Fluid, Electrolyte Chapter 26 PDF
Document Details
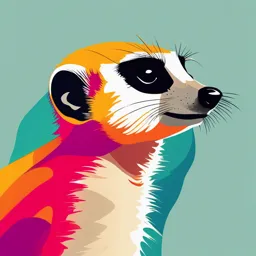
Uploaded by PanoramicCornet
University of Texas at El Paso
Tags
Summary
This document is a chapter on fluid and electrolytes, covering body water content, fluid compartments, composition, and comparison of extracellular and intracellular fluids. It's structured to be informative, detailing the various components of these systems.
Full Transcript
Body Water Content Total body water depends on age and body mass, but also on the relative amount of body fat % body water: – Infants: 73 or more (low body fat and bone mass) – Declines to 45 in old age – Adult males: 60 (generally more muscle mass, so higher % water)...
Body Water Content Total body water depends on age and body mass, but also on the relative amount of body fat % body water: – Infants: 73 or more (low body fat and bone mass) – Declines to 45 in old age – Adult males: 60 (generally more muscle mass, so higher % water) ▪ Skeletal muscle 75 water ▪ Adipose tissue less than 20% water (lowest of all tissues) – Adult females: 50 (generally higher fat content, less skeletal muscle mass) Copyright © 2025 Pearson Education, Inc. All Rights Reserved Fluid Compartments Water occupies fluid compartments within the body – Total body fluid (water) in adults averages 40 L – Two major fluid compartments: 1. Intracellular fluid (ICF) is inside of cells; 2 3 (25 L) of total body fluid 2. Extracellular fluid (ECF) is outside of cells; 13 (15 L) of total body fluid – Our body’s “internal environment” and our cells’ “external environment” – Two major ECF compartments: 1. Plasma is fluid part of blood (3 L) 2. Interstitial fluid (IF) fills the spaces between cells e.g. spaces between blood vessels and cells (12 L) – Other compartments include lymph, CSF, humors of the eye, synovial fluid, serous fluid, and gastrointestinal secretions Most are usually considered part of IF Copyright © 2025 Pearson Education, Inc. All Rights Reserved The Major Fluid Compartments of the Body Figure 26.1 The major fluid compartments of the body. Copyright © 2025 Pearson Education, Inc. All Rights Reserved Composition of Body Fluids Water is the universal solvent – Dissolved in water are solutes: electrolytes and nonelectrolytes – Water moves down an osmotic gradient—from area of lower osmolality (lower solute concentration) to area of higher osmolality Electrolytes and nonelectrolytes – Nonelectrolytes: molecules (mostly organic) that do not dissociate into charged particles in water; e.g., glucose, lipids, creatinine, urea – Electrolytes: compounds that dissociate into ions in water (and so conduct an electrical current); e.g., inorganic salts, acids and bases, some proteins ▪ Dissociate into at least two ions, so greater osmotic power than nonelectrolytes (greater ability to cause fluid shifts); for example: – Molecule of sodium chloride (NaCl → Na + Cl ) contributes 2 + − as many solute particles (2) as molecule of glucose (nonelectrolyte) glucose → glucose (one undissociated particle) – Molecule of magnesium chloride (MgCl → Mg2+ + 2Cl− ) contributes 3 Copyright © 2025 Pearson Education, Inc. All Rights Reserved Composition of Body Fluids – Electrolytes (cont.) inue d ▪ Electrolyte concentrations expressed in milliequivalents per liter (mEq / L), a measure of electrical charges per liter of solution ion concentration (mg / L) mEq / L = no.of electrical charges on one ion atomic weight of ion (mg / mmol) ▪ For single charged ions (Na+ ), 1 mEq = 1 mOsm ▪ For bivalent ions (Ca2 + ), 1 mEq = 1 mOsm 2 ▪ 1 mEq of either provides same amount of charge Copyright © 2025 Pearson Education, Inc. All Rights Reserved Composition of Body Fluids Comparison of extracellular and intracellular fluids – Each fluid compartment has a distinctive pattern of electrolytes ▪ Note: Na+ and K + concentrations in ECF and ICF are nearly opposite – Via ATP-dependent sodium potassium pumps – ECF fluids similar, except higher plasma protein levels (so less Cl− ) ▪ Major cation: Na+ / Major anion: Cl− – ICF (low concentration of Na+ and Cl− ) has 3 more soluble proteins than plasma ▪ Major cation: K + / Major anion: HPO42− – Electrolytes are most numerous solutes in body fluids ▪ Determine most chemical and physical reactions ▪ But proteins, phospholipids, cholesterol, and triglycerides constitute the greatest mass of dissolved solutes – 90% of mass in plasma, 60% in IF, and 97% in ICF Copyright © 2025 Pearson Education, Inc. All Rights Reserved Electrolyte Composition of Blood Plasma, Interstitial Fluid, and Intracellular Fluid Figure 26.2 Electrolyte composition of blood plasma, interstitial fluid, and intracellular fluid. Copyright © 2025 Pearson Education, Inc. All Rights Reserved Fluid Movement Among Compartments There is continuous exchange and mixing of fluids between compartments (via osmotic and hydrostatic pressures) – Water moves freely, between compartments, along osmotic gradients ▪ Many solutes cannot move freely because of their size or charge ▪ Any difference in solute concentration that develops between compartments causes net water movement (so differences don’t last long) Exchanges between plasma and IF occur across capillary walls – Fluid leaks from arteriolar end of capillary (most reabsorbed at venule end); lymphatics pick up excess fluid and return it to blood Exchanges between IF and ICF occur across plasma membranes – Two-way osmotic flow of water, while ions move selectively into or out of cells ▪ E.g., if large salt intake increases ECF osmolality, water shifts out of cells (ICF) into ECF until osmolality in both compartments equal again – Nutrients, wastes, gases have unidirectional flow Copyright © 2025 Pearson Education, Inc. All Rights Reserved Exchange of Gases, Nutrients, Water, and Wastes Between the Three Fluid Compartments of the Body Figure 26.3 Exchange of gases, nutrients, water, and wastes between the three fluid compartments of the body. Copyright © 2025 Pearson Education, Inc. All Rights Reserved 26.2 Both Intake and Output of Water Are Regulated Water intake must equal water output: 2500 ml / day – Water gains: ▪ 90% or more taken in via ingested foods and beverages ▪ Small amount produced via cellular metabolism ( 250 ml / day), called metabolic water or water of oxidation – Water losses: ▪ Insensible water loss across skin and airways (which we are unaware of) ▪ Sensible water loss mostly via urine ( 60%), also sweat and feces Osmolality is maintained around 280 − 300 mOsm – Rise in osmolality stimulates thirst and ADH release – Decrease in osmolality inhibits thirst and ADH release Copyright © 2025 Pearson Education, Inc. All Rights Reserved Major Sources of Water Intake and Output Figure 26.4 Major sources of water intake and output. Copyright © 2025 Pearson Education, Inc. All Rights Reserved Regulation of Water Intake Hypothalamic thirst center controls the thirst mechanism (driving force for intake); center simulated by: – Osmoreceptors ▪ Hypothalamic osmoreceptors activated by 1–2% rise in plasma osmolality – Dry mouth – A decrease in blood volume (or pressure) ▪ Via Angiotensin II or baroreceptor input Drinking water inhibits the thirst center as it enters the digestive tract; inhibitory feedback signals include: – Relief of dry mouth – Activation of stomach and intestinal osmoreceptors and stretch receptors Copyright © 2025 Pearson Education, Inc. All Rights Reserved The Thirst Mechanism for Regulating Water Intake Figure 26.5 The thirst mechanism for regulating water intake. Copyright © 2025 Pearson Education, Inc. All Rights Reserved Regulation of Water Output Unavoidable obligatory water losses explain why we cannot live without water very long; they include: – Insensible water loss across skin and airways – Sensible water loss from ▪ Urine (500 ml/day minimum to excrete wastes) ▪ Sweat and feces Volume and solute concentration of urine depend on fluid intake, diet, and variable water loss via sweat (substantial on a hot day) and feces (substantial with diarrhea) – Kidneys begin to eliminate excess water 30 minutes after ingestion (peaks at about one hour) ▪ Takes time for inhibition of ADH release Copyright © 2025 Pearson Education, Inc. All Rights Reserved Influence of Antidiuretic Hormone (ADH) Water reabsorption in collecting ducts is proportional to ADH release – Fall in ADH increases urine output and decreases volume of body fluids ▪ Urine diluted as less water is reabsorbed (less ADH = fewer aquaporins) – Rise in ADH decreases urine output and increases volume of body fluids ▪ Urine concentrated as more water (via more aquaporins) is reabsorbed ADH release stimulated by: – Relatively small rise in ECF osmolality ▪ Via hypothalamic osmoreceptors (primary regulator of ADH secretion) – Relatively large drop in blood volume or BP ▪ Via baroreceptors and renin-angiotensin-aldosterone system (indirectly) – ADH (also called vasopressin) constricts arterioles by binding to vasopressin receptors to directly raise BP ▪ Can be caused by intense sweating, vomiting, or diarrhea, severe blood loss, traumatic burns, and prolonged fever Copyright © 2025 Pearson Education, Inc. All Rights Reserved Mechanisms and Consequences of ADH Release Figure 26.6 Mechanisms and consequences of ADH release. Copyright © 2025 Pearson Education, Inc. All Rights Reserved Disorders of Water Balance Three main disorders of water balance: dehydration, hypotonic hydration, and edema Dehydration (ECF fluid loss) – Due to hemorrhage, severe burns, prolonged vomiting or diarrhea, profuse sweating, water deprivation, diuretic abuse, endocrine disturbances – Early signs and symptoms: ▪ Thirst, “cotton” mouth, dry flushed skin, reduced urine output (oliguria) – May lead to: ▪ Weight loss, mental confusion, and even hypovolemic shock Copyright © 2025 Pearson Education, Inc. All Rights Reserved Disturbances in Water Balance (1 of 2) Figure 26.7a Disturbances in water balance. Copyright © 2025 Pearson Education, Inc. All Rights Reserved Disorders of Water Balance Hypotonic hydration – Overhydration that occurs with renal insufficiency or rapid excess water ingestion – ECF osmolality decreases, causing hyponatremia ▪ Results in net osmosis of water into tissue cells (cells swell) ▪ Symptoms: severe metabolic disturbances, nausea, vomiting, muscular cramping, cerebral edema (which can lead to death) ▪ Acute hyponatremia treated with hypertonic saline Edema – Accumulation of IF; tissue swells, not cells (no change to ICF compartment) ▪ Only volume of IF is increased (no change to other ECF compartments) – Increases distance for diffusion of oxygen and nutrients from blood into cells ▪ Can impair tissue function – Caused by anything that increases fluid flow out of blood (or decreases return of fluid to it) Copyright © 2025 Pearson Education, Inc. All Rights Reserved Disturbances in Water Balance ) Figure 26.7b Disturbances in water balance. Copyright © 2025 Pearson Education, Inc. All Rights Reserved 26.3 Sodium, Potassium, Calcium, and Phosphate Levels Are Tightly Regulated Electrolyte balance usually refers only to salt balance even though electrolytes also include acids and bases, and some proteins Salts control fluid movements, and provide minerals (ions) for excitability, secretory activity, and permeability of cell membranes – Including: sodium (Na+ ), potassium (K + ), calcium (Ca2+ ), and phosphate (HPO42− ) Salts enter body by ingestion (some liberated during metabolism) – Lost via perspiration, feces, urine, vomit Copyright © 2025 Pearson Education, Inc. All Rights Reserved Causes and Consequences of Electrolyte Imbalances Table 26.1 Causes and Consequences of Electrolyte Imbalances Abnormality ION (Serum Value) Possible Causes Consequences Sodium Hypernatremia Dehydration; uncommon in healthy Thirst. CNS dehydration leads to + individuals; may occur in infants, older confusion and lethargy progressing to ( Nasu per pluse xce ss: greater than (Na excess : adults, or any individual unable to coma; increased neuromuscular 14 145 mEq/L) 5m il ieq ui v alentsper lit e r) indicate thirst; or may result from irritability evidenced by twitching and excessive intravenous NaCl convulsions. administration Hyponatremia Solute loss, water retention, or both Most common signs are those of + (e.g., excessive Na loss through neurologic dysfunction due to brain (Na+ deficit ( Nasu perplusde f icit lest h an Nasup er plus vomiting, diarrhea, burned skin, gastric swelling. If body sodium content is suction, or excessive use of diuretics); normal but water is excessive, the 135 mEq/L) deficiency of aldosterone (Addison’s 13 5m il ieq uivalen t sp er lit e r) symptoms are the same as those disease, Le pp. 625–626); renal f twardarr ow of water excess: mental confusion; disease; excess ADH release; excess giddiness; coma if development H2O ingestion H2O occurs slowly; muscular twitching, irritability, and convulsions if the condition develops rapidly. In hyponatremia accompanied by water loss, the main signs are decreased blood volume and blood pressure (circulatory shock). Copyright © 2025 Pearson Education, Inc. All Rights Reserved Causes and Consequences of Electrolyte Imbalances Table 26.1 [cont.] inue d Abnormality ION (Serum Value) Possible Causes Consequences Potassium Hyperkalemia Renal failure; deficit of Nausea, vomiting, diarrhea; aldosterone; rapid intravenous bradycardia; cardiac arrhythmias (K + excess : ( Ksu per pluse xce ss: greater than 5.5mi li e quivalen t spe r l iter ) infusion of KCl; burns or severe and arrest; skeletal muscle 5.5 mEq/L) tissue injuries that cause K + to Ksup er p lu s weakness; flaccid paralysis. leave cells Hypokalemia Gastrointestinal tract Cardiac arrhythmias, flattened T (K + deficit ( Ksupe r plusde f icit lest h an disturbances (vomiting, wave on ECG; muscular diarrhea), gastric suction; weakness; metabolic alkalosis; Cushing’s syndrome; inadequate 3.5mi li e quiva le ntsperl iter ) 3.5 mEq/L) mental confusion; nausea; dietary intake (starvation); vomiting. hyperaldosteronism; diuretic therapy Copyright © 2025 Pearson Education, Inc. All Rights Reserved Causes and Consequences of Electrolyte Imbalances Table 26.1 [cont.] inue d Abnormality ION (Serum Value) Possible Causes Consequences Phosphate Hyperphosphatemia Decreased urinary loss due to renal Clinical symptoms arise (H 2 − super 2 P O44 failure; hypoparathyroidism; major because of reciprocal changes ( HPO excess: mi n usexc ess : tissue trauma; increased intestinal in Ca2+ levels rather than 2.9 mEq / L) Casup er 2plus greater than2.9mil liequiva le nt spe r li ter) absorption directly from changes in plasma Hypophosphatemia phosphate concentrations. (HPO42− deficit: ( HPO4sup er 2minusd efi cit: Decreased intestinal absorption; increased urinary output; 1.6 mEq / L) lesstha n1.6m il ieq uivalen t sp er lit e r) hyperparathyroidism Chloride Hyperchloremia Dehydration; increased retention or No direct clinical symptoms; intake; metabolic acidosis; symptoms generally associated ( Cl s 105m il ieq (Cl− excess: > uperm inuse ui v xcess:gr e alentsper lit e at e r) rt h an hyperparathyroidism with the underlying cause, 105 mEq / L) which is often related to pH abnormalities. Hypochloremia Metabolic alkalosis (e.g., due to vomiting or excessive ingestion of (Cl− dificit: ( Cl s uperm inusdif icit: alkaline substances); aldosterone deficiency 95 mEq / L) lessthan95mi li e quivalen t sperl it e r) Copyright © 2025 Pearson Education, Inc. All Rights Reserved Causes and Consequences of Electrolyte Imbalances Table 26.1 [cont.] inue d Abnormality ION (Serum Value) Possible Causes Consequences Calcium Hypercalcemia Hyperparathyroidism; excessive Decreased neuromuscular vitamin D; prolonged immobilization; excitability leading to cardiac (Ca2+ excess: > (C 2amsu i lipeeruiv 2pl alu es te xce sls:it e 5. q nsper r or 10. 5mil ligr a mspercent) su per ast e r isk renal disease (decreased excretion); arrhythmias and arrest, skeletal 5.2 mEq / L or malignancy muscle weakness, confusion, stupor, and coma; kidney stones; 10.5mg%)* nausea and vomiting. Hypocalcemia Burns (calcium trapped in damaged Increased neuromuscular tissues); hypoparathyroidism; vitamin excitability leading to tingling (Ca2+ deficit:< 9mi li g ( Casu 4.5mi li e per 2plusde quivalen r amsperc f icit : t sperl it e ent) supe r or r as t erisk D deficiency; renal tubular disease; fingers, tremors, skeletal muscle 4.5 mEq / L or renal failure; hyperphosphatemia; cramps, tetany, convulsions; diarrhea; alkalosis depressed excitability of the 9 mg%)* heart; osteomalacia; fractures. Copyright © 2025 Pearson Education, Inc. All Rights Reserved Causes and Consequences of Electrolyte Imbalances Table 26.1 [cont.] inue d Abnormality ION (Serum Value) Possible Causes Consequences Magnesium Hypermagnesemia Rare; occurs in renal failure when Mg2+ is M g super 2 plus Lethargy; impaired CNS (Mg2+ excess: not excreted normally; excessive functioning, coma, ( Mgsupe r 2plusexces s: greater than2.2mil liequiva le nt spe r li ter) ingestion of Mg2+ -containing antacids M g super 2 plus respiratory depression; cardiac 2.2 mEq / L) arrest. Hypomagnesemia Alcohol use disorder; chronic diarrhea, Tremors, increased severe malnutrition; diuretic therapy neuromuscular excitability, (Mg2+ deficit: ( Mgsuper 2plusdeficit : lessthan1.4mil liequiva le nt spe r li ter) tetany, convulsions. 1.4 mEq / L) *1 mg% = 1 mg / 100 ml Copyright © 2025 Pearson Education, Inc. All Rights Reserved Clinical—Homeostatic Imbalance 26.1 Severe electrolyte deficiencies may prompt craving for salty foods – Common with Addison’s disease, a disorder in which too little aldosterone is produced by adrenal cortex (so too much Na+ lost in urine) Pica: abnormal cravings; eating substances like chalk, clay, starch, or burnt match tips – Caused by deficiency in minerals such as iron Copyright © 2025 Pearson Education, Inc. All Rights Reserved Central Role of Sodium in Fluid and Electrolyte Balance Sodium most abundant cation, sodium salts most abundant solutes, in ECF – NaHCO3 and NaCl contribute 280 of total 300 mOsm ECF solute concentration Only cation exerting significant osmotic pressure – Controls ECF volume and water distribution because water follows salt – Change in blood Na+ levels affect blood volume and pressure, but also ICF and IF volumes Na+ that leaks into cells is pumped out against its electrochemical gradient Na+ moves between ECF and body secretions (e.g., digestive secretions) Renal acid-base control mechanisms are coupled to Na+ transport Copyright © 2025 Pearson Education, Inc. All Rights Reserved Central Role of Sodium in Fluid and Electrolyte Balance Sodium concentration versus sodium content – Concentration of Na + ▪ Stable because of water shifts between compartments ▪ Determines ECF osmolality, and influences excitability of neurons and muscles ▪ Controlled long term by thirst and ADH – Content of Na + + ▪ Total Na content determines ECF volume and therefore blood pressure ▪ Regulated by hormones: – Renin-angiotensin-aldosterone system increases Na+ content + – Atrial natriuretic peptide (ANP) decreases Na content Copyright © 2025 Pearson Education, Inc. All Rights Reserved Table 26.2 Sodium Concentration and Sodium Content Blank ECF Na + Concentration Nasupe r plus Body Na + Content Nasupe r plus Homeostatic ECF osmolality Blood volume and blood pressure Importance Sensors Osmoreceptors Baroreceptors Primary ADH and thirst Renin-angiotensin-aldosterone and ANP Regulators mechanisms hormone mechanisms* *ADH and thirst are also required to maintain blood volume and for long-term control of blood pressure. Copyright © 2025 Pearson Education, Inc. All Rights Reserved Regulation of Sodium Balance (1 of 5) Na+ -water balance coupled to blood pressure and volume control mechanisms (no known receptors that directly monitor Na+ levels in body fluids) Changes in BP or volume trigger neural and hormonal controls to regulate Na+ content Influence of aldosterone and angiotensin II – Aldosterone plays biggest role in regulation of Na+ ▪ Regardless of aldosterone presence – 65% of filtered Na+ is reabsorbed in the PCT and another 25% in the nephron loops; only 10% remains entering DCT and CD Copyright © 2025 Pearson Education, Inc. All Rights Reserved Regulation of Sodium Balance (2 of 5) ▪ When aldosterone concentrations are high most of the remaining filtered Na+ is actively reabsorbed in the DCT and CD – Water follows, so ECF volume increases ▪ When aldosterone concentrations are low most of the remaining filtered Na+ is excreted – Water follows (into urine), so ECF volume decreases Copyright © 2025 Pearson Education, Inc. All Rights Reserved Regulation of Sodium Balance (3 of 5) Influence of aldosterone and angiotensin II (cont.) inue d – Renin-angiotensin-aldosterone system is main trigger for aldosterone release ▪ Granular cells of JGC secrete renin in response to: – Decreased stretch (due to decreased blood pressure) – Sympathetic nervous system stimulation (in response to low BP) – Low filtrate NaCl concentration (via signals from MD cells) ▪ Renin catalyzes first step in production of hormone angiotensin II, which: – Stimulates aldosterone release from adrenal cortex, which increases Na+ reabsorption by kidney tubules + – Aldosterone release also triggered by elevated K levels in ECF – Aldosterone brings about its effects (increased reabsorption of Na+ and secretion of K + ) slowly, over hours Copyright © 2025 Pearson Education, Inc. All Rights Reserved Mechanisms and Consequences of Aldosterone Release Figure 26.8 Mechanisms and consequences of aldosterone release. Copyright © 2025 Pearson Education, Inc. All Rights Reserved Clinical—Homeostatic Imbalance 26.2 Aldosterone deficiency, as in Addison’s disease, can result in huge NaCl and water loses – Patients at risk of hyponatremia and hypovolemia (low blood volume), must be monitored carefully and ingest adequate amounts of salt and fluids Copyright © 2025 Pearson Education, Inc. All Rights Reserved Regulation of Sodium Balance (4 of 5) Influence of atrial natriuretic peptide (ANP) – High BP stretches atrial (heart) cells, causing them to secrete atrial natriuretic peptide – ANP decreases blood pressure and blood volume via: ▪ Inhibits ADH, renin (so angiotensin II), and aldosterone production – Increases excretion of Na+ (natriuresis) and water (diuresis) – Promotes vasodilation directly, and by decreasing production of angiotensin II Copyright © 2025 Pearson Education, Inc. All Rights Reserved Mechanisms and Consequences of ANP Release Figure 26.9 Mechanisms and consequences of ANP release. Copyright © 2025 Pearson Education, Inc. All Rights Reserved Regulation of Sodium Balance (5 of 5) Influence of other hormones – Female sex hormones ▪ Estrogens (like aldosterone chemically) increase Na+ (salt) reabsorption in renal tubules (like aldosterone), which can lead to: – Salt and water retention during menstrual cycles and pregnancy ▪ Progesterone causes mild diuresis (probably blocks aldosterone receptors) – Glucocorticoids ▪ At high levels, glucocorticoids increase salt reabsorption in renal tubules (like aldosterone); water follows, which can lead to edema Cardiovascular baroreceptors – Baroreceptors alert brain to reduce SNS stimulation of the kidneys if blood volume and BP rise (opposite if they fall); decreased sympathetic output causes: + ▪ Afferent arterioles dilate → GFR increases → Na (salt) and water output increase → blood volume and pressure decline back to normal Copyright © 2025 Pearson Education, Inc. All Rights Reserved Mechanisms Regulating Sodium and Water Balance Help Maintain Blood Pressure Homeostasis Figure 26.10 Mechanisms regulating sodium and water balance help maintain blood pressure homeostasis. Copyright © 2025 Pearson Education, Inc. All Rights Reserved Regulation of Potassium Balance (1 of 3) Potassium (K + ) is most abundant ICF cation; required for essential metabolic activities, including functioning of the “excitable” cells (neurons and muscle cells) – ICF-ECF K + concentrations directly affect resting membrane potential (RMP) + ▪ Rise in ECF [K ] (hyperkalemia) makes RMP more positive, which causes depolarization (often followed by reduced excitability) + ▪ Fall in ECF [K ] (hypokalemia) causes hyperpolarization (RMP more negative), which causes reduced excitability (even nonresponsiveness) + – Abnormal [K ] (hyper- or hypokalemia) in heart can interfere with electrical conduction, leading to sudden death K + is also part of body’s buffer system: H+ shifts into and out of cells in exchange for K + to maintain cation balance – So ECF K + levels rise with acidosis and fall with alkalosis + – pH driven shifts in K + can affect ECF [K ] and therefore activity of excitable cells Copyright © 2025 Pearson Education, Inc. All Rights Reserved Regulation of Potassium Balance (2 of 3) Regulatory site: the DCT and collecting duct – K + balance is controlled in DCT and collecting ducts by regulating amount secreted into filtrate (after reabsorbing 90% in the PCT and nephron loop) + ▪ High ECF K + content favors principal cell secretion of K + ▪ Low ECF K + causes principal cells to minimize secretion of K – Also, type A intercalated cells can reabsorb some K + left in filtrate – Kidneys have limited ability to retain K + (regulation revolves around excretion), so K + loss (in urine) that exceeds K + intake leads to hypokalemia Copyright © 2025 Pearson Education, Inc. All Rights Reserved Regulation of Potassium Balance (3 of 3) Influence of plasma potassium concentration – Most important factor affecting K + secretion is its concentration in ECF + – High K + diet leads to increased K + content of ECF, which increases K entry into principal cells, and their subsequent secretion ▪ Low K + diet or accelerated K + loss reduces its secretion and promotes its limited reabsorption Influence of aldosterone – Aldosterone stimulates K + secretion (and Na + reabsorption) by principal cells – Adrenal cortical cells are directly sensitive to K + content of ECF + ▪ Increased ECF [K ] in adrenal cortex causes release of aldosterone, which increases K + secretion ▪ So, K + controls its own ECF concentrations via feedback regulation of aldosterone release Copyright © 2025 Pearson Education, Inc. All Rights Reserved Clinical—Homeostatic Imbalance 26.3 Salt substitutes have become popular in an effort to reduce NaCl levels that affect blood pressure Salt substitutes are high in potassium, and heavy consumption is safe only when aldosterone release in body is working normally – In the absence of aldosterone, hyperkalemia is swift and lethal regardless of K + intake – If an adrenocortical tumor pumps out extreme amounts of aldosterone, ECF K + levels fall so low that neurons hyperpolarize and paralysis occurs Copyright © 2025 Pearson Education, Inc. All Rights Reserved Regulation of Calcium and Phosphate Balance 99 of body’s Ca2+ is found in bones as calcium phosphate salts ECF [Ca2+ ] rarely deviates from normal, regulated by parathyroid hormone (PTH) – PTH promotes increase in ECF Ca2+ levels by targeting ▪ Bones (osteoclasts): increase bone resorption to release Ca2+ and PO43 − ▪ Kidneys (DCT): increase Ca2+ reabsorption, decrease PO43 − reabsorption – PTH reduces active transport of PO43 − at the DCT by decreasing its Tm – Also stimulates activation of vitamin D (hormone called Calcitriol) ▪ Small intestine: increase Ca2+ absorption (indirectly through Calcitriol) 2+ – 98 of filtered Ca passing through DCT normally reabsorbed due to PTH – PTH secretion inhibited when blood Ca2+ calcium levels normal or elevated Copyright © 2025 Pearson Education, Inc. All Rights Reserved Effects of Parathyroid Hormone on Bone, the Kidneys, and the Intestine Figure 16.12 Effects of parathyroid hormone on bone, the kidneys, and the intestine. Copyright © 2025 Pearson Education, Inc. All Rights Reserved Clinical—Homeostatic Imbalance 26.4 Ca2+ in ECF is important for: – Blood clotting – Cell membrane permeability – Secretory activities – Neuromuscular excitability (most importantly) Hypocalcemia increases neuromuscular excitability and can lead to muscle tetany Hypercalcemia inhibits neurons and muscle cells and may cause lethal heart arrhythmias Copyright © 2025 Pearson Education, Inc. All Rights Reserved Regulation of Anions Cl− is major anion accompanying Na+ in ECF, – Both help maintain normal osmotic pressure of blood – 99% of Cl− is reabsorbed (when pH normal or slightly alkaline) ▪ Passively follows Na+ in PCT; coupled to active transport of Na+ in other tubule segments When acidosis occurs, fewer Cl− are reabsorbed in lieu of HCO3− – Cl− reabsorption less critical than acid-base regulation Most other anions (like sulfates and nitrates) have transport maximums, so excesses are excreted in urine Copyright © 2025 Pearson Education, Inc. All Rights Reserved 26.4 Chemical Buffers and Respiratory Regulation Rapidly Minimize pH Changes (1 of 2) All proteins (via their hydrogen bonds) are affected by [H+ ], including enzymes – So nearly all biochemical reactions are affected by the pH of their environment Acid-base balance of body fluids is closely regulated – Normal pH of arterial blood: pH 7.4 ▪ Venous blood and interstitial fluid: pH 7.35, and ICF: pH 7.0 – Lower pH because more acidic metabolites and CO2 ▪ Alkalemia is arterial pH > 7.45 – Resulting condition of the body is called alkalosis ▪ Acidemia is arterial pH < 7.35 – Resulting condition of the body is called acidosis – pH between 7.0 and 7.35 called physiological acidosis (since technically in the neutral to alkaline range on pH scale) Copyright © 2025 Pearson Education, Inc. All Rights Reserved 26.4 Chemical Buffers and Respiratory Regulation Rapidly Minimize pH Changes (2 of 2) Small amounts of acidic substances enter body in food but most H+ generated as by-products or end products of metabolism: – Phosphorus-containing protein breakdown releases phosphoric acid into ECF – H+ and lactate from anaerobic respiration of glucose – Fatty acids and ketone bodies from fat metabolism – H+ is liberated when CO2 is converted to HCO3− in blood Plasma H+ concentration regulated sequentially by three mechanisms 1. Chemical buffers ▪ Immediate, first line of defense 2. Brain stem respiratory centers ▪ Acts within 1–3 minutes; changes ventilation to remove more (or less) CO2 from blood, which in turn lowers (or raises) H+ concentration in blood 3. Renal mechanisms ▪ Most potent, but require hours to days to correct acid-base imbalance Copyright © 2025 Pearson Education, Inc. All Rights Reserved Chemical Buffer Systems (1 of 4) Acids are proton (H+ ) donors – Strong acids dissociate completely in water; quickly release H+ to lower pH – Weak acids dissociate partially and reversibly ▪ Limiting fall in pH by releasing H+ when strong base added Bases are proton acceptors – Strong bases dissociate completely in water; quickly tie up H+ to raise pH – Weak bases dissociate partially and reversibly ▪ Minimizing rise in pH by binding H+ when strong acid added Chemical buffer is a system of one or more compounds (combining weak acid and weak base) that resist pH changes when a strong acid or base is added Three major buffering systems: bicarbonate, phosphate, and protein buffer systems Copyright © 2025 Pearson Education, Inc. All Rights Reserved Dissociation of Strong and Weak Acids In Water Figure 26.11 Dissociation of strong and weak acids in water. Copyright © 2025 Pearson Education, Inc. All Rights Reserved Chemical Buffer Systems (2 of 4) Bicarbonate buffer system (only important ECF chemical buffer) – Mixture of H2 CO3 (weak acid) and salts of HCO3 − (weak base); e.g., NaHCO3 – If strong acid is added, HCO3 − ties up H + and forms H2 CO3 HCl + NaHCO3 → H2CO3 + NaCl strong acid weak base weak acid salt ▪ pH decreases only slightly (as strong acid replaced by weak), unless all available HCO3 − (alkaline reserve) is used up − ▪ Blood [HCO3 ] is closely regulated by kidneys (normally ~ 25 mEq/L) – If strong base added, it causes H2 CO3 to dissociate and donate H + NaOH + H2CO3 → NaHCO3 + H2O strong base weak acid weak base water ▪ pH rises only slightly (as strong base replaced by weak) ▪ H2 CO3 supply (via CO2 + H2O) always available, subject to respiratory controls Copyright © 2025 Pearson Education, Inc. All Rights Reserved Chemical Buffer Systems (3 of 4) Phosphate buffer system – Works like ECF bicarbonate buffer, but more important for buffering urine and ICF (where phosphate concentrations are usually higher) ▪ Weak acid = dihydrogen phosphate (H2PO4 − ), 2− ▪ Weak base = monohydrogen phosphate (HPO4 ) – H + released by strong acids is tied up with a weak acid: HCl + Na2HPO4 → NaH2PO 4 + NaCl strong acid weak base weak acid salt – Strong bases are converted to weak bases: NaOH + NaH2PO4 → Na2HPO4 + H2O strong base weak acid weak base water Copyright © 2025 Pearson Education, Inc. All Rights Reserved Chemical Buffer Systems (4 of 4) Protein buffer system – Intracellular proteins most abundant and powerful buffers (plasma proteins also important) ▪ E.g., hemoglobin functions as intracellular buffer in RBCs – Proteins are amphoteric molecules (act as both weak acid and weak base) ▪ When pH rises, carboxyl groups ( −COOH) release H+ R − COOH → R − COO− + H+ ▪ When pH falls, amino groups ( −NH2 ) bind H+ R − NH2 + H+ → R − NH3+ Copyright © 2025 Pearson Education, Inc. All Rights Reserved Quick Summary of Chemical Buffer Systems Bicarbonate Buffer System Mixture of H2 CO3 (weak acid) and salts of HCO3 − (weak base) Main ECF buffer; also operates in ICF Phosphate Buffer System Salts of H2PO4 − (weak acid) and HPO42− (weak base) Important buffer in urine and ICF Protein Buffer System Some amino acid side chains can act as weak acids ( −COOH) or weak bases (e.g., −NH2 ) Most important buffer in ICF; also in blood plasma Figure 26.12 Quick summary of chemical buffer systems. Copyright © 2025 Pearson Education, Inc. All Rights Reserved + Respiratory Regulation of H Hsupe r plus (1 of 2) Respiratory system eliminates CO2 (therefore carbonic acid) from blood A reversible equilibrium exists in blood – During CO2 unloading (lungs), reaction shifts to left ( H+ is incorporated into H2O) – During CO2 loading (tissues), reaction shifts to right ( H+ is buffered by proteins) A rise in arterial PCO2 activates medullary chemoreceptors (via generating H+ in CSF), + while a rise in arterial [H ] activates peripheral chemoreceptors; both trigger: – Increased ventilation (respiratory rate and depth), causing more CO2 to be removed from blood, shifting reaction to the left, which reduces H+ concentration Copyright © 2025 Pearson Education, Inc. All Rights Reserved + Respiratory Regulation of H Hsupe r plus (2 of 2) A fall in arterial PCO2 or [H+ ] (rise in blood pH) inhibits medullary chemoreceptors – Decreased ventilation allows blood CO2 to accumulate, shifting reaction to the right, raising [H+ ] concentration (lowering pH) back to normal Respiratory system impairment causes acid-base imbalances – Hypoventilation causes respiratory acidosis – Hyperventilation causes respiratory alkalosis Copyright © 2025 Pearson Education, Inc. All Rights Reserved 26.5 Renal Regulation Is a Long-Term Mechanism for Controlling Acid-Base Balance Chemical buffers cannot eliminate excess acids or bases from body – Lungs eliminate carbonic acid, a volatile acid, by eliminating CO2 – Kidneys eliminate nonvolatile (fixed) acids produced by cellular metabolism (phosphoric, uric, and lactic acids and ketones) to prevent metabolic acidosis – Kidneys also regulate blood levels of alkaline substances; renew chemical buffers Kidneys regulate acid-base balance by adjusting blood [HCO3− ] by either: – Conserving (reabsorbing) or generating new HCO3− ▪ Adding one HCO3− is same as losing one H+ – Excreting (via secreting) HCO3− ▪ Removing one HCO3− is same as gaining one H+ Regulation of acid-base balance depends on kidney’s ability to secrete or retain H+ − – Kidneys must secrete H+ to reabsorb HCO3 – Kidneys must reabsorb (retain) H+ to secrete (and excrete) HCO3− Copyright © 2025 Pearson Education, Inc. All Rights Reserved Conserving Filtered Bicarbonate Ions: Bicarbonate Reabsorption (1 of 2) To maintain alkaline reserve, kidneys must replenish HCO3− Apical membranes of tubule cells are impermeable to HCO3− (but permeable to CO2 ) – HCO3− indirectly reabsorbed into tubule cell as CO2 ; once in cell, CO2 can be converted back into HCO3− + HCO3− reabsorption coupled to H+ secretion—for each secreted H that binds to HCO3− in the filtrate a HCO3− is reabsorbed (occurs in PCT and type A intercalated cells of CD) Copyright © 2025 Pearson Education, Inc. All Rights Reserved Conserving Filtered Bicarbonate Ions: Bicarbonate Reabsorption (2 of 2) – Step 1: CO2 + Η2O from H2CO3 in tubule cell (catalyzed by carbonic anhydrase) – Step 2: H2CO3 breaks down into H+ + HCO3− – Step 3: H+ is pumped into filtrate, and HCO3− enters blood in exchange for Cl− – Step 4: H+ combines with HCO3− in filtrate, forming H2CO3 – Step 5: H2CO3 dissociates into CO2 + Η2 O – Step 6: CO2 diffuses into tubule cell Copyright © 2025 Pearson Education, Inc. All Rights Reserved Figure Animation: Renal Regulation of PH Balance Click here to view ADA compliant Animation: Renal Regulation of pH Balance https://mediaplayer.pearsoncmg.com/assets/sci-ap-renal-regulation-of-ph-balance Copyright © 2025 Pearson Education, Inc. All Rights Reserved Reabsorption of Filtered HCO3− Is HC O3sup er minus + Coupled to H Secretion (1 of 6) Hsupe r plus Figure 26.13 Reabsorption of filtered HCO3− is coupled to H+ secretion. Copyright © 2025 Pearson Education, Inc. All Rights Reserved Reabsorption of Filtered HCO3− Is HC O3sup er minus + Coupled to H Secretion (2 of 6) Hsupe r plus Figure 26.13 Reabsorption of filtered HCO3− is coupled to H+ secretion. Copyright © 2025 Pearson Education, Inc. All Rights Reserved − Reabsorption of Filtered Is HCO3 HC O3sup er minus + Coupled to H Secretion (3 of 6) Hsupe r plus Figure 26.13 Reabsorption of filtered HCO3− is coupled to H+ secretion. Copyright © 2025 Pearson Education, Inc. All Rights Reserved Reabsorption of Filtered HCO3− Is HC O3sup er minus + Coupled to H Secretion (4 of 6) Hsupe r plus Figure 26.13 Reabsorption of filtered HCO3− is coupled to H+ secretion. Copyright © 2025 Pearson Education, Inc. All Rights Reserved − Reabsorption of Filtered Is HCO3 HC O3sup er minus + Coupled to H Secretion (5 of 6) Hsupe r plus − + Figure 26.13 Reabsorption of filtered HCO3 is coupled to H secretion. Copyright © 2025 Pearson Education, Inc. All Rights Reserved Reabsorption of Filtered HCO3− Is HC O3sup er minus + Coupled to H Secretion (6 of 6) Hsupe r plus + Figure 26.13 Reabsorption of filtered HCO3− is coupled to H secretion. Copyright © 2025 Pearson Education, Inc. All Rights Reserved Generating New Bicarbonate Ions (1 of 3) Metabolism generates new H+ , which must be balanced by generating new HCO3− − Secreted H+ , used to reabsorb filtered HCO3 is not excreted from body; it is incorporated into Η2O that is reabsorbed – So ECF has same number of HCO3− and H+ as it started out with; no net gains Two mechanisms in the PCT and type A intercalated cells (excretion of buffered H+ , in the form of H2PO4− and NH4+ ) generate “new” HCO3− – New because they are being added to the existing alkaline reserve Copyright © 2025 Pearson Education, Inc. All Rights Reserved Generating New Bicarbonate Ions (2 of 3) Via excretion of buffered H+ – Most important urine buffer is phosphate buffer system ▪ Secreted H+ binds to (buffered by) HPO42− , forming H2PO4 − (excreted in urine) ▪ “New” HCO3− is generated in tubule cell (alongside the secreted H+ ), and moves into IF via cotransport, then diffuses into peritubular capillary blood Copyright © 2025 Pearson Education, Inc. All Rights Reserved New HCO3 − Is Generated via Buffering of Secreted H+ HC O3sup er minus Hsupe r plus by HPO42− (Monohydrogen Phosphate) (1 of 5) HP O42minus − + 2− Figure 26.14 New HCO3 is generated via buffering of secreted H by HPO4 Copyright © 2025 Pearson Education, Inc. All Rights Reserved New HCO3 − Is Generated via Buffering of Secreted H+ HC O3sup er minus Hsupe r plus by HPO42− (Monohydrogen Phosphate) (2 of 5) HP O42minus Figure 26.14 New HCO3 − is generated via buffering of secreted H+ by HPO42− (monohydrogen phosphate). Copyright © 2025 Pearson Education, Inc. All Rights Reserved New HCO3 − Is Generated via Buffering of Secreted H+ HC O3sup er minus Hsupe r plus by HPO42− (Monohydrogen Phosphate) (3 of 5) HP O42minus + 2− Figure 26.14 New HCO3 − is generated via buffering of secreted H by HPO4 (monohydrogen phosphate). Copyright © 2025 Pearson Education, Inc. All Rights Reserved New HCO3 − Is Generated via Buffering of Secreted H+ HC O3sup er minus Hsupe r plus by HPO42− (Monohydrogen Phosphate) (4 of 5) HP O42minus Figure 26.14 New HCO3 − is generated via buffering of secreted H+ by HPO42− (monohydrogen phosphate). Copyright © 2025 Pearson Education, Inc. All Rights Reserved New HCO3 − Is Generated via Buffering of Secreted H+ HC O3sup er minus Hsupe r plus by HPO42− (Monohydrogen Phosphate) (5 of 5) HP O42minus Figure 26.14 New HCO3 − is generated via buffering of secreted H+ by HPO42− (monohydrogen phosphate). Copyright © 2025 Pearson Education, Inc. All Rights Reserved Generating New Bicarbonate Ions (3 of 3) Via NH4 + excretion – More important mechanism for excreting acid – Involves metabolism of glutamine in PCT cells – Each glutamine produces 2 NH4 + (2 H+ + 2 NH3 ) and 2 "new" HCO3 − ▪ HCO3 − transported to blood while NH4 + excreted in urine – Replenishes alkaline reserve of blood Copyright © 2025 Pearson Education, Inc. All Rights Reserved New HCO3 − Is Generated via Glutamine Metabolism HC O3sup er minus and NH4 + Secretion (1 of 3) NH 4s uper p l us Figure 26.15 New HCO3 − is generated via glutamine metabolism and NH4 + secretion. Copyright © 2025 Pearson Education, Inc. All Rights Reserved New HCO3 − Is Generated via Glutamine Metabolism HC O3sup er minus and NH4 + Secretion (2 of 3) NH 4s uper p l us + Figure 26.15 New HCO3 − is generated via glutamine metabolism and NH4 secretion. Copyright © 2025 Pearson Education, Inc. All Rights Reserved New HCO3 − Is Generated via Glutamine Metabolism HC O3sup er minus and NH4 + Secretion (3 of 3) NH 4s uper p l us Figure 26.15 New HCO3 − is generated via glutamine metabolism and NH4 + secretion. Copyright © 2025 Pearson Education, Inc. All Rights Reserved Bicarbonate Ion Secretion If plasma pH becomes alkaline, type B intercalated cells in the CD can: – Secrete HCO3 − while reabsorbing and generating H+ ▪ Opposite of type A intercalated cell Predominant process in nephrons and collecting ducts is HCO3 − reabsorption – Even during alkalosis more HCO3 − is conserved than excreted Copyright © 2025 Pearson Education, Inc. All Rights Reserved 26.6 Abnormalities of Acid-Base Balance Are Classified as Metabolic or Respiratory All cases of acidosis and alkalosis can be classified as either respiratory or metabolic (based on cause) – Respiratory acidosis and alkalosis ▪ Caused by failure of respiratory system to perform pH-balancing role ▪ Kidneys compensate to correct pH – Metabolic acidosis and alkalosis ▪ All abnormalities other than those caused by high or low arterial PCO2 ▪ Lungs compensate to correct pH Copyright © 2025 Pearson Education, Inc. All Rights Reserved Respiratory Acidosis and Alkalosis Most important indicator of respiratory function is PCO2 (normally 35–45 mm Hg) – PCO2 above 45 mm Hg indicates respiratory acidosis ▪ Common cause of acid-base imbalances ▪ Due to insufficient ventilation (slow, shallow breathing) or gas exchange (e.g., emphysema, pneumonia, cystic fibrosis) ▪ pH falls as arterial PCO2 rises – PCO2 below 35 mm Hg indicates respiratory alkalosis ▪ Common result of hyperventilation, often due to stress or pain ▪ pH rises as arterial PCO2 falls (CO2 is being eliminated faster than produced) Copyright © 2025 Pearson Education, Inc. All Rights Reserved Table 26.3-1 Causes and Consequences of Acid-Base Imbalances (1 of 2) Respiratory Acidosis (Hypoventilation) Condition and Hallmark Possible Causes; Comments If uncompensated (uncorrected): Impaired lung function (e.g., chronic PCO2 45 mm Hg; pH 7.35 PsubCO2greater tha n45mil lim e te r smercu r y;pH lest h an7.35 bronchitis, cystic fi brosis, emphysema): impaired gas exchange or alveolar ventilation Impaired ventilatory movement: paralyzed respiratory muscles, chest injury, extreme obesity Narcotic or barbiturate overdose or injury to brain stem: depression of respiratory centers, resulting in hypoventilation and respiratory arrest Copyright © 2025 Pearson Education, Inc. All Rights Reserved Table 26.3-1 Causes and Consequences of Acid-Base Imbalances (2 of 2) Respiratory Alkalosis (Hyperventilation) Condition and Hallmark Possible Causes; Comments If uncompensated: Strong emotions: pain, anxiety, fear, panic PCO2 35 mm Hg; pH > 7.45 attack PsubCO2l e ssthan3 5mi li metersmercury; pHlesstha n7.45 Hypoxemia: asthma, pneumonia, high altitude; represents effort to raise PO2 at the P sub O 2 expense of excessive CO2 excretion CO 2 Brain tumor or injury: abnormal respiratory controls Copyright © 2025 Pearson Education, Inc. All Rights Reserved Metabolic Acidosis and Alkalosis Metabolic acidosis – Low blood pH and HCO3 − – Causes: ▪ Ingestion of too much alcohol (converts to acetic acid) ▪ Excessive loss of HCO3 − (e.g., persistent diarrhea) ▪ Accumulation of lactic acid (exercise or shock), ketosis in diabetic crisis or starvation, and kidney failure Metabolic alkalosis – High blood pH and HCO3 − ▪ Much less common than metabolic acidosis – Causes: ▪ Vomiting acidic contents of stomach ▪ Intake of excess base (e.g., eating too many antacids) Copyright © 2025 Pearson Education, Inc. All Rights Reserved Table 26.3-2 Causes and Consequences of Acid-Base Imbalances (1 of 2) Metabolic Acidosis Condition and Hallmark Possible Causes; Comments If uncompensated: Severe diarrhea: bicarbonate-rich intestinal (and HCO3 − 22 mEq / L; pH 7.35 pancreatic) secretions rushed through digestive tract before HC O3mi n uslesstha n22mil liequ i valentspe r l iter; pHl e ssthan7.3 5 their solutes can be reabsorbed Renal disease: failure of kidneys to rid body of acids formed by normal metabolic processes Untreated diabetes mellitus: lack of insulin or inability of tissue cells to respond to insulin, resulting in inability to use glucose; fats are used as primary energy fuel, and ketoacidosis occurs Starvation: lack of dietary nutrients for cellular fuels; body proteins and fat reserves are used for energy—both yield acidic metabolites as they are broken down for energy Excess alcohol ingestion: results in excess acids in blood Copyright © 2025 Pearson Education, Inc. All Rights Reserved Table 26.3-2 Causes and Consequences of Acid-Base Imbalances (2 of 2) Metabolic Alkalosis Condition and Hallmark Possible Causes; Comments + If uncompensated: Vomiting or gastric suctioning: loss of stomach HCl requires that H Hsup er plus HCO3 − 26 mEq / L; pH 7.45 HC O3su per minu sgr e ater tha n26mi li e quiva le ntsperl iter ;pH g re ater than7.4 5 be withdrawn from blood to replace stomach acid; thus H+ decreases and HCO3− increases Hsup er plus HC O3su per minu s proportionately Selected diuretics: cause K + depletion and H2O loss. Low K + su per p lu s H2O Ksup er p lu s directly stimulates tubule cells to secrete H+. H su pe rp lu s Reduced blood volume elicits the renin-angiotensin-aldosterone system, which stimulates Na+ reabsorption and H+ secretion. Nasup er plus Hsup er plus Ingestion of excessive sodium bicarbonate (antacid): bicarbonate moves easily into ECF, where it enhances natural alkaline reserve Excess aldosterone (e.g., adrenal tumors): promotes excessive reabsorption of Na+ , which pulls increased amount of H+ into urine. Nasup er plus Hsup er plus Hypovolemia promotes the same relative effect because aldosterone secretion is increased to enhance Na+ (and H2O ) reabsorption. Nasup er plus H2O Copyright © 2025 Pearson Education, Inc. All Rights Reserved Effects of Acidosis and Alkalosis Blood pH below 6.8 causes depression of CNS, which can lead to coma and death Blood pH above 7.8 causes overexcitation of nervous system, leading to muscle tetany, extreme nervousness, and convulsions – Death often results from respiratory arrest Copyright © 2025 Pearson Education, Inc. All Rights Reserved Respiratory and Renal Compensations (1 of 3) If acid-base imbalance due to malfunction of: – Respiratory system (failing to maintain normal arterial PCO2 ) ▪ Renal compensations occur – Kidneys attempt to compensate by increasing or decreasing plasma [HCO3 − ] – Urinary system (failing to maintain normal alkaline reserve: [HCO3 − ] in plasma) ▪ Respiratory compensations occur – Lungs attempt to compensate by changing ventilation to increase or decrease arterial PCO2 Copyright © 2025 Pearson Education, Inc. All Rights Reserved Respiratory and Renal Compensations (2 of 3) Respiratory compensation – Lungs try to compensate for metabolic pH problems by adjusting ventilation – Metabolic acidosis: − ▪ Indicated by blood pH below 7.35 and [HCO3 ] below 22 mEq/L ▪ High H + levels stimulate respiratory centers, increasing ventilation, lowering plasma PCO2 levels below normal (to reduce H + and raise pH) – Respiratory compensation indicated by PCO2 below 35 mm Hg – Metabolic alkalosis: ▪ Indicated by blood pH above 7.45 and [HCO3 − ] above 26 mEq/L ▪ Low H + levels inhibit respiratory centers, reducing ventilation, raising plasma PCO2 levels above normal (to generate H + and lower pH) – Respiratory compensation indicated by PCO2 above 45 mm Hg Copyright © 2025 Pearson Education, Inc. All Rights Reserved Respiratory and Renal Compensations (3 of 3) Renal compensation – Kidneys compensate for PCO2 -induced pH problems by adjusting plasma [HCO3 − ] – Respiratory acidosis (via hypoventilation) ▪ Indicated by blood pH below 7.35 and PCO2 above 45 mm Hg ▪ Kidneys secrete more H + while reabsorbing (and producing) more HCO3 − – Renal compensation indicated by [HCO3 − ] above 26 mEq/L – Respiratory alkalosis (via hyperventilation) ▪ Indicated by blood pH above 7.45 and PCO2 below 35 mm Hg ▪ Kidneys excrete more HCO3 − – Respiratory system cannot compensate for respiratory acidosis or alkalosis – Kidneys can only compensate for respiratory acidosis or alkalosis − ▪ Not for imbalances in the alkaline reserve (plasma [HCO3 ]) Copyright © 2025 Pearson Education, Inc. All Rights Reserved Developmental Aspects of Fluid, Electrolyte, and Acid-Base Balance Infants have proportionately more ECF than adults until about 2 years of age Fluid, electrolyte, and acid-base balance problems most common in infancy, reflecting: – The very low residual volume of infant lungs – The high rate of fluid intake and output in infants ( 7 adult values) – The relatively high infant metabolic rate ( 2 adult, yield more metabolic wastes) – The high rate of insensible water loss in infants because of their larger surface area relative to body volume ( 3 adult) – The inefficiency of infant kidneys (at concentrating urine and eliminating acids) ▪ Newborns at risk for dehydration and acidosis (adequate kidney function can take up to a month) In older adults, total body water often decreases (mostly ICF) as muscle mass decreases and fat increases; also, acid-base control mechanisms less responsive – Elderly may be unresponsive to thirst, which puts them at risk for dehydration – Congestive heart failure and diabetes mellitus may cause fluid, electrolyte, or acid- base problems Copyright © 2025 Pearson Education, Inc. All Rights Reserved Copyright This work is protected by United States copyright laws and is provided solely for the use of instructors in teaching their courses and assessing student learning. Dissemination or sale of any part of this work (including on the World Wide Web) will destroy the integrity of the work and is not permitted. The work and materials from it should never be made available to students except by instructors using the accompanying text in their classes. All recipients of this work are expected to abide by these restrictions and to honor the intended pedagogical purposes and the needs of other instructors who rely on these materials. Copyright © 2025 Pearson Education, Inc. All Rights Reserved