproductive toxicity (EOGRT) study conducted in accordance with OECD TG 443 and under GLP conditions, E171-E was administered to rats via the diet resulting in doses of 0, 100, 300 or 1000 mg/kg bw/day (LPT 2020). The F0 generation was exposed from 10 weeks prior to mating until weaning of the F1 generation. An evaluation of ACF in the colon of a satellite group (10/sex/group) of this generation was undertaken, using a definition of ‘foci containing more than two aberrant crypts’. Treatment with a colorectal tumour initiator was not included in this study. No evidence of ACF formation was observed following treatment with E171. In a study conducted in compliance with OECD TG 408, food-grade TiO2 dispersed in water by ultrasonication was administered by oral gavage to 5-week-old Sprague Dawley rats (10/sex/group) at doses of 0, 10, 100 or 1000 mg/kg bw/day for 90 days (Han et al 2021). Clinical signs and food consumption were monitored throughout the study. Blood was collected at necropsy for haematology and clinical chemistry analysis, tissues and organs were weighed and subjected to macroscopic and microscopic evaluation. All animals survived to the end of the study and no treatment-related clinical signs were observed. There were no treatment-related adverse effects on haematology, clinical chemistry, organ weights and incidence of macroscopic and histopathological changes, including in the gastrointestinal tract. TiO2 was detected on the surface of the stomach and in the mucosa in high dose rats (sex not reported). The study authors described this finding as ‘accumulation in the stomach’, despite TiO2 only being found on the stomach surface and in the mucosa. The authors considered the NOAEL to be between 100 and 1000 mg/kg bw/day based on this observation, however as cellular uptake was not demonstrated FSANZ considers the NOAEL to be 1000 mg/kg bw/day. Summary While Bettini et al (2017) reported evidence suggesting an increased incidence of ACF in the colon of rats exposed to sonicated food-grade TiO2 (10 mg/kg bw/day) dispersed in drinking water, such changes were not observed in two studies in which food-grade TiO2 was administered via the diet at substantially higher doses (up to 267 or 1000 mg/kg bw/day). An OECD TG-compliant study also found no treatment-related histopathological changes in the gastrointestinal tract of rats administered sonicated food-grade TiO2 by gavage at doses up to 1000 mg/kg bw/day for 90 days. In addition, the findings of Bettini et al (2017) are inconsistent with the NCI 2-year bioassay, which found no evidence of carcinogenicity of a test item comparable to food-grade TiO2 in the colon or other tissues in rats and mice at dietary concentrations up to 50,000 ppm, equivalent to doses of 2250 – 2900 and 6500 – 8350 mg/kg bw/day, respectively. The different modes of exposure in these studies may explain the different results. As noted previously, studies involving dietary administration of food-grade TiO2 are considered most relevant to human exposures to TiO2 in liquid and solid foods, and have been given the highest weight in this review. The evidence from such studies indicates that dietary exposure to food-grade TiO2 is unlikely to induce pre-neoplastic or neoplastic lesions in the colon or other tissues. 3.2.2 Genotoxicity A limited number of in vivo genotoxicity studies with food-grade TiO2 are available. Two studies of orally administered food-grade TiO2 were identified, as well as two studies involving intraperitoneal (i.p.) administration of Unitane 0-220. Although i.p. administration is 13 not directly relevant to food use, it may be expected to result in higher systemic exposure compared with oral exposures given the limited oral absorption of TiO2, and is therefore useful for hazard identification. Bettini et al (2017) administered 10 mg/kg bw/day food-grade TiO26 to adult male Wistar rats (10/group) by oral gavage for 7 days. The test item was ultrasonicated in water prior to administration, following the NANOGENOTOX dispersion protocol. Water was used as the negative control, but no positive control was included. Peyer’s patch cells were collected7 and the alkaline comet assay was used to detect DNA strand breaks and alkali-labile sites. A parallel digestion with formamidopyrimidine DNA glycosylase (Fpg), allowing the detection of oxidative DNA damage, was also performed. No induction of DNA damage was observed following treatment with TiO2 in the presence or absence of Fpg. Jensen et al (2019) administered food-grade TiO28 comprising three different size groups (135 ± 6, 305 ± 61 and 900 ± 247 nm) to female lean Zucker rats (Crl:ZUZ-Leprfa; 10/group) by oral gavage. TiO2 was suspended in water containing 2% foetal bovine serum (FBS) and sonicated for 16 minutes prior to administration. TiO2 was administered in single weekly doses of 0, 50 or 500 mg/kg bw/week for 10 weeks. Animals were killed 24 hours after the last exposure and DNA damage in liver and lung tissue samples was determined by the alkaline comet assay. DNA damage was assessed as DNA strand breaks and as oxidatively damaged DNA using Fpg and human oxoguanine glycosylase 1 (hOGG1). THP-1 cells treated with KBrO4 (5 mM) were used as a positive control for detection of Fpg- and hOGG1- sensitive sites. No differences in the level of DNA strand breaks, Fpg- or hOGG1-sensitive sites were observed in liver and lung tissue from vehicle controls and rats treated with either dose of TiO2. The positive control increased the level of Fpg- and hOGG1-sensitive sites as expected. Limitations of this study include that systemic exposure to the test item was not confirmed, and given the time lag between the last exposure and tissue collection it is possible that DNA repair could have taken place. Two studies by the US National Toxicology Program (NTP) evaluated the genotoxicity of a range of substances previously tested for rodent carcinogenicity by the NCI/NTP, including TiO2 (Shelby et al 1993; Shelby and Witt 1995). The TiO2 used in these studies was not described in detail, but is likely to have been Unitane O-2209. In a micronucleus study, male B6C3F1 mice (5/group) received i.p. injections of 0, 250, 500 or 1000 mg/kg bw/day of TiO2 (Unitane O-220) for three days (Shelby et al 1993). The vehicle control was corn oil and the positive control was dimethylbenzanthracene (DMBA). Mice were killed 24 hours after the final dose and the frequency of micronucleated polychromatic erythrocytes (PCEs) among 2000 PCEs was assessed in bone marrow and peripheral blood. Cytotoxicity was assessed by the percentage of PCE among 200 erythrocytes. In a second study, mice were injected with 0, 500, 1000 or 1500 mg/kg bw/day for three days and the incidence of micronuclei was examined in bone marrow. In the first study, no increase in micronuclei was observed in peripheral blood erythrocytes. In bone marrow, a significant positive trend with a significant increase in micronucleated PCEs at the high dose was observed in in the first test, however no dose-response was observed: the incidence at the middle dose was lower than that at the low dose. In addition, all values were within the range of solvent control data for the same sex and strain at the test facility. In the 6 E171; primarily anatase; primary particle size 118 ± 53 nm; 44.7% < 100 nm; obtained from a French commercial food colouring supplier 7 timing of sampling not reported 8 E171; 99.8% anatase/0.2% rutile; from Bolsjehuset, a supplier of ingredients for candy production 9 The TiO was sourced from the NTP chemical repository and is stated to have previously been used in in vitro 2 genotoxicity studies by Tennant et al (1987). Tennant et al further indicated that the chemicals tested in their study were those tested for rodent carcinogenicity by NCI and NTP, and that where possible they tested the same chemical lot as was used in the rodent carcinogenicity studies. 14 second study, no significant trend was observed. A significant increase in micronucleated bone marrow erythrocytes was observed at 1000 mg/kg bw/day but not at 500 or 1500 mg/kg bw/day and all values were within the range of historical solvent control data. Based on these data the results of this micronucleus assay were considered to be negative. Male B6C3F1 mice (8/group) were administered a single i.p. injection of 0, 625, 1250 or 2500 mg/kg bw TiO2 (Unitane O-220) (Shelby and Witt 1995). The vehicle control was corn oil. A concurrent positive control was included, although the substance used was not specified. Mice were subcutaneously implanted with BrdU 18 hours prior to tissue collection to allow selection of first metaphase cells for scoring. Colchicine was injected i.p. two hours prior to tissue collection to arrest cells in metaphase. Mice were killed 17 or 36 hours after injection and femur bone marrow cells (50/animal/group) were scored for the presence of chromosomal aberrations. No increase in the incidence of chromosomal aberrations in bone marrow cells was observed at any dose compared with vehicle controls at either time point. The results of the chromosomal aberration assay were therefore considered to be negative. Supporting information – in vitro studies with food-grade TiO2 Information submitted to FSANZ following the call for data included unpublished reports of recently completed OECD TG compliant in vitro genotoxicity studies with food-grade TiO2. While in vivo studies are considered most relevant for assessing the genotoxicity of TiO2, the submitted in vitro studies were reviewed as supporting information given the limited number of in vivo studies using oral administration of food-grade TiO2. FSANZ is aware that additional in vitro studies with food-grade TiO2 (6 studies) or Unitane 0- 220 (four studies) are available in the published literature, reporting both positive and negative results. Health Canada’s review of TiO2 noted that none of these studies were conducted according to OECD test guidelines and the majority had significant deficiencies resulting in low confidence in the reliability and/or relevance of the findings (Health Canada 2022). In light of these limitations these studies were not considered further by FSANZ. No evidence of mutagenicity was observed in a bacterial reverse mutation assay with TiO2 (E171-E) conducted in compliance with OECD TG 471 (1997) and under GLP conditions in the presence and absence of metabolic activation (S9 mix) (Bioreliance 2021a). However, the bacterial reverse mutation assay may not be suitable for detecting genotoxicity induced by nanomaterials because the bacterial cells used lack the ability to perform endocytosis and diffusion of nanomaterials across the bacterial cell wall may be limited (Doak et al 2012; OECD 2014). In addition, TiO2 nanoparticles have been shown to possess antimicrobial properties (López de Castillo et al. 2020). In a guideline compliant (OECD TG 476, 2016), GLP in vitro mammalian cell forward mutation gene mutation assay, TiO2 (E171-E) was evaluated for its ability to induce mutations at the hypoxanthine-guanine phosphoribosyl transferase (HPRT) locus of Chinese Hamster Ovary (CHO) cells (Bioreliance 2021b). Tissue culture medium was used as the vehicle control and appropriate positive controls were included. Test substance dilutions were prepared and delivered to the test system with hood lights off. Based on observations of precipitation at concentrations ≥ 2.5 µg/mL in a preliminary toxicity assay, cultures treated with concentrations of 0.156 – 2.5 µg/mL and 0.0781 – 2.5 µg/mL were evaluated in the presence and absence of S9 metabolic activation, respectively. A follow up study assessed concentrations of 7.5, 15 and 30 µg/mL TiO2 with and without S9. No significant increases in mutant frequency compared with vehicle control were observed in either assay. All vehicle and control values were within acceptable ranges, confirming the validity of the assay. It was concluded that TiO2 (E171-E) was not mutagenic under the conditions of this study. Samples of CHO cells cultured with 30 µg/mL TiO2 (E171-E) in the presence and absence of 15 metabolic activation in this study were sent for transmission electron microscopy (TEM) analysis. No cellular uptake of the test item was observed. TiO2 (E171-E) was tested for the potential to induce micronuclei in human peripheral blood lymphocytes (HPBL) in the presence and absence of metabolic activation (S9 mix) (Bioreliance 2021c). The vehicle control was water and appropriate positive controls were included. The study was conducted in compliance with OECD TG 487 (2016) and under GLP conditions. HPBL were treated for four hours in the presence or absence of S9, washed and then cultured for a further 20 hours in the presence of cytochalasin B. Cells were also treated for 24 hours without metabolic activation in the presence of cytochalasin B. All treatment steps were conducted under strict avoidance of direct illumination or under red light. A preliminary toxicity assay found no evidence of cytotoxicity, but based on the presence of visible precipitate concentrations ranging from 0.3 – 30 µg/mL were selected for the micronucleus assay. No cytotoxicity was observed in the micronucleus assay and there were no increases in the frequency of micronucleated cells compared with the vehicle controls. Results with vehicle and positive controls were within the laboratory’s historical control data range, confirming the validity of the test system. It was concluded that TiO2 was not clastogenic or aneugenic under the conditions of this study. No information on whether cellular uptake of the test item occurred was available for this study. In an unpublished study, cellular uptake of various TiO2 particles including E171-E was investigated in vitro (Evans et al 2021). The test materials were incubated with TK6 (human lymphoblast) and A549 (human lung epithelium) cells at concentrations ranging from 1 – 100 µg/cm2, and cellular uptake was assessed by TEM in cells treated with 10 µg/cm2 of each test item (100 µg/cm2 resulted in structural abnormalities in the sections prepared for TEM so this concentration was not used for uptake analysis). No cytotoxicity was observed with any of the test items in either cell line. Cellular uptake of all test materials, including E171-E, was observed in A549 cells. In all cases the material was localised within the cytoplasm, and none of the test materials were detected in the nucleus. Only one non-food-grade TiO2 material was internalised by TK6 cells. Again, this material was found in the cytoplasm but not the nucleus. Flow cytometry analysis of the interaction of E171-E with cells (either internally or at the cell surface) found that interactions only occurred with a small proportion of the cell populations (~ 6.7% of A549 cells and ~ 31% of TK6 cells). Summary No in vivo genotoxicity studies with food-grade TiO2 administered via the diet are available. No DNA damage was observed in the two comet assays with food-grade TiO2 administered by oral gavage, and micronucleus and chromosomal aberration studies with Unitane 0-220 administered by the intraperitoneal route were also negative. Recent GLP- and OECD test guideline-compliant in vitro studies with food-grade TiO2 also found no evidence of mutagenicity in mammalian cells, and no evidence of clastogenicity or aneugenicity in a micronucleus assay with human peripheral blood lymphocytes. Transmission electron microscopy analysis of CHO cells used in the in vitro mutagenicity assay found no evidence of cellular uptake, while information on cellular uptake was not available for the in vitro micronucleus assay. In vitro studies of food-grade TiO2 found no evidence of cellular uptake in TK6 cells. Uptake and internalisation by A549 cells was observed, but no particles were detected in the nucleus. The absence of confirmed cellular uptake in the in vitro genotoxicity studies may limit confidence in the negative results, although alternatively the absence of direct exposure of the nucleus to food-grade TiO2 in these studies may indicate a low intrinsic hazard from a direct genotoxicity perspective (OECD 2014). 16 3.2.3 Developmental and reproductive toxicity EFSA’s review in 2016 noted a lack of adequate data on developmental and reproductive toxicity, and recommended that an EOGRT study be performed. Details of this study are available in EFSA’s 2021 review and summarised below. TiO2 (E171-E) was administered via the diet at doses of 0, 100, 300 or 1000 mg/kg bw/day to male and female rats of the F0 generation from 10 weeks prior to mating until weaning of the F1 generation (LPT 2020). The F1 generation was administered the same doses from weaning until postnatal day (PND) 4 or 8 of the F2 generation, and the F2 generation was exposed through the milk until PND 4 or 8, at which point the study was terminated. Small dose-related increases in titanium concentrations were observed in blood and urine in treated animals, including in the F2 cohort at PND 4-7 indicating exposure via placenta and possibly milk. No treatment-related clinical signs were observed, with the exception of pale faeces in F0 males and females at 300 and 1000 mg/kg bw/day, and at 1000 mg/kg bw/day in F1 males and females. There were no treatment-related adverse effects on haematology, clinical chemistry and urinalysis parameters, thyroid hormones, sex hormones, gross pathology or histopathology (F0 and F1 generations). No adverse effects on reproduction (F0 and F1 generations) or development (F0, F1 and F2 generations) were observed. An evaluation of neurodevelopmental and neurofunctional endpoints also found no adverse effects. The no observed adverse effect level (NOAEL) in this study was 1000 mg/kg bw/day, the highest dose tested. Rodríguez-Escamilla et al (2019) administered food-grade TiO2 (from Sensient) to male BALB/c mice (4/group) via the diet for 7 weeks at concentrations of 0, 0.1, 0.5 and 1%, resulting in calculated doses of 102, 682 or 1379 mg/kg bw/day for the treated groups, respectively. An additional group was administered 0 or 5 mg/kg bw/day E171 suspended in drinking water by oral gavage for 10 weeks. An increase in germ cell sloughing was observed in the testis in animals exposed to TiO2 via the diet (high dose only) and by gavage. Inflammatory cell infiltration was observed in the seminiferous tubules, as well as changes in markers of blood-testis barrier integrity, suggesting disruption of this barrier. Germinal epithelium cell detachment was observed following dietary exposure at all three doses without a clear dose-response, and this change was not observed following gavage administration. The effects in the testis were similar following both modes of administration despite the much larger doses administered by the diet, leading the authors to suggest that exposure via the feed may result in reduced systemic absorption compared with gavage administration. Similar changes were not observed in the testis in a follow-up study, in which food-grade TiO2 was administered to male C57BL/6 mice (9/group) via the drinking water at 0 or 5 mg/kg bw/day for 16 weeks (Medina-Reyes et al 2020). No effects on sperm concentration, spermatozoa motility or morphology were observed in this study. Summary No evidence of developmental or reproductive toxicity was observed in the EOGRT study with food-grade TiO2 and the NOAEL was 1000 mg/kg bw/day, the highest dose tested. While Rodríguez-Escamilla et al (2019) reported germ cell sloughing in the testis in mice administered food-grade TiO2 by oral gavage and in the diet, similar changes were not observed in a follow-up study, and no histopathological changes were seen in the testis in the EOGRT study summarised above, the 100-day dietary toxicity study by Blevins et al (2019), or in a 90-day toxicity study conducted in accordance with OECD TG 408 in which 17 food-grade sonicated TiO2 was administered by gavage at doses up to 1000 mg/kg bw/day (Han et al 2021; summarised in section 3.2.1). 3.2.4 Immunotoxicity A concern raised by EFSA (2021) was that some findings regarding immunotoxicity with TiO2 may be indicative of adverse effects. FSANZ identified three studies in which food-grade TiO2 administered via the diet was assessed for potential immune effects. In addition, a test guideline-compliant sub-chronic toxicity study evaluated the effects of gavage administration of food-grade TiO2 on a number of immune parameters. In the EOGRT study with TiO2 (E171-E), effects on developmental immunotoxicity were assessed in a cohort of the F1 generation (10/sex/group; PND 53 – 61) by examination of the ability to raise an antibody response to a sensitising antigen, the keyhole limpet haemocyanin (KLH) antigen (LPT 2020). Animal were killed 5 days after i.v. tail vein injection of KLH and the level of anti-KLH IgM antibodies measured in serum. Satellite F1 animals (10/sex; PND 55) were immunised with KLH and treated with the immunosuppressant cyclophosphamide as a positive control. A slight decrease (~ 9%) in the KLH-specific IgM level was found in the high dose males (1000 mg/kg bw/day), but without an apparent dose- response. The positive control group was not treated at the same time as the other F1 animals, however, so the results of the KLH assay were not considered valid and the sensitivity of the assay could not be confirmed. Haematology, spleen weight and histopathology of lymphoid organs animals from the F1 cohort found no treatment-related adverse effects. Analysis of lymphocyte subpopulations in the spleen found no treatment- related changes in the percentage of T cells, T helper cells, T suppressor/cytotoxic cells, NK cells and B cells in animals of either sex. The study by Blevins et al (2019) included an assessment of the effects of dietary exposure to food-grade TiO2 at doses up to 267 mg/kg bw/day for 7 or 100 days on several immune parameters. No treatment-related effects on the frequency of CD103+ dendritic cells, CD4+ T helper cells or total or activated T regulatory cells were observed in Peyer’s patches, spleen or whole blood leukocytes. Inflammatory cytokine/chemokine levels were not significantly altered in plasma, or sections of the small intestine (jejunum) and colon. No treatment-related histopathological changes were observed in the spleen or small and large intestines. In a study by Riedle et al (2020), administration of food-grade TiO2 in the diet to C57BL/6 mice at doses up to 100 mg/kg bw/day for 18 weeks had no effects on the profile of immune cells (CD4 or CD8 T cells, B cells or phagocytes) in the Peyer’s patches. In a 90-day OECD TG 408-compliant toxicity study in which food-grade sonicated TiO2 was administered by gavage to rats at doses up to 1000 mg/kg bw/day, no adverse effects were observed on a range of immune parameters (Han et al 2021). No changes in immune organ weights or histology were observed. A slight, statistically significant reduction in the proportion of lymphocytes (~8%) compared with controls was observed at the low and high dose in male but not female rats. Levels of granulocyte-macrophage colony-stimulating factor (GM-CSF) were reduced in high dose females and IgM was reduced in high dose males and females, without a clear dose-response. Given the lack of a dose-response, the small magnitude of the changes and the known natural variability in these parameters these changes were not considered to be adverse. Summary Studies of food-grade TiO2 administered via dietary administration found no evidence of adverse immunological effects in rats or mice. In addition, an OECD TG-compliant study found no treatment-related adverse effects on immune parameters in rats administered 18 sonicated food-grade TiO2 by gavage at doses up to 1000 mg/kg bw/day for 90 days. Although the positive control in the cohort assessed for developmental immunotoxicity in the EOGRT study was not considered valid, no evidence of developmental immunotoxicity was observed with TiO2 in this study. 3.2.5 Neurotoxicity EFSA’s 2021 review also noted that some findings regarding neurotoxicity with TiO2 nanoparticles may be indicative of adverse effects. FSANZ was only able to identify one study in which neurotoxicity was examined using food-grade TiO2 administered via the diet. As part of the EOGRT study with TiO2 (LPT 2020), qualitative and quantitative neurohistopathological examinations were performed in animals of both sexes from the F1 generation with no changes indicative of neurotoxicity being found. No adverse effects were observed on neurofunctional endpoints including auditory startle response and a functional observational battery. 4. Discussion and conclusions Concerns about the safety of TiO2 as a food additive are primarily based on findings in studies with TiO2 test items completely in the nanoscale, or studies in which food-grade TiO2 was dispersed in solution by sonication and administered by oral gavage or via drinking water. The relevance of such studies for assessing the human health risk from dietary exposure to food-grade TiO2 is limited because TiO2 in food is not sonicated, there is no evidence that TiO2 in foodstuffs de-agglomerates in the gastrointestinal tract and exposure to TiO2 via gavage or drinking water does not take into consideration food matrix effects. Studies using unsonicated food-grade TiO2 administered via the diet were therefore the primary focus of this review. The available evidence from animal and human studies suggests that absorption of TiO2 following oral exposure is very limited. Newly available studies with food-grade TiO2 submitted to FSANZ indicates the relative oral bioavailability in rats is ≤ 0.0013%. Given the age of the subjects in the available studies with human cadavers, TiO2 levels in the tissue can be expected to have achieved steady state, and these studies indicate relatively low bioavailability. FSANZ’s previous review of TiO2 noted that in humans, the presence of inorganic micro- particles containing TiO2 and other sub-micron particles in basal ‘pigmented cells’ of Peyer’s patches is a normal occurrence throughout Western populations. These particle-containing pigmented cells are mainly mature macrophages that appear to be of low metabolic and immunological activity, with no evidence for differential phenotype or activation In vivo genotoxicity studies using dietary administration of food-grade TiO2 are currently lacking. However, there is no evidence that food-grade TiO2 administered by other modes was genotoxic in vivo. Oral gavage studies with food-grade TiO2 and i.p. studies with Unitane 0-220, representative of food-grade TiO2, found no evidence of DNA damage, clastogenicity or aneugenicity. In addition, GLP- and test guideline-compliant in vitro studies with food- grade TiO2 evaluated as supporting information also found no evidence of genotoxicity. Additional GLP- and test guideline-compliant in vivo genotoxicity (e.g. mutagenicity and micronucleus) studies with food-grade TiO2 would be valuable to confirm this conclusion. 19 While pre-neoplastic lesions in the colon were observed in a drinking water study with sonicated food-grade TiO2 at 10 mg/kg bw/day, these findings were not replicated in two studies in which food-grade TiO2 was administered via the diet up to considerably higher doses (up to 267 or 1000 mg/kg bw/day). Observations of pre-neoplastic lesions are also inconsistent with the findings of a 2-year bioassay of TiO2 in rats and mice conducted by the US NCI. No evidence of toxicity or carcinogenicity was observed at dietary concentrations up to 50,000 ppm in this study. A recent OECD TG-compliant EOGRT study in rats with food-grade TiO2 administered via the diet at doses up to 1000 mg/kg bw/day found no evidence of systemic toxicity, developmental or reproductive toxicity or developmental neurotoxicity. Although the positive control in the cohort assessed for developmental immunotoxicity was not considered valid, no evidence of developmental immunotoxicity was observed with TiO2 in this study. Based on the data currently available, FSANZ concludes there is no evidence to suggest that dietary exposures to food-grade TiO2 are of concern for human health. 20 5. References ANSES (Agence nationale de sécurité sanitaire de l’alimentation, de l’environnement et du travail) 2017. Groupe d’expertise collective en urgence « TiO2». Expert Committee commissioned by ANSES. Procès-verbal de la reunion du « 24 mars 2017». Available online at: https://www.anses.fr/fr/system/files/GECU-TiO2-PV-2017-03-24.pdf Accessed 28 July 2022 Bettini S, Boutet-Robinet E, Cartier C, Coméra C, Gaultier E, Dupuy J, Naud N, Taché S, Grysan P, Reguer S, Thieriet N, Réfrégiers M, Thiaudière D, Cravedi JP, Carrière M, Audinot JN, Pierre FH, Guzylack-Piriou L, Houdeau E (2017) Food-grade TiO 2 impairs intestinal and systemic immune homeostasis, initiates preneoplastic lesions and promotes aberrant crypt development in the rat colon. Sci Rep 7:40373. BioReliance (2021a) Bacterial reverse mutation assay. Titanium dioxide E171-E. BioReliance Corporation, Rockville, MD, USA. BioReliance (2021b) In vitro mammalian cell forward gene mutation (CHO/HPRT) assay with duplicate cultures. Titanium dioxide E171-E. BioReliance Corporation, Rockville, MD, USA. BioReliance (2021c) In vitro mammalian cell micronucleus assay in human peripheral blood lymphocytes (HPBL). Titanium dioxide E171-E. BioReliance Corporation, Rockville, MD, USA. Blevins LK, Crawford RB, Bach A, Rizzo MD, Zhou J, Henriquez JE, Khan DMIO, Sermet S, Arnold LL, Pennington KL, Souza NP, Cohen SM, Kaminski NE (2019) Evaluation of immunologic and intestinal effects in rats administered an E171-containing diet, a food grade titanium dioxide (TiO2). Food Chem Toxicol 133:110793. Böckmann J, Lahl H, Eckert T, Unterhalt B (2000) Blood titanium levels before and after oral administration titanium dioxide. [Article in German] Pharmazie 55(2):140-3. Charles S, Jomini S, Fessard V, Bigorgne-Vizade E, Rousselle C, Michel C (2018) Assessment of the in vitro genotoxicity of TiO2 nanoparticles in a regulatory context. Nanotoxicology 12(4):357-374. Clapper ML, Chang WL, Cooper HS (2019) Dysplastic Aberrant Crypt Foci: Biomarkers of Early Colorectal Neoplasia and Response to Preventive Intervention. Cancer Prev Res 13(3):229-240. COT (2022) Interim position paper on titanium dioxide. Committee on Toxicity of Chemicals in Food, Consumer Products and the Environment, UK. Available at: https://cot.food.gov.uk/2021-statementsandpositionpapers Accessed 28 June 2022. Disdier C, Devoy J, Cosnefroy A, Chalansonnet M, Herlin-Boime N, Brun E, Lund A, Mabondzo A (2015) Tissue biodistribution of intravenously administrated titanium dioxide nanoparticles revealed blood-brain barrier clearance and brain inflammation in rat. Part Fibre Toxicol 12:27. Doak SH, Manshian B, Jenkins GJ, Singh N (2012) In vitro genotoxicity testing strategy for nanomaterials and the adaptation of current OECD guidelines. Mutat Res 745(1-2):104-11. 21 Dudefoi W, Rabesona H, Rivard C, Mercier-Bonin M, Humbert B, Terrisse H, Ropers MH (2021) In vitro digestion of food grade TiO 2 (E171) and TiO 2 nanoparticles: physicochemical characterization and impact on the activity of digestive enzymes. Food Funct 12(13):5975-5988. Dudefoi W, Terrisse H, Richard-Plouet M, Gautron E, Popa F, Humbert B, Ropers MH (2017) Criteria to define a more relevant reference sample of titanium dioxide in the context of food: a multiscale approach. Food Addit Contam Part A Chem Anal Control Expo Risk Assess 34(5):653-665. EFSA (2016) Re-evaluation of titanium dioxide (E 171) as a food additive. EFSA Panel on Food Additives and Nutrient Sources added to Food (ANS). EFSA Journal 14(9):4545, 83 pp. EFSA (2018) Scientific Opinion on the evaluation of four new studies on the potential toxicity of titanium dioxide used as a food additive (E 171). EFSA Journal 16(7):5366, 27 pp. EFSA (2019) Scientific opinion on the proposed amendment of the EU specifications for titanium dioxide (E 171) with respect to the inclusion of additional parameters related to its particle size distribution. EFSA Panel on Food Additives and Flavourings (FAF). EFSA Journal 17(7):5760, 23 pp. EFSA (2021) Safety assessment of titanium dioxide (E 171) as a food additive. EFSA Panel on Food Additives and Flavourings (FAF). EFSA Journal 19(5):6585, 130 pp. Evans SJ, Clift MJD, Doak SH (2021) Evaluating the in vitro cellular uptake of titanium dioxide. In Vitro Toxicology Group, Swansea University Medical School, Wales, UK. Farrell TP, Magnuson B (2017) Absorption, Distribution and Excretion of Four Forms of Titanium Dioxide Pigment in the Rat. J Food Sci 82(8):1985-1993. Furukawa F, Nishikawa A, Kitahori Y, Tanakamaru Z, Hirose M (2002) Spontaneous development of aberrant crypt foci in F344 rats. J Exp Clin Cancer Res 21(2):197-201. Geraets L, Oomen AG, Krystek P, Jacobsen NR, Wallin H, Laurentie M, Verharen HW, Brandon EF, de Jong WH (2014) Tissue distribution and elimination after oral and intravenous administration of different titanium dioxide nanoparticles in rats. Part Fibre Toxicol 11:30. Han HY, Yang MJ, Yoon C, Lee GH, Kim DW, Kim TW, Kwak M, Heo MB, Lee TG, Kim S, Oh JH, Lim HJ, Oh I, Yoon S, Park EJ (2021) Toxicity of orally administered food-grade titanium dioxide nanoparticles. J Appl Toxicol 41(7):1127-1147. Health Canada (2022) State of the science of titanium dioxide (TiO2) as a food additive. Food Directorate, Health Canada, Ottawa, Canada. Available at: https://www.canada.ca/en/health- canada/services/food-nutrition/reports-publications/titanium-dioxide-food-additive-science- report.html Accessed 28 June 2022. Heringa MB, Peters RJB, Bleys RLAW, van der Lee MK, Tromp PC, van Kesteren PCE, van Eijkeren JCH, Undas AK, Oomen AG, Bouwmeester H (2018) Detection of titanium particles in human liver and spleen and possible health implications. Part Fibre Toxicol 15(1):15. Jensen DM, Løhr M, Sheykhzade M, Lykkesfeldt J, Wils RS, Loft S, Møller P (2019) Telomere length and genotoxicity in the lung of rats following intragastric exposure to food- grade titanium dioxide and vegetable carbon particles. Mutagenesis 34(2):203-214. 22 Jones K, Morton J, Smith I, Jurkschat K, Harding AH, Evans G (2015) Human in vivo and in vitro studies on gastrointestinal absorption of titanium dioxide nanoparticles. Toxicol Lett 233(2):95-101. Kreyling WG, Holzwarth U, Haberl N, Kozempel J, Hirn S, Wenk A, Schleh C, Schäffler M, Lipka J, Semmler-Behnke M, Gibson N (2017a) Quantitative biokinetics of titanium dioxide nanoparticles after intravenous injection in rats: Part 1. Nanotoxicology 11(4):434-442. Kreyling WG, Holzwarth U, Schleh C, Kozempel J, Wenk A, Haberl N, Hirn S, Schäffler M, Lipka J, Semmler-Behnke M, Gibson N (2017b) Quantitative biokinetics of titanium dioxide nanoparticles after oral application in rats: Part 2. Nanotoxicology 11(4):443-453. Kristiansen E (1996) The role of aberrant crypt foci induced by the two heterocyclic amines 2-amino-3-methyl-imidazo[4,5-f]quinoline (IQ) and 2-amino-1-methyl-6-phenyl-imidazo[4,5- b]pyridine (PhIP) in the development of colon cancer in mice. Cancer Lett 110(1-2):187-92. López de Dicastillo, Carol & Correa, Matías & Martínez, Fernanda & Streitt, Camilo & Galotto, Maria. (2020) Antimicrobial effect of titanium dioxide nanoparticles. 10.5772/intechopen.90891. LPT (Laboratory Pharmacology and Toxicology), 2020. Interim Report - EOGRT study of Titanium dioxide E171-E in rats by oral administration via the diet. Report No. 36222. Submitted by the European Commission on 27 May 2020; updated version of the report submitted by Titanium Dioxide Manufacturers Association on 1st September 2020. Details available in EFSA (2021). Magnuson BA, Carr I, Bird RP (1993) Ability of aberrant crypt foci characteristics to predict colonic tumor incidence in rats fed cholic acid. Cancer Res 53(19):4499-504. Medina-Reyes EI, Delgado-Buenrostro NL, Díaz-Urbina D, Rodríguez-Ibarra C, Déciga- Alcaraz A, González MI, Reyes JL, Villamar-Duque TE, Flores-Sánchez ML, Hernández- Pando R, Mancilla-Díaz JM, Chirino YI, Pedraza-Chaverri J (2020) Food-grade titanium dioxide (E171) induces anxiety, adenomas in colon and goblet cells hyperplasia in a regular diet model and microvesicular steatosis in a high fat diet model. Food Chem Toxicol 146:111786. Naegeli H, Gsell C (2022) "Nano-ghosts": Risk assessment of submicron-sized particles in food biased towards fictional "nano". EXCLI J 21:279-299. NCI (1979) Bioassay of titanium dioxide for possible carcinogenicity. National Cancer Institute Carcinogenesis Technical Report Series No. 97. NCI-CG-TR-97. OECD (2014) Genotoxicity of manufactured nanomaterials: report of the OECD expert meeting. Series on the safety of manufactured nanomaterials, No. 43. ENV/JM/MONO(2014)34. Organisation for Economic Co-operation and Development, Paris. Pele LC, Thoree V, Bruggraber SF, Koller D, Thompson RP, Lomer MC, Powell JJ (2015) Pharmaceutical/food grade titanium dioxide particles are absorbed into the bloodstream of human volunteers. Part Fibre Toxicol 12:26. Peters RJB, Oomen AG, van Bemmel G, van Vliet L, Undas AK, Munniks S, Bleys RLAW, Tromp PC, Brand W, van der Lee M (2020) Silicon dioxide and titanium dioxide particles found in human tissues. Nanotoxicology 14(3):420-432. 23 Pretlow TP, O'Riordan MA, Somich GA, Amini SB, Pretlow TG (1992) Aberrant crypts correlate with tumor incidence in F344 rats treated with azoxymethane and phytate. Carcinogenesis 13(9):1509-12. Provivo Biosciences (2022) Relative oral bioavailability of 5 different TiO2 grades. Provivo Biosciences, Hamburg, Germany. Quintanilla I, López-Cerón M, Jimeno M, Cuatrecasas M, Zabalza M, Moreira L, Alonso V, Rodríguez de Miguel C, Muñoz J, Castellvi-Bel S, Llach J, Castells A, Balaguer F, Camps J, Pellisé M (2019) Rectal aberrant crypt foci in humans are not surrogate markers for colorectal cancer risk. Raju J, Sondagar C, Roberts J, Aziz SA, Caldwell D, Vavasour E, Mehta R (2011) Dietary acrylamide does not increase colon aberrant crypt foci formation in male F344 rats. Food Chem Toxicol 49(6):1373-80. Riedle S, Wills JW, Miniter M, Otter DE, Singh H, Brown AP, Micklethwaite S, Rees P, Jugdaohsingh R, Roy NC, Hewitt RE, Powell JJ (2020) A murine oral-exposure model for nano- and micro-particulates: demonstrating human relevance with food-grade titanium dioxide. Small 16(21):e2000486. Rodríguez-Escamilla JC, Medina-Reyes EI, Rodríguez-Ibarra C, Déciga-Alcaraz A, Flores- Flores JO, Ganem-Rondero A, Rodríguez-Sosa M, Terrazas LI, Delgado-Buenrostro NL, Chirino YI (2019) Food-grade titanium dioxide (E171) by solid or liquid matrix administration induces inflammation, germ cells sloughing in seminiferous tubules and blood-testis barrier disruption in mice. J Appl Toxicol 39(11):1586-1605. Shelby MD, Erexson GL, Hook GJ, Tice RR (1993) Evaluation of a three-exposure mouse bone marrow micronucleus protocol: results with 49 chemicals. Environ Mol Mutagen 21(2):160-79. Shelby MD, Witt KL (1995) Comparison of results from mouse bone marrow chromosome aberration and micronucleus tests. Environ Mol Mutagen 25(4):302-13. Singh N, Dev I, Pal S, Yadav SK, Idris MM, Ansari KM (2022) Transcriptomic and proteomic insights into patulin mycotoxin-induced cancer-like phenotypes in normal intestinal epithelial cells. Mol Cell Biochem 477(5):1405-1416 Taurozzi JS, Hackley VA, Wiesner MR (2011) Ultrasonic dispersion of nanoparticles for environmental, health and safety assessment--issues and recommendations. Nanotoxicology 5(4):711-29. TDMA (2022) Comparison of current food grade titanium dioxide (E171) with historical samples of Unitane O-220. Titanium Dioxide Manufacturers Association, Brussels, Belgium. Tennant RW, Margolin BH, Shelby MD, Zeiger E, Haseman JK, Spalding J, Caspary W, Resnick M, Stasiewicz S, Anderson B, et al (1987) Prediction of chemical carcinogenicity in rodents from in vitro genetic toxicity assays. Science 236(4804):933-41. Thoree V, Skepper J, Deere H, Pele LC, Thompson RP, Powell JJ (2008) Phenotype of exogenous microparticle-containing pigment cells of the human Peyer's patch in inflamed and normal ileum. Inflamm Res 57(8):374-8. ToxConsult (2016) Potential health risks associated with nanotechnologies in existing food additives. A report prepared by ToxConsult Pty Ltd for Food Standards Australia New 24 Zealand.. https://www.foodstandards.gov.au/publications/Documents/Safety%20of%20nanotechnology %20in%20food.pdf Accessed 5 April 2022 Verleysen E, Waegeneers N, Brassinne F, De Vos S, Jimenez IO, Mathioudaki S, Mast J (2020) Physicochemical Characterization of the Pristine E171 Food Additive by Standardized and Validated Methods. Nanomaterials 10(3):592. Verleysen E, Waegeneers N, De Vos S, Brassinne F, Ledecq M, Van Steen F, Andjelkovic M, Janssens R, Mathioudaki S, Delfosse L, Machiels R, Cheyns K, Mast J (2021) Physicochemical characterization of nanoparticles in food additives in the context of risk identification. EFSA supporting publication. 2021:EN-6678. 31 pp. Weir A, Westerhoff P, Fabricius L, Hristovski K, von Goetz N (2012) Titanium dioxide nanoparticles in food and personal care products. Environ Sci Technol 46(4):2242-50. West B, Wyzan H (1963) Unpublished report by American Cyanamid Company. WHO (1969) Toxicological evaluation of some food colours, emulsifiers, stabilizers, anti- caking agents and certain other substances. FAO Nutrition Meetings Report Series No. 46A WHO/FOOD ADD/70.36 https://inchem.org/documents/jecfa/jecmono/v46aje19.htm Accessed 5 April 2022 Winkler HC, Notter T, Meyer U, Naegeli H (2018) Critical review of the safety assessment of titanium dioxide additives in food. J Nanobiotechnology 16(1):51. 25 Appendix 1: Independent literature search Overall search strategy Relevant data on the safety of TiO2 as a food additive was identified from publically available literature sources, using a PECOS question to define study inclusion criteria. This method was based on the PECO concepts outlined in (Hoffmann et al., 2017; JECFA, 2017; OHAT, 2019). FSANZ pre-defined PECOS study criteria were: Population Included: Humans without age restriction Mammalian model organisms without age restriction Excluded: Non-mammalian model organisms In vitro studies using mammalian cells/tissues Exposure Included: All food grade titanium dioxide (Sample) All titanium dioxide test samples (inc. nano particles) Rutile or anatase titanium dioxide Excluded: Titanium dioxide nanoparticles with an average diameter < 30 nm10 Titanium dioxide nanotubes Titanates (FeTiO3, H4TiO4) Titanium dioxide nanofibers Exposure Included: Ingestion (feed, drinking water, gavage) (Type) Intravenous injection (toxicokinetic & genotoxicity studies only) Excluded: Dermal contact Other Injection (e.g. intraperitoneal11, subcutaneous) Inhalation Control Included: Any comparator Outcome Included: General signs of toxicity (weight loss, etc.) Genotoxicity Carcinogenicity Developmental toxicity Reproductive toxicity Immunotoxicity Inflammatory response Neurotoxicity 10 EFSA’s review noted that particles < 30 nm amounted to less than 1% of food-grade TiO by number. Studies 2 with TiO2 nanoparticles < 30 nm were therefore considered to be of limited relevance. 11 Although intraperitoneal administration was excluded from the PECO criteria, two in vivo genotoxicity studies with a test item representative of food-grade TiO2 were included in the review due to the limited number of in vivo studies with food-grade TiO2. 26 Pharmacokinetics Study Design Included: Study reports conducted according to OECD Test guidelines Reports detailing OECD GLP compliant studies Peer reviewed original research Study reports of original research Excluded: Reports lacking original data Abstracts, conference presentations, posters, and editorials Search parameters Using the PECOS inclusion criteria, search queries were formulated for the Web of Science12 (WoS; Clarivate, USA) and PubMed13 (National Library of Medicine, USA) scientific literature databases. Web of Science: Search conducted on 29/6/2021 References identified using search strategy: 1161 Search Parameters: Search language = English; Databases = WOS, MEDLINE; Timespan = All years Search queries: [#1]: TI=("titanium dioxide" OR TiO2 OR "TiO 2" OR E171 OR "E 171" OR Anatase OR Rutile OR Nanoanatase OR Nanotitanium) [#2]: TI=(safe* OR pharmaco* OR toxi* OR aneugen* OR aneuploid* OR (chromosom* AND aberration*) OR clastogen* OR “DNA adduct*” OR “DNA damage*” OR “DNA strand break*” OR “gene mutation*” OR “genetic damage*” OR “genetic toxic*” OR “genetic toxic*” OR genotox* OR safe* OR adverse OR carcinogen* OR inflammat* OR microinflammat* OR neuroinflammat* OR proinflammat* OR immunotox* OR reproducti* OR reprotox*) [#3]: AB=(aneugen* OR aneuploid* OR (chromosom* AND aberration*) OR clastogen* OR “DNA adduct*” OR “DNA damage*” OR “DNA strand break*” OR “gene mutation*” OR “genetic damage*” OR “genetic toxic*” OR “genetic toxic*” OR genotox* OR safe* OR adverse OR carcinogen* OR inflammat* OR microinflammat* OR neuroinflammat* OR proinflammat* OR immunotox* OR reproducti* OR reprotox*) [#4]: AB=(laser OR catalys* OR sunscreen* OR solar OR "visible light" OR "visible lights" OR batter* OR electr* OR cosmeti* OR diode OR electron OR voltage OR nanotubes OR photo*) 12 Available at: https://www.webofscience.com 13 Available at: https://pubmed.ncbi.nlm.nih.gov 27 [#5]: TI=(dermal OR skin OR inhal* OR lung OR plant OR aquatic OR algae OR fung* OR fish OR effluent OR ecology) [#6]: #2 OR #3 [#7]: #1 AND #6 [#8]: #7 NOT #4 [#9]: #8 NOT #5 PubMed: Search conducted on 30/6/2021 References identified using search strategy: 1303 Search query: ("titanium dioxide"[Supplementary Concept] OR "titanium dioxide"[tiab] OR TiO2[tiab] OR "TiO 2"[tiab] OR E171[tiab] OR "E 171"[tiab] OR anatase[tiab] OR rutile[tiab] OR nanoanatase[tiab] OR nanotitanium[tiab]) AND "english"[Language] AND ((aneugen*[tiab] OR aneuploid*[tiab] OR (chromosom*[tiab] AND aberration*[tiab]) OR clastogen*[tiab] OR “DNA adduct*”[tiab] OR “DNA damage*”[tiab] OR “DNA strand break*”[tiab] OR “gene mutation*”[tiab] OR “genetic damage*”[tiab] OR “genetic toxic*”[tiab] OR “genetic toxic*”[tiab] OR genotox*[tiab] OR micronucle*[tiab] OR mutagen*[tiab] OR mutation*[tiab] OR polyploid*[tiab] OR safe*[tiab] OR adverse[tiab] OR inflammat*[tiab] OR microinflammat*[tiab] OR neuroinflammat*[tiab] OR proinflammat*[tiab] OR immunotox*[tiab] OR reproducti*[tiab] OR reprotox*[tiab]) OR ("Toxicity Tests"[mesh] OR "In Vitro Techniques"[mesh] OR "Toxic Actions"[mesh] "Toxicological Phenomena"[mesh] OR "Pharmacokinetics"[mesh] OR "Toxicokinetics"[mesh] OR "Food additives"[mesh] OR "Cell Survival"[mesh] OR "Allergy and Immunology"[mesh] OR "Cytotoxins"[mesh] OR "Endocrine disruptors"[mesh] OR "Hormones"[mesh] OR "Hypersensitivity"[mesh] OR "Immunotoxins"[mesh])) NOT (laser[tiab] OR catalys*[tiab] OR sunscreen*[tiab] OR "visible light"[tiab] OR "visible lights"[tiab] OR batter*[tiab] OR electr*[tiab] OR cosmeti*[tiab] OR diode[tiab] OR electron[tiab] OR voltage[tiab] OR nanotubes[tiab] OR photo*[tiab] OR Editorial[Publication Type] OR Review[Publication Type] OR News[Publication Type] OR Comment[Publication Type] OR dermal[ti] OR skin[ti] OR inhal*[ti] OR lung[ti] OR Plants[mesh] OR aquatic[ti] OR algae[ti] OR fung*[ti] OR fish[ti] OR effluent[ti] OR ecology[ti] OR "Ecological and Environmental Phenomena"[mesh] OR Ecology[mesh]) Study screening and selection Results collated from the WoS and PubMed search queries, resulted in 1931 unique references. Titles and abstracts of each reference were screened manually against the PECOS criteria and studies not meeting these criteria were excluded. Full-text copies of remaining studies were reviewed manually and assigned a score of reliability established using pre-determined criteria (Table A1). After full-text review, if studies were rated as tier 4 (not assignable/unusable), or determined to not meet the PECOS criteria, they were excluded from consideration. 28 From the studies remaining after the screening and selection process, the review focused on studies in which food-grade TiO2 was administered via the diet without prior sonication, reflecting human dietary exposures. In some cases, studies involving other modes of administration were taken into account as supporting evidence, as discussed in the relevant sections of this report. In addition to the studies identified in the literature search, several unpublished studies submitted in response to FSANZ’s call for data were included in the review. Table A1: Study Quality and Reliability Scores Tier 1 (Reliable without Restriction) Performed according to GLP or equivalent OECD TG methodology or equivalent, with detailed documentation Adequately described methodology, results and analysis Food grade TiO2 (administered orally for in vivo studies) Tier 2 (Reliable with Restriction) OECD TG methodology or equivalent study, without detailed documentation OECD TG methodology or equivalent study, with acceptable restrictions Test procedure in accordance with national standard methods with acceptable restrictions Well documented study meeting accepted scientific principles and is suitable for assessment Food grade TiO2 (administered orally for in vivo studies) Tier 3 (Not Reliable - only consider for weight of evidence) Significant methodological deficiencies Does not meet acceptability criteria Unsuitable system (Poorly reflective of TiO2 as a food additive) Uses TiO2 that is not food grade (or was not administered orally for in vivo studies) Laboratory has not demonstrated proficiency in the assay Tier 4 (Not assignable/usable) Documentation or study unsuitable for risk assessment *Based on the systematic approach for evaluating the quality of toxicological data as described by Klimisch et al. (1997) References Hoffmann, S., de Vries, R. B. M., Stephens, M. L., Beck, N. B., Dirven, H. A. A. M., Fowle, J. R., Goodman, J. E., Hartung, T., Kimber, I., Lalu, M. M., Thayer, K., Whaley, P., Wikoff, D., Tsaioun, K. (2017) A primer on systematic reviews in toxicology. Arch Toxicol. 91(7):2551- 2575. doi: 10.1007/s00204-017-1980-3. Joint FAO/WHO Expert Committee on Food Additives (2017) Guidance to JECFA Experts on Systematic Literature Searches. World Health Organisation. 29 https://www.who.int/publications/m/item/guidance-to-jecfa-experts-on-systematic-literature- searches. Accessed: 4th June 2021 Klimisch, H. J., Andreae, M., Tillmann, U. (1997) A systematic approach for evaluating the quality of experimental toxicological and ecotoxicological data. Regul Toxicol Pharmacol. 25(1):1-5. doi: 10.1006/rtph.1996.1076. Office of Health Assessment and Translation (2019). Handbook for Conducting a Literature- Based Health Assessment Using OHAT Approach for Systematic Review and Evidence Integration. National Toxicology Program, USA. https://ntp.niehs.nih.gov/go/ohathandbook. Accessed: 4th June 2021. 30